Understanding Carbon Sequestration and Its Impact
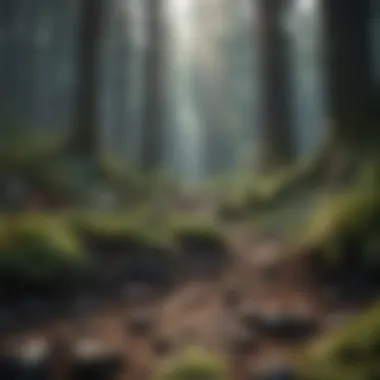
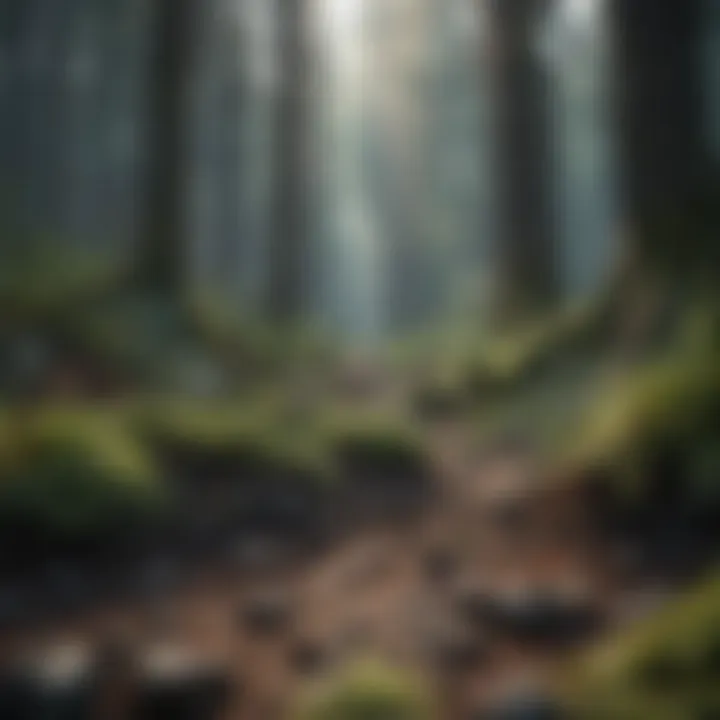
Intro
Carbon sequestration is recognized as a critical response to the urgent challenge of climate change. It involves capturing and storing carbon dioxide from the atmosphere. The necessity for carbon sequestration arises from the rampantly increasing concentrations of carbon dioxide due to human activities, particularly fossil fuel consumption and deforestation. This article provides a comprehensive look into the various mechanisms of carbon sequestration, the implications for environmental policy, and an examination of both natural and technological solutions. Understanding these methodologies is crucial for students, researchers, educators, and professionals engaged in climate science and sustainability initiatives.
Methodologies
Description of Research Techniques
Research into carbon sequestration employs a myriad of techniques designed to evaluate its efficacy and efficiency. Field studies play a pivotal role, as scientists monitor natural ecosystems and their ability to capture carbon. These studies utilize long-term data collection to understand the carbon dynamics in soils, forests, and oceans. Remote sensing also offers insight into large-scale carbon capture processes. This technology helps identify and quantify carbon stocks and fluxes over vast areas, informing policy and ecological assessments.
In addition to observational strategies, laboratory experiments contribute valuable data on carbon absorption mechanisms. Controlled conditions allow scientists to test various hypotheses regarding soil properties, plant species, and microbial activity that enhance sequestration.
Tools and Technologies Used
The field of carbon sequestration is rich with advanced tools and technologies. Key innovations include the use of direct air capture systems. These systems chemically bind CO2 from the atmosphere and either store it or utilize it for other purposes. Notable companies in this space include Climeworks and Carbon Engineering, which aim to scale up these technologies for broader impact.
Geological storage technologies are also significant, which involve injecting CO2 into underground formations. Tools like geological modeling software are essential for predicting storage capabilities and ensuring safety during the process.
Discussion
Comparison with Previous Research
The evolution of carbon sequestration research has shown considerable shifts in methodologies and approaches. Historical methods focused primarily on natural sequestration processes. Recent studies, however, emphasize a mixed approach, combining natural and technological methods to achieve greater carbon reductions. Compared to past models, contemporary research recognizes that solely relying on nature may not suffice to meet global climate targets.
Theoretical Implications
The implications of research into carbon sequestration extend beyond environmental impact. They pose fundamental questions about the reliance on technology versus natural processes. Understanding the carbon cycle dynamics informs theoretical frameworks of climate policy. Researchers argue that integrating both methodologies could lead to a more robust strategy. Policymakers must deliberate on the balance between fostering natural sequestration methods, such as afforestation and preserving ecosystems, and investing in technological advancements.
