Exploring Short Hairpin RNA Knockdown Mechanisms
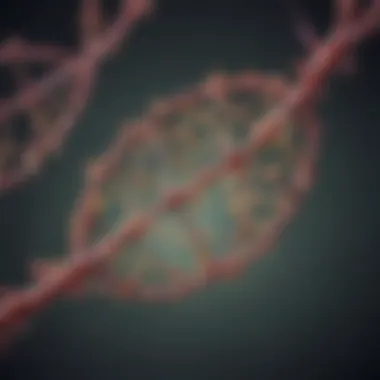
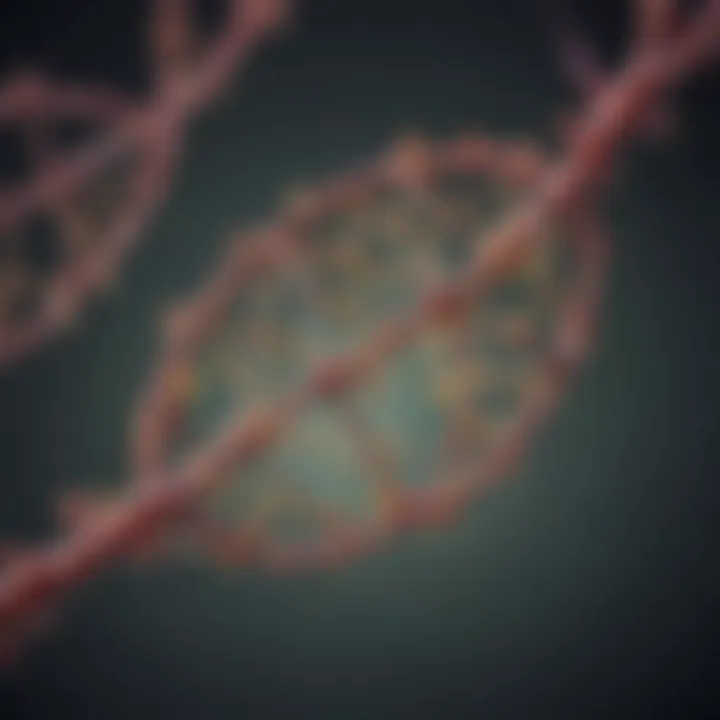
Intro
Short hairpin RNA (shRNA) techniques have gained significant traction in the field of molecular biology, offering potent strategies for gene silencing. This introduction aims to contextualize shRNA within the broader landscape of genetic engineering and therapeutic applications. As researchers and practitioners seek ways to manipulate gene expression, the relevance of shRNA becomes increasingly evident, providing tools to explore gene functionality and therapeutic avenues.
The growing interest in gene knockdown strategies like shRNA reflects the need for precision in manipulating biological pathways. This technology not only facilitates fundamental research but also presents opportunities for developing interventions in various diseases. Traditional methods of gene silencing, such as antisense oligonucleotides or dominant negative constructs, have inherent limitations. shRNA, by contrast, leverages the cellular machinery in a more efficient way to achieve lasting reductions in target gene expression.
In this article, we will -- step by step -- delve into the methodologies employed in shRNA research, the technological advancements that support its applications, and the implications of these methods in both research and clinical environments. By articulating the mechanisms and applications of shRNA, we intend to provide a thorough understanding for students, researchers, educators, and professionals interested in the ever-evolving field of molecular biology.
Methodologies
Understanding the methodologies surrounding shRNA is crucial for grasping its applications in gene regulation.
Description of Research Techniques
Researchers typically utilize various techniques to design, synthesize, and deliver shRNA. The initial phase often involves bioinformatics tools to identify target genes and design specific shRNA sequences. This bioinformatics work is essential because the efficiency of shRNA knockdown is heavily reliant on the sequence’s complementarity to its target mRNA. Once designed, the shRNA can be synthesized using chemical methods or through in vitro transcription using RNA polymerases.
The next stage involves the delivery of shRNA into cells. Common delivery methods include:
- Viral vectors: Lentiviral or adenoviral vectors are frequently used due to their ability to integrate into the host genome, providing stable and sustained knockdown effects.
- Non-viral delivery systems: Techniques like lipid nanoparticles and electroporation are also viable, especially for transient expression in primary or hard-to-transfect cells.
Tools and Technologies Used
The landscape of tools and technologies used in shRNA applications is constantly evolving. Significant advancements include optimization in vector design, more sophisticated delivery methods, and improved techniques for analysis. Key tools used in shRNA research include:
- RNA scaffolds: These stable structures allow for the efficient expression of shRNA in cells.
