Exploring the Complex World of Quantum Computer Chips
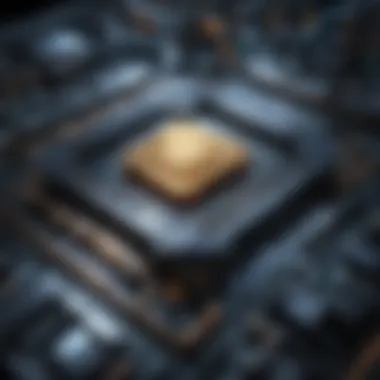
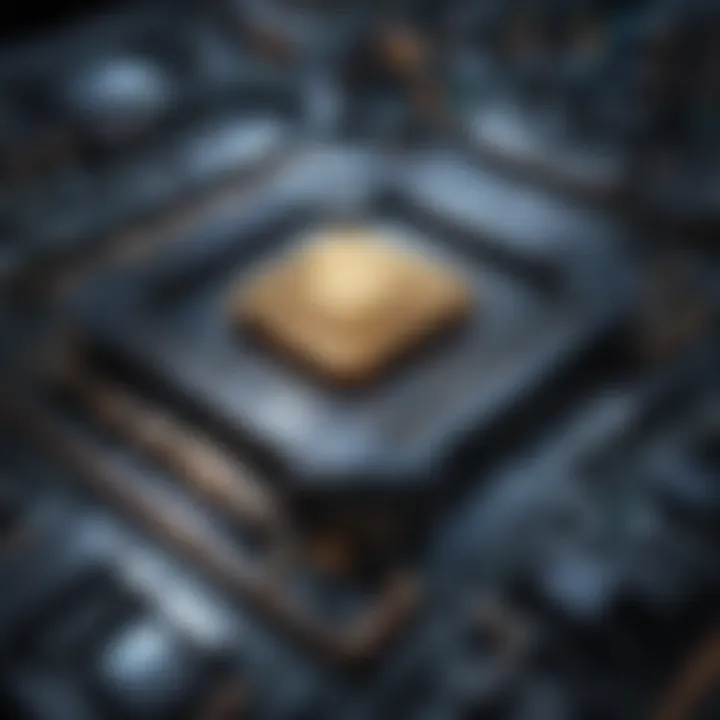
Intro
In the rapidly evolving realm of technology, quantum computing stands as a beacon of transformative potential. The development of quantum computer chips has captivated researchers, engineers, and industry players alike. These chips, unlike their traditional counterparts, leverage the principles of quantum mechanics to perform calculations at speeds that can boggle the mind.
The essence of quantum chips lies in their ability to manipulate qubits rather than bits. Instead of a binary approach, qubits can represent a 0, a 1, or both simultaneously. This property, called superposition, opens avenues for parallelism that classical computers struggle to match. However, the journey from theory to practical, functional chips has not been without its hurdles.
Understanding the architecture, materials, and performance metrics that define quantum computer chips is crucial for anyone interested in the future of computing. This exploration aims to connect the dots between abstract principles and tangible advancements, shedding light on the unfolding narrative of quantum technology.
By outlining the key components and challenges, this piece will not only serve as a primer but also as a bridge for those seeking detailed insights into quantum computing's future trajectories.
Intro to Quantum Computing
Quantum computing represents a profound shift in how we process information. Unlike classical computers, which operate using bits as the smallest unit of data, quantum computers leverage the unique characteristics of quantum mechanics. This shift is not merely a step forward in computational speed; it opens doors to solving complex problems that remain intractable for today's best supercomputers.
This section aims to illuminate the crucial role of quantum computing in advancing technology, finance, pharmaceuticals, and numerous other fields. The potential applications span from cryptography to optimization and beyondâdelving into realms that are currently not feasible under classical paradigms. By grasping the significance of quantum computing, readers can appreciate its transformative impact on our digital world.
Defining Quantum Computing
Quantum computing can be defined as the study of how quantum systems can be manipulated to perform calculations. At its core, it diverges from traditional computing through the employment of qubits, the quantum analog of classical bits. Unlike classical bits that exist in a state of either 0 or 1, qubits can exist in a superposition of states, representing both 0 and 1 simultaneously. This characteristic, in addition to phenomena like entanglement and interference, allows quantum computers to process a vast amount of data concurrently, leading to potentially exponential increases in computing capability.
For instance, consider the task of factoring large numbersâan essential component of many encryption systems. A quantum computer has the potential to solve this problem significantly quicker than any classical counterpart by significantly reducing the computational complexity. Herein lies the intrigue and necessity of quantum computing; redefining how we approach problems that plague various industries.