"Carbon sequestration represents a potential cornerstone in the global strategy to combat climate change, but understanding its mechanisms is key to its successful implementation."
For additional insights on this subject, refer to Wikipedia or explore further materials on Britannica.
Foreword to Carbon Sequestration
Carbon sequestration has gained significant attention in the context of climate change. It represents a critical process through which carbon dioxide is captured and stored to mitigate its impact on global warming. Understanding this process is essential as we explore not only the underlying mechanisms but also the broader implications for the environment and society.
Definition and Importance
Carbon sequestration refers to the process of capturing atmospheric carbon dioxide, often with the intent of storing it in a way that prevents its release back into the atmosphere. This can occur through natural or technological means. For example, forests serve as natural carbon sinks by absorbing carbon dioxide during photosynthesis. Technological approaches, such as Carbon Capture and Storage (CCS), actively trap and store carbon emissions from industrial sources.
The importance of carbon sequestration lies in its potential to reduce greenhouse gas concentrations in the atmosphere. By limiting the amount of carbon dioxide, we can slow the rate of climate change. This process is crucial for maintaining the balance of our ecosystems, enhancing soil health, and contributing to sustainable development. Given the growing urgency of climate action, an understanding of carbon sequestration methods provides a foundation for developing effective environmental policies.
Brief Historical Context
The concept of carbon sequestration is not new. It has evolved over time, driven by the rising awareness of climate change and the need for effective solutions. The scientific understanding of the carbon cycle laid groundwork for recognizing how human activities, particularly fossil fuel combustion, have disrupted natural processes. In the late 20th century, organizations and governments began to prioritize research into methods for capturing carbon emissions.
In 1991, the first underground geological storage test in Norway marked a significant milestone, demonstrating the feasibility of storing carbon dioxide underground. Since then, both natural and technological approaches have advanced significantly. The Kyoto Protocol in 1997 further propelled the global agenda, emphasizing the need for emissions reductions and the role of sequestration as a key strategy.
Today, the field continues to evolve. Ongoing research into innovative techniques and policy frameworks is shaping the future of carbon management strategies. Understanding the historical context not only provides insights into past challenges and successes but also highlights the urgency for continued innovation and support in this field.
Mechanisms of Carbon Sequestration
Carbon sequestration represents a crucial strategy in addressing climate change. Understanding the mechanisms behind it is essential for developing effective policies and practices. There are two primary categories of carbon sequestration: natural and technological. Both play significant roles in capturing and storing carbon dioxide, thereby reducing atmospheric concentrations. This section explores these mechanisms in detail, focusing on their characteristics, benefits, and areas of consideration.
Natural Carbon Sequestration
Natural carbon sequestration involves processes that occur in the environment, specifically within ecosystems. This can be further divided into three main processes: photosynthesis in plants, oceanic absorption, and soil organic matter.
Photosynthesis in Plants
Photosynthesis is a fundamental biological process where plants convert carbon dioxide from the atmosphere into organic compounds. This process plays a vital role in carbon sequestration as it captures carbon dioxide and stores it in biomass, such as wood and leaves. The key characteristic of photosynthesis is that it not only helps in the growth of plant material but also reduces greenhouse gas concentrations.
Beneficial Aspects:
- Absorption of significant amounts of CO2 daily.
- Creation of oxygen as a byproduct, promoting a balanced atmosphere.
- Contribution to biodiversity through habitat creation.
However, the disadvantages include the dependence on environmental factors, such as light and water availability, which can limit its efficacy in areas experiencing climate extremes.
Oceanic Absorption
The ocean acts as a massive carbon sink, absorbing approximately 30% of the carbon dioxide emitted into the atmosphere. The process of oceanic absorption occurs primarily through physical and biological mechanisms. Water absorbs CO2, which is then utilized by marine organisms for photosynthesis. This natural process is characterized by its vast scale and capacity to sequester carbon over long periods.