- High-throughput screening platforms: They enable systematic analysis of gene function and pathway involvement in various cellular systems.
Integrating these technologies creates robust methodologies that are essential for successful shRNA knockdown.
Discussion
In this section, we will assess the implications of shRNA technologies in terms of their theoretical underpinnings and comparative framework.
Comparison with Previous Research
Historically, RNA interference (RNAi) has evolved from early observations of natural mechanisms to the more refined applications we see today. Previous research largely revolved around small interfering RNA (siRNA) technology. However, shRNA presents distinct advantages, notably in its ability to achieve prolonged silencing and a more favorable delivery profile. Comparing the efficiency, duration, and specificity of shRNA with siRNA has led to an understanding of how best to leverage these techniques.
Theoretical Implications
The theoretical implications of shRNA technology extend beyond just gene silencing. It challenges and refines our fundamentals of gene regulation and expression. The ability to disrupt specific pathways can help elucidate complex interactions within cellular systems and highlight targets for therapeutic intervention.
"The advent of shRNA technology represents a significant leap in our capacity to dissect gene function and tackle disease."
By synthesizing these discussions, we can appreciate how shRNA knockdown continues to pave the way for advancements in research and therapeutic strategies, underscoring its relevance in modern biotechnology and medicine.
Preface to Short Hairpin RNA
Short hairpin RNA (shRNA) has emerged as a significant tool in the field of molecular biology and genetic engineering. This section aims to underline the importance of understanding shRNA, particularly regarding its role in gene regulation and silencing. Such knowledge is critical in research settings and clinical applications, where precision in targeting specific genes can lead to advancements in therapies for various diseases.
The exploration of shRNA mechanisms opens up new horizons for studying gene function and interaction. By utilizing shRNA in experiments, researchers can dissect gene pathways more clearly. This understanding assists in identifying potential targets for drug development, providing insights that can enhance therapeutic strategies. Consequently, shRNA's relevance transcends basic research, influencing practical applications in biotechnology.
Definition and Structure
Short hairpin RNA is characterized by its unique structure, consisting of a 20-25 base pair stem and a short loop. This specific conformation allows shRNA to effectively interact with the RNA interference (RNAi) machinery within the cell. When shRNA is introduced into cells, it is processed into small interfering RNA (siRNA) by the enzyme Dicer. The siRNA then engages with the RNA-induced silencing complex (RISC), ultimately leading to the degradation of complementary messenger RNA (mRNA). This mechanism represents a powerful method for downregulating gene expression.
The design of shRNA takes into account several structural elements. The stability of the stem, the length of the loop, and sequence complementarity are all crucial for optimally targeting the desired mRNA. Ensuring high efficacy requires careful consideration during shRNA design, making understanding its structure a fundamental aspect of successful implementation.
Historical Context
The discovery of RNA interference as a means of post-transcriptional gene silencing dates back to the late 1990s. Originally identified in plants, this phenomenon rapidly gained traction as scientists recognized its potential applicability across various organisms, including mammals. The concept of shRNA specifically emerged from this foundational understanding of RNAi.
As research progressed, the ability to manipulate gene expression using shRNA became a focus for laboratories worldwide. This prompted development of various vectors and delivery mechanisms, significantly advancing genetic research methodologies. The recognition of shRNA as a precise tool for gene knockdown has since evolved, leading to a myriad of applications in both research and therapeutic contexts.
The historical developments in shRNA technology demonstrate how essential it has become in molecular biology, paving the way for future innovations. Understanding this timeline not only informs current practices but also inspires ongoing exploration in the field.