Historical Context
The journey into quantum computing began in the 1980s, as physicist Richard Feynman proposed that classical computers could not efficiently simulate quantum systems. This insight catalyzed a wealth of theoretical exploration. Subsequently, in 1994, Peter Shor introduced an algorithm capable of factoring big numbers exponentially faster than classical algorithms, painting a vivid picture of its possibilities.
Over the decades, significant milestones have been reached, not just in theory but in practical implementation too. Companies like IBM and Google have made strides in building functional quantum computers, with IBMâs Quantum Hummingbird and Google's Sycamore chip laudable examples. The present day sees more than just academic interest; an entire ecosystem of startups, researchers, and large enterprises is vigorously pushing the frontier of quantum technology.
Understanding these historical developments is critical for comprehending the current landscape and future direction of quantum computing. Each advancement builds upon its predecessors, drawing a roadmap of what has been achieved and providing insight into what might lie ahead.
"Quantum computing holds the promise of solving problems in milliseconds that would take classical computers millions of years."
This narrative sets the stage for deeper exploration into the intricacies of quantum computer chips, from their components to their performance metrics, laying a clear path forward in this astonishingly complex yet rewarding field.
Fundamentals of Quantum Information Theory
In the realm of quantum computing, understanding the fundamentals of quantum information theory is akin to grasping the foundation of a skyscraper; serve as the basis upon which all other complexities rest. This area of study encapsulates the principles that govern how information is stored, processed, and transmitted in quantum systems. It is crucial not just for researchers diving headfirst into quantum mechanics, but also for practitioners who aim to harness these concepts for practical applications. By dissecting the unique attributes of quantum information, one can appreciate the profound differences between classical and quantum computing.
The significance of quantum information theory stems from its ability to elucidate new algorithms and protocols that outshine classical counterparts, opening doors to possibilities previously thought to be unattainable. Let's break this down further by delving into two core components: Qubits vs Classical Bits, and Quantum Entanglement.
Qubits vs Classical Bits
At the core of quantum information lies the concept of qubits. Unlike classical bits, which can exist in one of two statesâ0 or 1âqubits can thrive in a superposition of both states simultaneously. This duality means a single qubit can store more information than its classical counterpart, effectively enabling quantum computers to perform a multitude of calculations in parallel.
Key differences between qubits and classical bits:
- Superposition: A qubit can represent both 0 and 1 at once, doubling the amount of information it can hold.
- Entanglement: Qubits can become entangled, creating correlations that classical bits cannot achieve. This phenomenon allows for incredibly efficient information transfer.
- Measurement: Observing a qubit forces it into one of the two states, fundamentally altering its state and providing a unique challenge in manipulation.
The implications of these differences allow quantum computers to tackle specific problemsâsuch as factoring large numbers or simulating atomic structuresâmuch more efficiently than classical machines. As intriguing as all of this sounds, one must consider that the practical application of qubits is fraught with challenges.
Quantum Entanglement
Quantum entanglement is one of the most captivating phenomena within quantum information theory. Essentially, entanglement occurs when pairs or groups of qubits become interlinked in such a way that the state of one qubit instantaneously influences the state of another, regardless of the distance separating them. This instantaneous connection raises questions about the very nature of information transfer and communication.
The importance of entanglement can be summarized as follows:
- Instantaneous Correlation: Measurements performed on one entangled qubit can affect remote qubits, leading to fast and efficient computation.
- Quantum Communication: Entangled qubits are the backbone of quantum communication technologies, such as quantum key distribution, which ensures secure transmission of information.
- Quantum Computing Power: Entangled qubits enable more complex operations to be conducted with fewer resources, bolstering the potential computational power of quantum systems.
"Quantum entanglement is not just a trick of quantum theoryâit's the key that unlocks the door to enhanced information processing capabilities."
In summary, the fundamentals of quantum information theory pave the way for advancements in quantum computing. With qubits providing an unprecedented ability to manipulate information and entanglement creating deep connections between quantum particles, understanding these concepts is indispensable for anyone involved in this field. The consequences stretch beyond theoretical physics, directly influencing emerging technologies poised to reshape various industries.