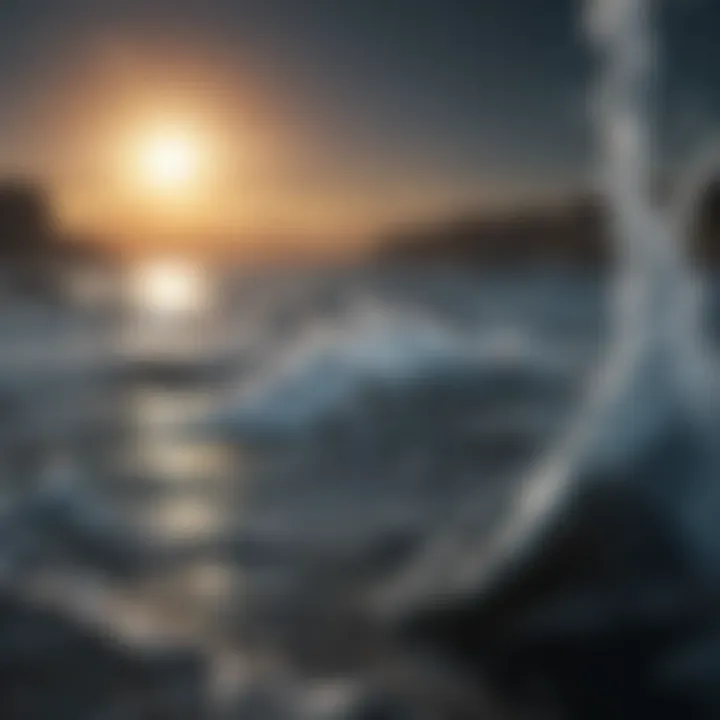
Beneficial Aspects:
- Significant reduction of atmospheric CO2.
- Support for marine ecosystems through nutrient cycling.
Still, the disadvantages are notable. Increasing acidification poses a threat to marine life and may diminish the ocean's capacity to sequester carbon in the future.
Soil Organic Matter
Soil organic matter is another important aspect of natural carbon sequestration. It refers to the organic constituents in soil, resulting from the decomposition of plant and animal material. This process signifies a long-term storage mechanism for carbon. The key characteristic of soil organic matter is its ability to store carbon for decades or even centuries.
Beneficial Aspects:
- Improves soil health and fertility.
- Enhances water retention and resilience to drought.
On the downside, disturbances such as deforestation or agricultural practices can release stored carbon back into the atmosphere, undermining the benefits.
Technological Carbon Sequestration
Technological carbon sequestration includes various human-made methods designed to capture and store carbon dioxide. This category encompasses direct air capture technology, carbon capture and storage (CCS), and enhanced weathering.
Direct Air Capture Technology
Direct air capture technology uses machinery to extract CO2 directly from the ambient air, reducing atmospheric concentrations effectively. The key characteristic of this technology is its ability to be set up anywhere, not requiring specific geographical conditions. This flexibility makes it a popular choice for mitigating climate change.
Beneficial Aspects:
- Immediate impact on reducing atmospheric CO2 levels.
- Scalability allows for potential widespread application.
However, it involves high operational costs and energy use, which could affect the overall effectiveness.
Carbon Capture and Storage (CCS)
Carbon capture and storage (CCS) involves capturing CO2 emissions from sources like power plants before they reach the atmosphere. Once captured, CO2 is transported and stored underground in geological formations. The technological aspect of CCS is its integration within existing industries, allowing for immediate mitigation in high-emission sectors.
Beneficial Aspects:
- Reduces emissions from industrial sources effectively.
- Utilizes existing infrastructure for implementation.
Nevertheless, concerns remain surrounding the long-term safety of underground storage and the potential for leaks.
Enhanced Weathering
Enhanced weathering is a process that accelerates the natural weathering of rocks to capture atmospheric CO2. The key characteristic here is the use of minerals that react with CO2, forming stable carbonates. By enhancing this natural process, the captured carbon can be stored securely in the form of minerals.
Beneficial Aspects:
- Provides a long-term solution for carbon storage.
- Enhances soil quality and reduces ocean acidity.
The challenges associated with enhanced weathering include the need for large-scale mining and transportation of minerals, which raises questions about sustainability and environmental impact.
Understanding these mechanisms highlights the importance of both natural and technological approaches to effectively combat climate change. Each method has its own strengths, weaknesses, and implications, which are critical to consider for future climate strategies.
Biological Mechanisms in Detail
Understanding the biological mechanisms of carbon sequestration is essential for recognizing how natural systems contribute to the reduction of atmospheric carbon dioxide levels. Forests, oceans, and soils work together to capture and store carbon, creating a buffer against climate change. These biological processes are not only vital for carbon storage but also play a crucial role in maintaining biodiversity and supporting ecosystem functionality.
Role of Forests in Carbon Sequestration
Carbon Stocks in Trees
Trees serve as significant carbon sinks, holding large amounts of carbon in their biomass and surrounding soil. The ability of different tree species to accumulate carbon varies, with some species being particularly efficient in this role. Their growth cycles lead to biomass increase, which directly translates to stored carbon. This makes forests a beneficial choice for carbon sequestration strategies.