Mechanisms of Action
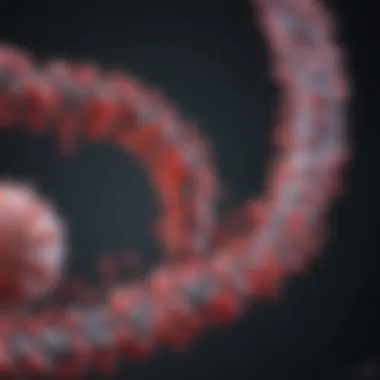
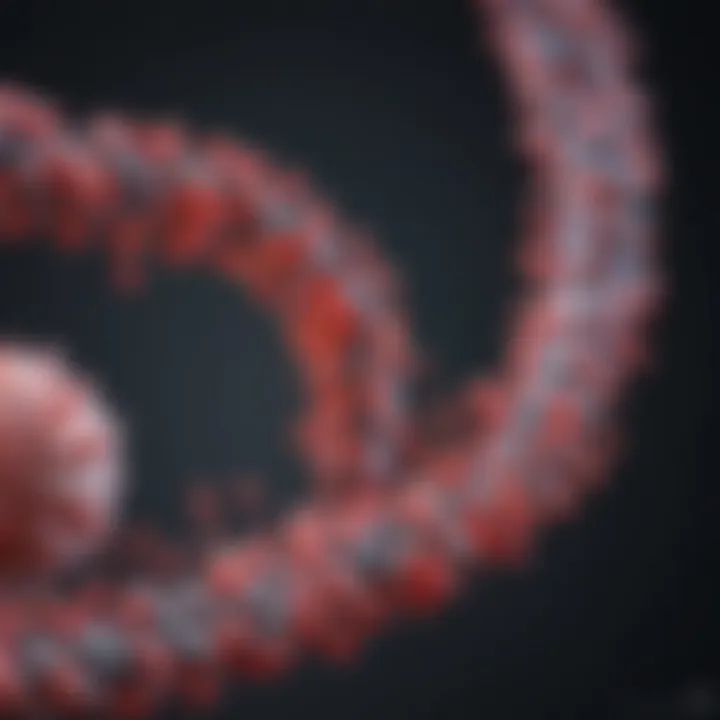
Understanding the mechanisms of action is essential for grasping how short hairpin RNA (shRNA) exerts its influence on gene regulation. This section delves into the key processes involved, allowing us to appreciate the transformative capabilities of shRNA in various fields, particularly in biomedical research and therapeutic settings. By dissecting these mechanisms, one gains insights into both the benefits and challenges associated with implementing shRNA technology.
RNA Interference Pathway
The RNA interference pathway is a crucial mechanism through which shRNA achieves its gene silencing effects. This pathway is initiated when shRNA is introduced into a cell, where it is processed into small interfering RNA (siRNA) by cellular machinery. The key players in this stage are Dicer and the RNA-induced silencing complex (RISC).
- Initiation of the Pathway:
Upon entering the cytoplasm, the double-stranded RNA structure of shRNA is recognized by Dicer, an RNase III enzyme. Dicer cuts the shRNA into 21-23 nucleotide long fragments, producing mature siRNA. - Formation of RISC:
The siRNA then associates with the RISC complex. The RISC, which contains Argonaute proteins, is responsible for guiding the siRNA to its complementary mRNA target. This step is vital since it determines the specificity of gene silencing. By scanning mRNA for complementary sequences, the RISC can selectively bind to and inhibit gene expression. - Gene Silencing:
Once RISC is guided to the target mRNA, it effectively cleaves the mRNA, preventing its translation into protein. This efficiently reduces the protein levels associated with the targeted gene, showcasing the power of shRNA in modulating gene expression with precision.
The importance of understanding this pathway cannot be overstated. It provides a framework for researchers looking to leverage shRNA in various applications, particularly where specific silencing of genes can lead to profound biological insights.
Dicer and RISC Complex Involvement
Dicer's role in processing shRNA into functional siRNA is pivotal. This enzyme’s ability to accurately and efficiently cleave shRNA requires precise enzymatic activity, which is fundamental to the effectiveness of shRNA knockdown. The integrity of Dicer is critical. Without its function, the initial step of the RNA interference pathway would not occur.
The RISC complex follows Dicer in this chain. RISC’s design ensures that only complementary mRNA strands are targeted, making it a selective tool for gene regulation. This selectivity is crucial to avoid off-target effects, which can lead to unintended consequences in the cellular environment.
Furthermore, the Argonaute proteins incorporated in RISC are integral for the subsequent enzymatic activity that leads to mRNA degradation. Their interaction with siRNA determines not only the accuracy of targeting but also the efficiency of gene silencing achieved through shRNA administration.
Understanding the roles of Dicer and RISC is fundamental for researchers who wish to optimize shRNA strategies for therapeutic and experimental applications. Any disruption in their function can compromise the entire gene silencing process.
In summary, the mechanisms of action of shRNA, particularly its involvement in the RNA interference pathway and the roles of Dicer and the RISC complex, demonstrate the intricacies of gene regulation. As we explore further applications and implications of shRNA, being aware of these mechanisms is essential for harnessing its full potential.
Designing Effective shRNA
Designing effective short hairpin RNA (shRNA) is crucial for maximizing the potential of RNA interference (RNAi) technology. This section discusses key elements in shRNA design, emphasizing the importance of thoughtful selection of target sequences and the incorporation of stabilizing elements. These considerations not only increase the efficacy of gene silencing but also help in minimizing potential off-target effects.