Components of Quantum Computer Chips
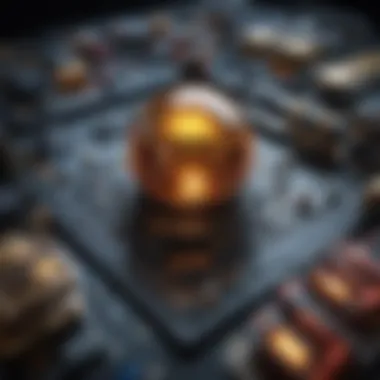
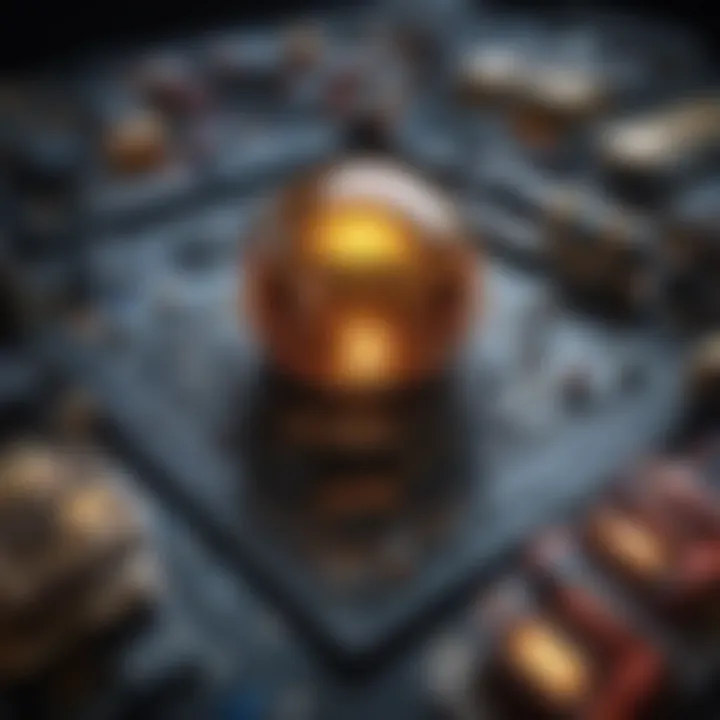
Understanding the components of quantum computer chips is pivotal to grasp the overall framework that supports the burgeoning field of quantum computing. At the heart of these chips lie the elements that facilitate the transfer, manipulation, and storage of quantum information. A profound comprehension of these components not only bolsters our knowledge of how quantum computers operate but also highlights potential areas of innovation and research. By focusing on specific elements such as superconducting materials, quantum dots, and trapped ion technology, we can better appreciate the intricate dance of physics and engineering that underpins quantum computation.
Superconducting Materials
Superconducting materials are awarded a special place in the realm of quantum chips, primarily due to their unique properties that allow for the transmission of electrical current without resistance. This absence of resistance means that superconducting qubits can maintain quantum states longer, which is crucial for operation. By using materials like niobium or aluminum, researchers can craft superconducting circuits that act as the building blocks for qubit creation.
Notably, these materials exhibit a phase transition at specific temperatures, below which they become superconducting. This phenomenon is harnessed in several quantum computing architectures. One of the foremost examples is the transmon qubit, which utilizes superconducting circuits to achieve relatively long coherence times.
- Benefits:
- Low power consumption
- Longer coherence times
- Scalability for larger systems
On a practical level, many groups are running experiments on optimizing these materials to improve performance further. As they push toward the quantum advantage, understanding how to engineer superconductors at the microscopic level will continue to be a field of rich inquiry.
Quantum Dots as Qubits
Quantum dots, often referred to as artificial atoms, are another vital component of quantum computer chips. These nanometer-scale semiconductor structures confine electrons in three-dimensional spaces, effectively creating well-defined energy levels. The behavior of electrons in these dots can be precisely controlled, which makes them particularly useful as qubits.
The beauty of quantum dots lies in their versatility. Depending on the configuration and external fields applied to them, quantum dots can exhibit various quantum phenomena such as entanglement and superposition, essential for quantum computations. Moreover, they can be manipulated using conventional electric fields, making interfacing with classical circuitry more manageable.
- Key Aspects:
- High scalability potential
- Compatibility with existing semiconductor technology
- Flexibility in choosing materials for desired properties
These characteristics open up avenues for compact designs and integration into existing electronic frameworksâa significant stride toward realizing practical quantum computers.
Trapped Ion Technology
Trapped ion technology marks another significant advancement in the components of quantum chips. This approach utilizes ions, which are electrically charged atoms, confined using electromagnetic fields. Once trapped, these ions can be manipulated using laser beams to perform quantum operations. This method boasts remarkable precision because the ions can be highly controlled both in their position and in the quantum states.