Notably, tropical forests exhibit higher carbon density due to their diverse and rapid growth rates. The potential for carbon storage in trees is determined not only by the species but also by the age and health of the forest overall. Healthy forests can sequester carbon effectively while simultaneously promoting habitat for wildlife. However, forestry practices and land-use changes can threaten these benefits, highlighting the significance of sustainable management in forest ecosystems.
Reforestation and Afforestation Benefits
Reforestation and afforestation present distinct but important approaches to enhancing carbon sequestration. Reforestation involves replanting trees in deforested areas, restoring ecosystems that have been lost. On the other hand, afforestation refers to planting trees in areas that were not previously forested. These practices contribute significantly to the overall goal of sequestering carbon.
The primary advantage of these techniques is their ability to increase carbon stocks quickly. Newly planted forests can absorb significant amounts of CO₂ as they grow. Besides carbon storage, they also provide numerous ecological benefits such as soil erosion prevention, improving local water cycles, and enhancing biodiversity. However, these practices should be conducted thoughtfully. Poor species selection or inappropriate plantings can lead to ecological imbalance.
Marine Ecosystems
Role of Phytoplankton
Phytoplankton, the microscopic plants in the ocean, play a fundamental role in carbon sequestration. Through photosynthesis, they absorb CO₂, converting it into organic matter. This makes phytoplankton crucial for global carbon cycles. Not only do they help in drawing down atmospheric carbon, but they also produce oxygen, supporting marine life.
One key characteristic of phytoplankton is their rapid growth rate, allowing them to sequester carbon efficiently. They form the base of the marine food web, meaning their health impacts larger organisms and ecosystems. Disruptions to phytoplankton communities, whether due to pollution or climate change, can reduce their effectiveness in capturing carbon. Maintaining ocean health is thus vital for the continued efficacy of these organisms in carbon sequestration.
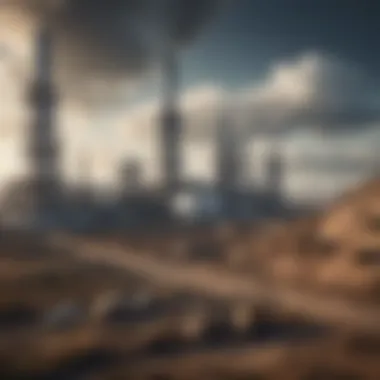
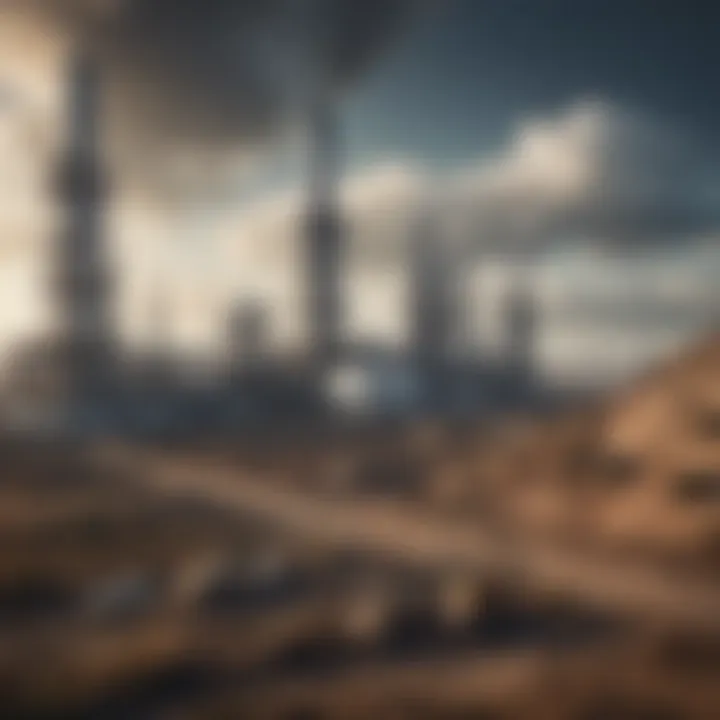
Carbon Sequestration in Mangroves and Seagrasses
Mangroves and seagrasses are crucial coastal ecosystems with a remarkable capacity for carbon sequestration. Mangroves store carbon in their vegetation and the soil beneath them, often more effectively than terrestrial forests. Their unique root structures help stabilize sediments, promoting the accumulation of organic matter, which benefits overall carbon storage.