The advantages of using trapped ions include:
- Excellent coherence times
- High-fidelity quantum gate operations
- Strong entanglement capabilities across multiple ions
"The trapped ion approach is akin to holding a tiny, glowing star in place, exploiting its light to conduct quantum transactions while remaining immobile among a dynamic environment."
In recent developments, researchers have been pushing the boundaries further by enhancing the scalability of trapped ion approaches. The design involves arrays of ions that can perform quantum computations in parallel, amplifying the speed and efficiency of calculations.
In essence, these three componentsâsuperconducting materials, quantum dots, and trapped ionsâare instrumental to the functioning of quantum computer chips. Understanding their individual benefits and potential applications heralds a new era of computing that has the prospect of solving problems deemed intractable by classical computers.
Chip Architecture and Design
The architecture and design of quantum computer chips play a pivotal role in their efficacy and application. Unlike classical chips, quantum chips must leverage unique principles rooted in quantum mechanics. The layout, connections, and the materials used in constructing these chips determine the level of performance and stability they can achieve. Essentially, a well-designed architecture can make all the difference between a functional quantum processor and one that struggles under the weight of physical limitations.
The major elements in quantum chip architecture include layout design, connectivity between qubits, and the methods of integrating different types of qubits. As quantum technologies advance, recognizing how these elements interplay becomes crucial. Proper articulation of the design not only boosts computational ability but also enhances error resilience.
Quantum Circuits
Quantum circuits serve as the blueprint for quantum computation. Each circuit is designed to execute specific quantum algorithms, operating via gates that manipulate qubits. A fundamental advantage of quantum circuits is their ability to perform numerous calculations simultaneously, a feat unattainable by classical circuits.
Key considerations when designing quantum circuits include:
- Gate Implementation: The choice of gates significantly affects computational efficiency. For example, some premium gates provide higher fidelity and lower error rates.
- Connectivity: The manner in which qubits interactâthrough direct connections or intermediary linksâcan bolster or hinder the computation flow.
- Scalability: As the demand for computational power rises, it is vital to assess how circuits can scale alongside increasing qubit counts.
"The architecture must be resilient enough to accommodate potential scaling issues while maintaining integrity throughout computations."
The design of a quantum circuit must embrace both flexibility and extensibility. By focusing on integration methods that allow for seamless interactions between qubits, developers can potentially elevate the performance of future quantum chips.
Error Correction Mechanisms
With quantum computing's astonishing potential comes the challenge of error rates due to decoherence and other noise factors. Hence, incorporating robust error correction mechanisms is not just beneficial; itâs indispensable. Error correction works by encoding quantum information across multiple physical qubits, effectively safeguarding it from disruptions.
Several strategies for error correction include:
- Shor's Code: This elaborate scheme combines qubits in such a way that even if several are flawed, the consistency of the overall system remains intact.
- Surface Codes: By employing two-dimensional lattices of qubits, surface codes can detect and correct errors with remarkable efficiency.
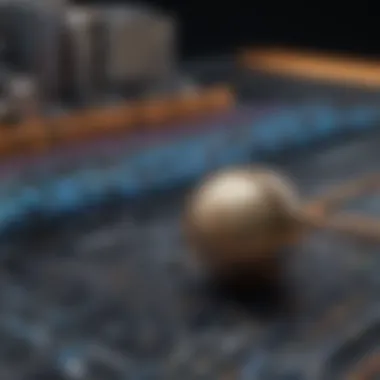
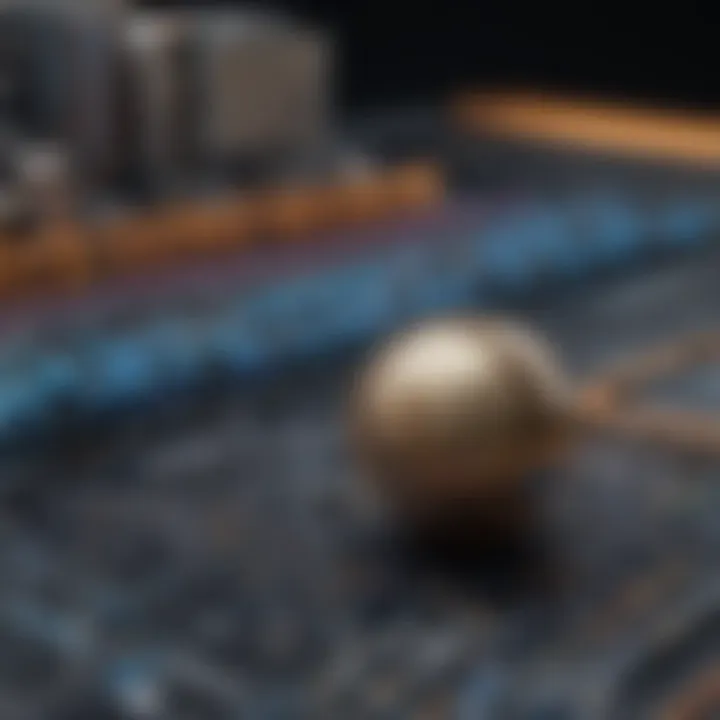
Addressing errors upfront during chip design leads to fewer performance bottlenecks during operations. This proactive stance mitigates risks associated with quantum entanglement and parallel processing tasks. As technologies evolve, so will the sophistication of these correction mechanisms, ultimately enhancing the reliability of quantum computational processes.
In summary, the chip architecture and design are cornerstone aspects of quantum computer functionality. By understanding quantum circuits and implementing effective error correction, researchers and developers can pave the path toward a more robust and efficient quantum computing landscape.
Measuring Quantum Chip Performance
Evaluating quantum chip performance is paramount to understanding how effectively these chips can execute quantum computing tasks. Unlike classical computers, quantum chips require specialized parameters to assess their capabilities. These measurements provide insights not just into the hardware capabilities but also into how well quantum algorithms will perform once they are deployed.
The performance of quantum chips hinges on certain key elements:
- Quantum Gate Fidelity evaluates how accurately quantum gates perform operations. High fidelity indicates low error rates, which is essential for reliable quantum computation.
- Decoherence Times measure how long qubits can maintain their quantum state. Longer decoherence times translate to more complex calculations before information is lost, making this a critical metric in performance assessments.
These factors contribute directly to the overall utility of a quantum computing system, which can have practical implications across various industriesâfrom cryptography to pharmaceuticals. When researchers analyze these performance metrics, they obtain a clearer picture of the quantum chip landscape. Such evaluations help guide future investments, research directions, and potential applications of quantum technologies.
"Understanding performance metrics is fundamental for bridging the gap between theoretical quantum computing and real-world applications."
Quantum Gate Fidelity
Quantum gate fidelity refers to the accuracy with which a quantum gate performs its intended operation on qubits. In essence, it measures how well a quantum gate can preserve the quantum state while performing calculations. High fidelity is crucial because even minor deviations can compound over multiple operations, leading to significant errors in the final output.
Each gate's fidelity can be affected by various factors:
- Physical imperfections in materials used for building qubits.
- Operational errors during implementation of quantum gates.
- Environmental noise, which can introduce disturbances.
The importance of gate fidelity cannot be overstated; it determines the robustness of quantum algorithms. Researchers often strive to achieve gates with fidelity levels greater than 99%, a necessary threshold for scaling quantum computers reliably.
Decoherence Times
Decoherence times are a measure of how long a qubit can maintain its quantum state before it loses coherence with the environment. This decay process occurs due to interactions with external disturbances such as thermal fluctuations, electromagnetic radiation, or even cosmic rays. As a result, decoherence limits the time available for executing quantum operations.
Typically, there are several types of decoherence times depending on the context in which theyâre measured:
- T1 (Energy relaxation time) indicates the time it takes for a qubit to lose energy to its surroundings.
- T2 (Phase decoherence time) measures the loss of coherence and is critical for operations that require maintaining superposition.
Maintaining longer decoherence times allows for executing more intricate calculations, thus expanding the potential applications for quantum computing. Researchers continuously explore new materials and technologies to enhance decoherence times, which can significantly impact the future of quantum computing.
In summary, measuring quantum chip performance through quantum gate fidelity and decoherence times is vital for edging towards successful operational quantum computers. These metrics will define the technologyâs trajectory and open doors to numerous applications that seem just on the horizon.
Challenges in Quantum Chip Development
The development of quantum computer chips holds enormous potential, but it's hardly a walk in the park. As the technology matures, the hurdles become clearer. Understanding the challenges in quantum chip development is pivotal, not just for researchers and engineers but also for anyone interested in the broader scope of quantum computing. The relevance of this discussion expands to implications for industries and academia alike, where practical applications of quantum technology are increasingly sought after.