Seagrasses, found underwater, also contribute significantly by trapping sediment and providing habitats for marine species. They sequester carbon in addition to their primary productivity. The challenge lies in the vulnerability of these systems to human activity and climate change. Coastal development, pollution, and rising sea levels can all lead to a loss of these ecosystems, and with them, their vital carbon storage capacities.
Protecting and restoring mangroves and seagrasses is essential for enhancing carbon sequestration while also providing critical coastal protection and biodiversity benefits.
In summary, biological mechanisms are indispensable in the realm of carbon sequestration. Forests, phytoplankton, mangroves, and seagrasses each contribute in unique and significant ways, supporting not only carbon reduction but also the broader health of our planet.
Technological Approaches Explained
Technological approaches to carbon sequestration are becoming increasingly relevant as climate change intensifies. These methods aim to capture carbon dioxide emissions from sources such as power plants, industrial processes, and even directly from the atmosphere. The significance of these technologies lies in their potential to mitigate greenhouse gas emissions while supporting energy production and industrial activities. Although traditional methods like afforestation play an important role, technological innovations can offer scalable and effective solutions. In turn, these can be integrated with existing infrastructures for a more comprehensive response to climate challenges.
Direct Air Capture: Methodology
Direct Air Capture (DAC) refers to technology that extracts carbon dioxide directly from the ambient air. The process generally involves using chemical reactions to capture carbon dioxide from the atmosphere. The methodologies typically consist of two main phases: capture and storage. In the first phase, large fans pull air into a system where it passes through specialized filters containing sorbents. These materials either chemically bond with or absorb the carbon dioxide.
Once captured, the next step involves storing the carbon. This can be achieved through various means such as geological formations or utilization in various products. The benefit of DAC is its ability to address emissions from dispersed sources. However, it requires a significant amount of energy and resources to operate efficiently.
Carbon Capture and Storage (CCS) Process
Carbon Capture and Storage (CCS) encompasses a suite of technologies aimed at capturing carbon dioxide emissions from large point sources such as coal-fired power plants. The CCS process involves three critical steps: capture, transport, and storage. Initially, carbon dioxide is separated from other gases during combustion. This can be accomplished through absorption, adsorption, or cryogenic processes.
Once captured, the carbon dioxide is compressed and transported, usually through pipelines, to storage sites. These storage locations are typically geological formations located deep underground, often in depleted oil and gas fields or saline aquifers. The efficacy of CCS lies in its demonstrated capacity to prevent significant amounts of carbon dioxide from entering the atmosphere. Nevertheless, concerns about storage integrity and long-term monitoring remain.
Innovative Sequestration Solutions
Bioenergy with Carbon Capture and Storage (BECCS)
Bioenergy with Carbon Capture and Storage (BECCS) is a hybrid approach combining biomass energy generation with carbon capture technologies. In this system, biomass, which certanly absorbs carbon dioxide during its growth, is used as an energy source. The carbon emissions resulting from the combustion of biomass are captured and stored using CCS techniques. This method presents a net-negative emission pathway when managed properly.
The key characteristic of BECCS is its dual benefit of producing energy while potentially removing carbon from the atmosphere. This makes it a promising option for addressing climate change. However, the unique feature of BECCS includes the reliance on sustainable biomass sources. If not managed responsibly, it may lead to land use conflicts or deforestation.
Chemical Looping
Chemical Looping is another innovative approach in carbon sequestration. This technology utilizes metal oxides to transfer oxygen for combustion, allowing for the separation of carbon dioxide with minimal energy loss. The process is designed in such a way that the combustion occurs in a separate reactor, simplifying the carbon capture process.
A key characteristic of Chemical Looping is that it requires lower energy input compared to conventional capture methods. It utilizes the inherent properties of oxygen carriers that are reusable. However, the disadvantages include technical challenges related to material durability and the need for extensive research to optimize processes at a commercial scale.
In summary, integrating technological approaches like DAC, CCS, BECCS, and Chemical Looping is essential for advancing carbon sequestration solutions.
Evaluating the Efficacy of Carbon Sequestration
Evaluating the efficacy of carbon sequestration is critical for understanding its role in climate change mitigation. This analysis encompasses several aspects, including measurements, verification processes, and the overall impact of these efforts on ecosystems and economies. To determine how effective carbon sequestration methods are, it is important to systematically assess their performance and integration into broader climate strategies.
The effectiveness of carbon sequestration is influenced by several specific elements. One is the accuracy of measurements regarding how much carbon is actually captured and stored. Proper methodologies allow for the monitoring of carbon levels and the assessment of whether specific techniques are yielding anticipated results. Additionally, verification processes are essential for validating these findings.