Scalability Issues
Scalability is a paramount concern when it comes to quantum chips. In essence, moving from a handful of qubits to a larger number presents numerous complications. A basic quantum processor is not simply a larger classical computer; it requires an architecture and mechanisms that support quantum behavior. A few highlights include:
- Error Rates Increase: As we scale up to more qubits, the error rates tend to spike, especially in systems like superconducting qubits or trapped ions. What initially seems viable can quickly become unmanageable when you scale the quantum gates.
- Interconnectedness: The challenge isn't just about the number of qubits, but also how they communicate with each other. Effective coupling is crucial for quantum operations, and ensuring that qubits remain entangled as they scale is a daunting task.
- Cost Efficiency: Meeting the high demand for qubits means increasing production. However, manufacturing costs skyrocket, making it prohibitive for widespread adoption unless a feasible solution is found.
The various methods of constructing qubits, such as using superconducting circuits or adding more quantum dots, must undergo investigation to assess their feasibility in larger systems. "Scaling up is like trying to fit an elephant into a Volkswagen," someone might say; the objective is clear but the means are intricate and laden with complications.
Thermal Control Problems
Another layer of complexity comes from thermal control issues. Quantum chips often function at cryogenic temperatures which adds an extra layer of engineering constraints. Here are some key points:
- Thermal Isolation: Any heat generated by the chip can disrupt fragile quantum states. Thus, designing a chip that maintains low temperatures while minimizing heat influx becomes essential. This often requires a back-and-forth between engineering and materials science â a balancing act if you will.
- Cryogenic Systems: The systems that cool these chips must be ultra-reliable. Failures in these cooling systems can cause the entire chip to enter a decoherence state, which defeats the purpose. An unreliable cooling system doesn't just slow down research; it hampers the entire field.
- Material Limitations: Not all materials hold up when cooled to such low temperatures. The selection of materials can pose weighty repercussions for device performance and integration.
In many ways, tackling these thermal challenges is akin to walking a tightrope; one misstep can lead to catastrophic failure.
Effective management of these challenges will ultimately determine how viable quantum chips become in practical applications. The growth of this technology hinges on overcoming scalability and thermal management issues that can otherwise stunt its progress.
As we venture deep into the world of quantum chip technology, itâs crucial to stay attuned to these nuanced concerns. Addressing scalability and thermal control is not just about optimizing chip performance; it links directly to the future viability and functionality of quantum computers in real-world scenarios.
Recent Advances in Quantum Chip Technology
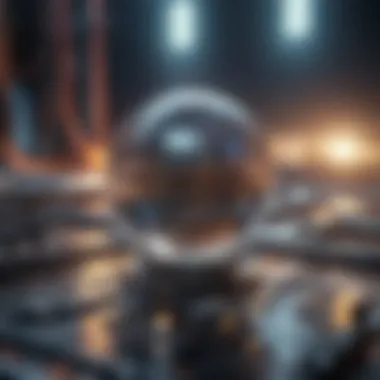
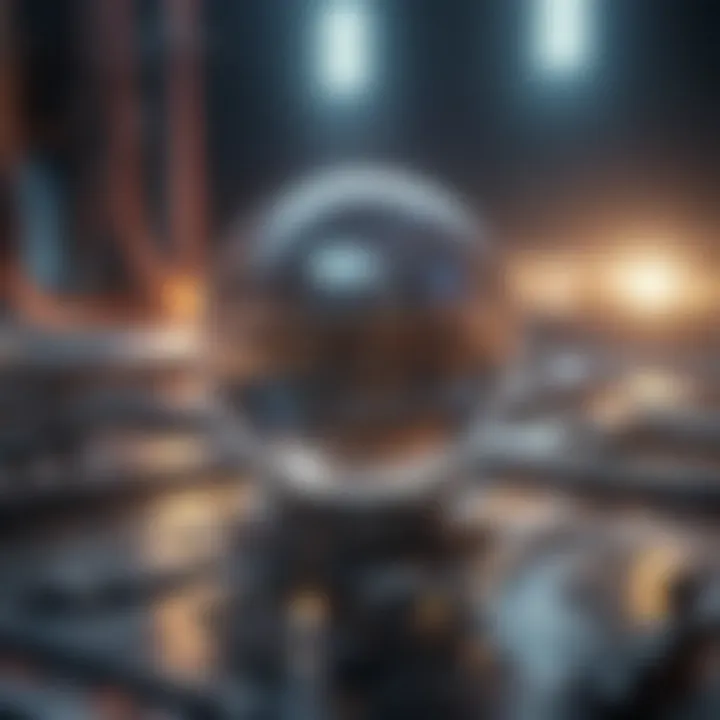
The landscape of quantum chip technology is rapidly evolving. Recent advancements are pivotal, not just for academia but also for industries aiming for breakthroughs in speed and efficiency. Each leap forward in this field clears the fog and reveals new pathways, encouraging collaboration between researchers, engineers, and businesses. The implications for fields like cryptography and complex problem-solving are enormousâand understanding where we stand is key for anyone involved in technology today.