Considerations about evaluating efficacy extend beyond mere numbers. It involves understanding the broader benefits to ecosystems and communities. A comprehensive approach helps identify practices that serve both climate initiatives and local environments.
Measurement and Verification
Measurement and verification provide the foundation to assess the performance of carbon sequestration methods. To ensure that carbon sequestration strategies are truly effective, precise data collection and monitoring must occur. Various technologies and methodologies are employed to measure carbon dioxide levels both before and after sequestration processes. These include:
- Remote sensing technologies: Utilizing satellite imagery and sensors to track carbon uptake.
- Ground-based monitoring: Installing equipment that measures carbon levels in the soil and atmosphere directly.
- Modeling approaches: Creating simulations to predict the behavior of carbon in ecosystems under various conditions.
Verification is crucial to add credibility to these measurements. With third-party evaluations, stakeholders can trust that reports on carbon capture are accurate. Such verification processes can clarify questions related to permanence; namely, how long the carbon remains sequestered and under what conditions it might be released back into the atmosphere.
Life Cycle Assessment
A Life Cycle Assessment (LCA) is an analytical method that examines the environmental impacts associated with all stages of a product's life, from cradle to grave. In the context of carbon sequestration, this assessment provides valuable insights regarding:
- Input resources required for various sequestration methods, such as energy and materials.
- Potential emissions from the technologies employed, allowing for a more comprehensive understanding of net impacts on climate.
- Long-term sustainability of these methods in various ecological contexts.
The LCA approach encourages a holistic view of carbon sequestration practices. It emphasizes that while certain techniques may capture significant amounts of CO2, they must also be evaluated regarding overall feasibility and their environmental footprint. This dual focus ensures that strategies enhance carbon storage without creating adverse effects elsewhere in the ecosystem.
"Evaluating the efficacy of carbon sequestration provides necessary insights that refine our understanding, enabling informed decisions on climate strategies."
By integrating rigorous measurement protocols with comprehensive life cycle assessments, researchers can significantly contribute to the development of effective carbon capture and storage practices. This ensures that the fight against climate change is backed by sound science.
Environmental and Economic Impacts
Understanding the environmental and economic impacts of carbon sequestration is critical for grasping its role in combating climate change. The methods of carbon capture not only have implications for reducing greenhouse gases but also offer significant benefits to the ecosystems and society. This section aims to unravel these complexities, examining both the positive outcomes and the financial considerations associated with different sequestration techniques.
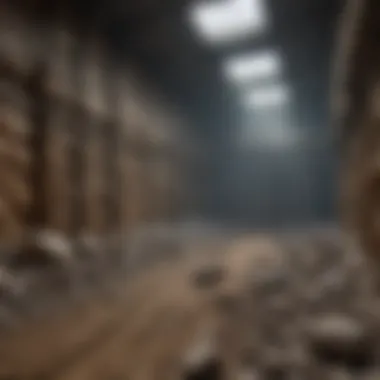
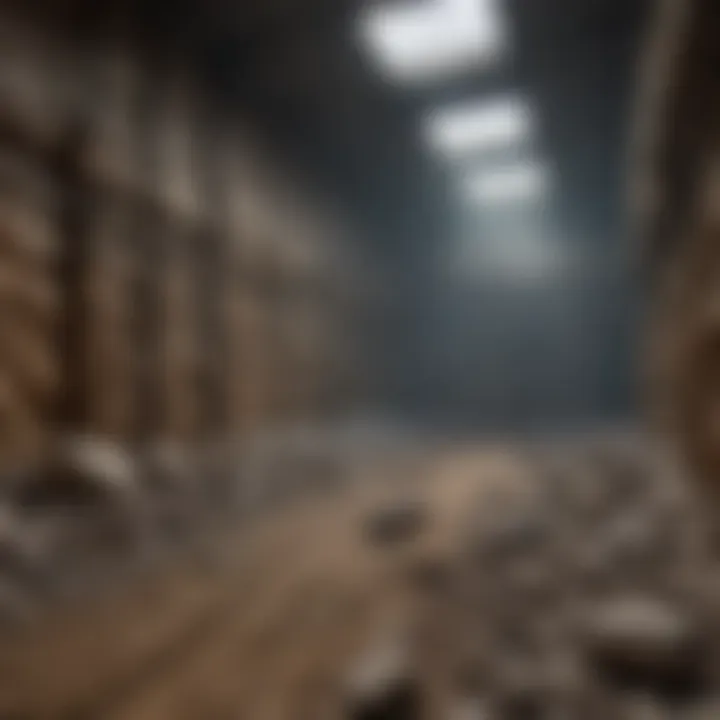
Ecosystem Benefits
Carbon sequestration provides several ecosystem benefits that go beyond just reducing atmospheric CO2.
- Biodiversity Conservation: Healthy ecosystems are essential for maintaining biodiversity. Implementing sequestration strategies, particularly reforestation and afforestation, enhances habitats for numerous species. Forests, wetlands, and oceans serve as vital reservoirs for various forms of life.
- Soil Health Improvement: Natural carbon sequestration processes improve soil health by increasing organic matter. Healthier soil contributes to better water retention and nutrient cycling, which can support agricultural productivity.
- Climate Resilience: Ecosystems that actively sequester carbon are often more resilient to climate-induced changes. For example, forests and mangroves can reduce the impact of flooding and soil erosion, providing significant protection to surrounding communities.
"Effective carbon sequestration strategies can transform landscapes, offering ecological benefits that are essential for sustaining life in the face of climate change."
Cost Analysis of Sequestration Techniques
Evaluating the costs associated with different carbon sequestration techniques is essential for effective policy-making and investment decisions. The costs can vary significantly, shaped by technology, geography, and implementation scale.
- Natural Approaches:
- Technological Solutions:
- Afforestation and Reforestation: These methods tend to have relatively low initial costs, primarily involving land preparation and planting. However, long-term maintenance and monitoring add to costs, making sustainability a concern.
- Soil Carbon Sequestration: Practices like no-till agriculture and cover cropping can improve soil health and yield, often making them cost-effective long-term strategies.
- Direct Air Capture: This emerging technology significantly varies in cost, with estimates ranging from hundreds to thousands of dollars per ton of CO2 captured. The scalability of this method is still under evaluation, affecting its potential as a widespread solution.
- Carbon Capture and Storage (CCS): The initial investment is high, but the long-term benefits might offset these costs, particularly for industries that can utilize captured CO2.
In summary, the economic considerations of carbon sequestration reflect a balance between initial costs, long-term benefits, and environmental sustainability. Understanding these aspects is vital to fostering strategies that not only aim at reducing carbon emissions but also enhance ecological and economic well-being.
Limitations and Challenges
The topic of limitations and challenges is critical when discussing carbon sequestration. Understanding these factors not only provides a realistic view of what is achievable but also emphasizes the need for innovation and careful consideration in implementing various techniques. In an era where climate change poses significant risks, recognizing these boundaries can guide future research and policy formulation.
Scalability Issues
One of the main limitations of carbon sequestration is scalability. Many promising methods, especially in the technological realm, have not yet proven their effectiveness on a broad scale. For instance, while Direct Air Capture shows potential, it faces hurdles in scaling up to the volumes needed to significantly impact atmospheric CO2 levels. High costs and energy demands can deter widespread adoption.
- Infrastructure Needs: Expanding carbon capture networks requires substantial infrastructure investments. Without this, options become limited, particularly in developing areas.
- Resource Allocation: Adequately funding large-scale projects competes with other pressing societal needs. This prioritization can hinder advancements in sequestration technologies.
- Long-term Storage Solutions: Developing reliable storage sites is complex. Many sites around the world may not be suitable or safe for long-term carbon storage, adding another layer of challenge to scalability.
Despite these issues, ongoing research into methods like Bioenergy with Carbon Capture and Storage (BECCS) showcases the potential to address some of these scalability barriers. Ultimately, addressing scalability requires a multifaceted approach involving both technological innovation and strategic planning.
Environmental Concerns
Another significant challenge is the environmental impact of carbon sequestration methods. Some techniques may inadvertently lead to ecological disturbances, compromising the very ecosystems they aim to protect. For example, utilizing land for biofuel production can lead to habitat destruction and loss of biodiversity. This trade-off raises ethical questions about the balance of benefits and harm.
- Land Use Changes: Depending on the sequestration method, land that could support natural ecosystems may be converted for other purposes, impacting wildlife and plant life.
- Water Usage: Technologies like BECCS can consume large quantities of water, compounding strains on local water resources, which are often already vulnerable.
- Potential for Leakage: In geological carbon storage, concerns about leakage remain. If captured carbon finds its way back to the atmosphere, it could negate the benefits gained from initial sequestration efforts.
Understanding these environmental concerns is crucial for developing effective, sustainable carbon sequestration strategies. Policymakes must consider ecological impacts and work toward minimizing adverse effects while maximizing carbon mitigation potentials.