Breakthrough Research Initiatives
Much of the excitement around quantum computing stems from groundbreaking research initiatives worldwide. Universities and institutions are racing to find solutions to the inherent issues within quantum technologies. One notable initiative is the Quantum ASICs project, focused on developing bespoke application-specific integrated circuits designed for quantum computing. This could significantly increase the performance of quantum chips by tailoring the hardware directly to the needs of quantum algorithms.
Another compelling area of research is the development of topological qubits, which promise to be more stable than traditional qubits. By minimizing decoherenceâan effect where quantum information is lostâit is anticipated that we can achieve error rates significantly lower than those currently prevalent. These endeavors illustrate how calculated strategies in the research community can yield tangible benefits toward practical quantum computers.
Innovative Companies and Startups
A number of innovative companies and startups are making waves. For instance, Rigetti Computing is not just throwing its hat in the ring; itâs crafting a whole new dimension for quantum chips. Their cloud platform allows developers to access quantum processors, fostering a unique ecosystem that combines quantum and classical computing. This integration could help democratize access to quantum computing resources, fueling further innovation.
Another standout is IonQ, which has taken the trapped ion approach to the next level. Their systems boast impressive performance metrics and have even attracted attention from investors in the tech space. The companyâs emphasis on practical applicationsâlike enhancing machine learning algorithmsâcreates an interesting bridge between current technology and future possibilities.
Through these initiatives, we see the customary lines between academia and industry blurring, fostering an environment ripe for rich collaboration and rapid advancement.
In summary, the quest for effective quantum chips is fostering a vibrant landscape of research and innovation. These efforts, spearheaded by research initiatives and inventive startups, position the field of quantum computing on the cusp of monumental breakthroughs. The synergy of these components could lead us to entirely new computational paradigms, setting the stage for what might very well be a revolution in technology.
Applications of Quantum Computer Chips
The significance of quantum computer chips cannot be overstated as they pave the way for revolutionary shifts across various industries. These chips, harnessing the peculiar principles of quantum mechanics, can accomplish complex calculations far beyond the reach of classical counterparts. Their applications touch on numerous fieldsâfrom cybersecurity to aeronautical engineering. To understand their potential, letâs break down some of the most compelling uses:
Cryptography
In the realm of cryptography, quantum computer chips offer groundbreaking solutions for securing data. Unlike traditional encryption methods, quantum encryption exploits the laws of physics. For instance, Quantum Key Distribution (QKD) enables two parties to share a secret key securely. If any eavesdropper attempts to intercept the key, the quantum state of the particles used would change, alerting the parties involved. This unique trait offers a level of security that classical encryption simply canât match. As a consequence, industries handling sensitive informationâsuch as finance and healthcareâmay soon turn to quantum-safe algorithms to perpetually safeguard their data.
Optimization Problems
Optimization problems lurk in almost every corner of business and technology, from logistics to oil drilling. Here, quantum chips shine brightly. Their ability to process vast sets of possibilities simultaneously allows for finding optimal solutions faster than older technologies. Consider the traveling salesman problem: a quantum computer can evaluate multiple routes at once, arriving at the best one quicker than any classic computer ever could. Companies dealing with supply chain management, where even a slight increase in efficiency can translate to considerable cost savings, stand to benefit enormously from quantum optimizations.
Simulating Quantum Systems
Simulating quantum systems is another area where quantum computer chips have a significant edge. Many materials and reactions at the quantum level are inherently challenging to model using classical computing methods because they involve vast variables and uncertainties. Quantum chips can mimic natural processes, making them invaluable in chemistry and physics. For example, drug discovery can be revolutionized by accurately simulating molecular interactions at a quantum level, speeding up the discovery and development of new medicines. As we delve into these simulations, we begin to unlock doors to innovations that were once just a dream.
"Quantum computers, by their very nature, can address problems that were impossible or impractical to solve with classical techniques."
The potential applications of quantum computer chips are as vast as they are varied. As researchers and companies continue to explore these dimensions, the ripples of change will undoubtedly reshape entire industries, making our world more efficient, secure, and interconnected.
The Future of Quantum Computing
Understanding the future of quantum computing is akin to looking through a fogged window. The view may be obscured, but the underlying potential is clear and exhilarating. This topic is pivotal as it encompasses the transformative ongoing developments in technology, predicting trends that can radically alter various industries. The quantum computing field stands on the brink of breakthroughs that can offer major benefits, but with these come critical considerations that merit thoughtful dialogue.
Predicted Trends
Several trends are expected to shape the landscape of quantum computing in the coming years. The following points illustrate some of the most notable ones:
- Increased Qubit Capacity: As technology accelerates, the ability to fabricate and manage larger qubit systems is projected to improve drastically. Organizations like Google and IBM are already pushing the envelope, indicating the likelihood of systems with thousands of qubits soon.
- Hybrid Quantum-Classical Systems: The future will likely see a blend of classical and quantum computing methods. This allows for near-term applications while fully harnessing quantum properties. Utilizing both in harmony presents a more pragmatic approach to problem-solving.
- Enhanced Error Correction: With scalability becoming more feasible, the focus also shifts to refining error correction methods. Robust algorithms are vital as they play a crucial role in maintaining coherence and improving fidelity.
"The true power of quantum computing lies not only in what we can compute, but also in how we can correct the missteps along the way."
- Quantum Internet Development: Moving beyond isolated quantum computers, the quest for a quantum internet is gaining traction. Entanglement-based communication could redefine security protocols and elevate data transmission capabilities.
- Industry-Specific Applications: From pharmaceuticals to financial modeling, experts predict the emergence of tailored quantum solutions. These applications wonât be limited to a single sector, as diverse industries recognize the unparalleled advantage quantum technology offers in enhancing efficiencies and solving complex problems.
Ethical Considerations
With great power comes great responsibility. As quantum computing technology advances, ethical considerations cannot be sidelined. Here are key points to mull over:
- Data Privacy Risks: The capabilities of quantum computers to potentially break classical encryption methods pose serious threats to personal privacy and commercial confidentiality. There are ongoing debates about how to safeguard information in the quantum era.
- Equitable Access to Technology: As quantum technology emerges, itâs essential to ensure that access is equitable across borders and demographics. Disparities could widen between those with access to advanced technology and those without, posing socio-economic risks.
- Dual-Use Concerns: Quantum computing can serve both beneficial and malicious purposes. This duality raises questions on regulation and oversightâhow to prevent the technology from falling into the wrong hands and being used for nefarious activities.
- Impact on Employment: The rise of automation through quantum computing could reshape job markets. Thereâs the possibility of significant job displacement, prompting discussions on retraining programs and the future of work.
Epilogue
The exploration of quantum computer chips represents not merely a technical endeavor but a significant leap into the future of computing. Over the course of this article, numerous elements have been examinedâranging from fundamental components and architectures to real-world applications and future implications. The importance of this topic cannot be overstated, particularly when considering how quantum computing stands poised to revolutionize various fields.
One core benefit is the unparalleled processing power that quantum chips can offer. When compared to classical chips, their ability to perform calculations at an astonishing speed holds the promise of solving complex problems that are currently beyond our reach. From cryptography to optimization, the applications of these chips are vast, encouraging not just academic interest but investment from both established industries and budding startups.
Moreover, the challenges highlightedâscalability, thermal control, and moreâare equally important. Understanding the pitfalls and hurdles in quantum chip development informs researchers and practitioners alike, guiding future innovations. Addressing these considerations is paramount for harnessing the full potential of quantum technology.
In addition, ethical considerations linked to quantum computing cannot be brushed aside. As these chips become integrated into everyday applications, the conversations surrounding security, data integrity, and the societal impacts of quantum advancements become more pressing. What systems and frameworks need to be established to ensure ethical use? How do we navigate the challenges this technology might pose to existing infrastructures?
"The future of quantum computing will not only depend on technological advancements but also on our response to the ethical dilemmas they present."
In summary, the reflections and insights compiled in this article provide a well-rounded understanding of quantum computer chips. They bring clarity to a complicated subject, illuminating what lies ahead. For students, researchers, educators, and professionals alike, grasping both the science and the implications behind quantum technology can lead to informed discussions and innovative thinking in this rapidly evolving field. The horizon is bright, and as we delve deeper into quantum realms, continuous engagement with these topics will be essential.