"Recognizing both advantages and limitations leads to a balanced approach in addressing carbon emissions and climate change."
This understanding of limitations and challenges is essential for shaping the future of carbon sequestration strategies. By identifying these hurdles, scientists, policymakers, and stakeholders can better allocate resources and efforts towards effective solutions.
Future Directions and Innovations
The landscape of carbon sequestration is continually evolving, giving rise to innovative techniques and regulatory approaches that seek to optimize carbon capture and storage. This section underscores the importance of future directions and innovations by illustrating how advancements can enhance the efficiency of existing methods and develop new pathways for reduction of greenhouse gases in the atmosphere. As climate change remains a pressing global issue, focusing on emerging technologies and supportive policy frameworks will be pivotal in achieving meaningful progress in carbon sequestration efforts.
Emerging Technologies
Emerging technologies in carbon sequestration are crucial for addressing increasing atmospheric carbon levels. By investing in cutting-edge solutions, researchers and industries aim to create more efficient systems that can capture and store carbon at a larger scale. Notable innovations include:
- Direct Air Capture (DAC): This technology uses large, industrial-scale machines to extract carbon dioxide directly from the air. By employing chemical solvents, DAC systems can pull CO2 into a concentrated form that can then be sequestered underground or used in various applications.
- Biochar: Produced by pyrolysis of biomass, biochar not only sequesters carbon but also enhances soil fertility. Its long-term stability in the soil makes it an effective method for storing carbon over extended periods.
- Carbon Mineralization: This process involves converting CO2 into stable mineral forms, such as carbonates, which can then be stored safely. This method mimics natural processes but aims to accelerate the rate at which carbon is mineralized, potentially sequestering large volumes of CO2.
The combination of these innovations illustrates the potential for technology to play a transformative role in reducing atmospheric CO2 levels.
Policy and Regulatory Framework
The effectiveness of carbon sequestration efforts heavily relies on supportive policy and regulatory frameworks. Governments and organizations must develop comprehensive guidelines to encourage investment and innovation in this field. Key considerations in policy-making include:
- Carbon Pricing: Implementing mechanisms such as carbon taxes or cap-and-trade systems can promote the adoption of carbon capture technologies by making emissions more costly.
- Incentives for Research and Development: Financial support for R&D can spur technological advancements, enabling breakthroughs in carbon capture and storage methods. Grants and subsidies can make significant contributions in this area.
- Public Awareness and Education: Ensuring the public is informed about the necessity and benefits of carbon sequestration is critical. Effective communication strategies can facilitate a greater understanding and acceptance of these initiatives.
- International Cooperation: Climate change is a global challenge requiring collaborative efforts. International regulations may be needed to create a unified framework that encourages nations to share technologies and strategies.
The End
The conclusion of this article encapsulates the critical aspects of carbon sequestration as a pivotal strategy in combatting climate change. It serves not simply as a summary but as a reflection on what has been covered regarding both natural and technological mechanisms employed to capture and store atmospheric carbon. This synthesis enhances the reader's understanding of how these practices can mitigate environmental issues while simultaneously shedding light on future directions.
Summary of Key Points
In this article, we have traversed various facets of carbon sequestration, delving into its mechanisms and implications. Primary points include:
- Natural Carbon Sequestration: We explored how ecosystems such as forests and oceans play a vital role in drawing down carbon dioxide via processes like photosynthesis and oceanic absorption.
- Technological Innovations: Direct air capture and carbon capture and storage methods are discussed, illuminating their potential in significantly reducing greenhouse gas concentrations in the atmosphere.
- Environmental and Economic Impacts: We analyzed how effective carbon storage positively contributes to ecosystems and assessed the associated costs of different sequestration techniques, weighing their benefits against financial feasibility.
- Limitations and Challenges: Issues concerning scalability and environmental impacts were highlighted, emphasizing that while carbon sequestration holds vast promise, it is not devoid of complexities and challenges.
- Future Directions: Finally, the prospects of emerging technologies, alongside the necessary policy frameworks, form the core of how we will advance carbon sequestration efforts going forward.
In summary, understanding the nuances of carbon sequestration is essential for informed policy-making and environmental strategies. This knowledge enables stakeholders—be they students, researchers, educators, or policy makers—to advocate for approaches that are scientifically sound, feasible, and ultimately beneficial for our planet's future.
"Carbon sequestration is not just a tool, but a necessary pivot for achieving sustainability in an era of climate crisis."
The knowledge provided in this article is crucial in informing discussions and actions around climate policy. It is a step further in the collective endeavor toward a sustainable environmental future.