Choosing Target Sequences
The first step in effective shRNA design is the selection of target sequences. This choice is fundamental because the sequence must align precisely with the mRNA of the gene intended for silencing. The ideal target region is typically around 21–23 nucleotides long, allowing efficient binding to the complementary mRNA. Factors to consider when choosing target sequences include:
- Specificity: The target sequence should ideally be unique to the gene of interest to reduce off-target interactions. Computational tools, such as RNAi design software, can aid in predicting the specificity of designs.
- Accessibility: The target mRNA region should be located in a part of the transcript that is accessible and not obscured by secondary structures.
- Conservation: For sequences present in multiple homologous genes, consider designing shRNA that will selectively silence the desired target to avoid unintentional silencing of related genes.
Inclusion of mismatches or bulges can also help in fine-tuning the specificity and can lead to improved silencing effects in some cases.
Incorporating Stabilizing Elements
Incorporating stabilizing elements into shRNA constructs is essential for enhancing their stability and functionality in cellular environments. shRNA molecules can be subject to degradation by cellular nucleases, therefore, modifications are necessary to protect them. Key strategies may include:
- Chemical Modifications: 5' caps and 3' tails can be chemically modified to prevent degradation. Also, incorporation of phosphorothioate linkages can increase stability between nucleotides.
- Loop Structures: The design of stabilizing loop structures can enhance proper processing and increase the stability of shRNA.
- Delivery Vector Optimization: The delivery method can affect stability; non-viral vectors and viral vectors like lentivirus can be optimized for better maintenance of shRNA integrity post-delivery.
Effective design of shRNA relies heavily on these considerations, as they impact the overall success of gene silencing experiments and therapeutic applications. The strategic alignment of target sequence selection and incorporation of stabilizing modifications are not just technical duties but are pivotal in driving the innovation of gene regulation strategies in molecular biology.
"Designing effective shRNA is an indispensable skill for researchers, leading to impactful advancements in gene regulation and potential therapeutic applications."
It is clear that the ability to efficiently silence specific genes using shRNA will continue to be an essential area of focus in genetic research and therapy development.
Methods of Delivery
The delivery of short hairpin RNA (shRNA) is a critical factor in achieving effective gene silencing. This section discusses the different methods available for delivering shRNA into cells along with their specific characteristics and advantages. A well-chosen delivery method can significantly enhance the potency and specificity of shRNA, enabling researchers and clinicians to use this powerful tool in various applications, from molecular biology research to therapeutic interventions.
Viral Vectors
Viral vectors are one of the most efficient methods for delivering shRNA into target cells. Utilizing modified viruses, such as lentivirus, adenovirus, or adeno-associated virus, researchers can achieve high transfection rates. The primary advantage of viral vectors lies in their ability to infect a wide range of cell types, including dividing and non-dividing cells.
Key benefits of viral vectors include:
- High efficiency – They can effectively introduce shRNA into the cell’s genomic material.
- Stable integration – Some viral vectors allow for stable integration of shRNA into the host genome, facilitating long-term gene silencing.
- Easy modification – Templates for shRNA can be easily incorporated into the viral genome.
However, there are considerations like potential immunogenic responses and the need for specialized containment to work with certain viral systems. Ensuring that the viral vector is designed carefully to minimize off-target effects and unwanted immune reactions is essential. The success of using viral vectors often relies on the precise development and handling of these systems to maintain safety and efficiency.
Non-Viral Methods
Non-viral delivery methods are another crucial approach for introducing shRNA into cells. These methods encompass a variety of techniques that do not rely on viruses, making them attractive due to lower risk of immune reactions and easier scalability.
Some common non-viral delivery methods include:
- Liposome-mediated transfection – Liposomes encapsulate shRNA molecules, facilitating their fusion with cellular membranes and subsequent uptake by the target cells. This method can be tailored for different cell types.
- Electroporation – This method applies an electric field to create temporary pores in the cell membrane, allowing shRNA to enter the cell. It is particularly effective for hard-to-transfect cells.
- Microinjection – Direct injection of shRNA into the cytoplasm ensures high delivery efficiency, although it can be technically challenging for large-scale applications.
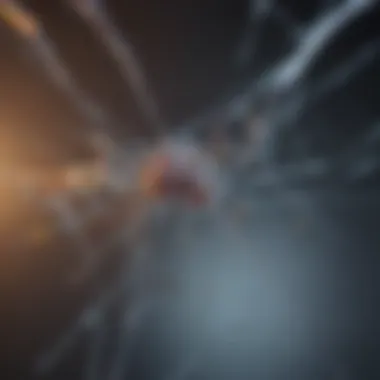
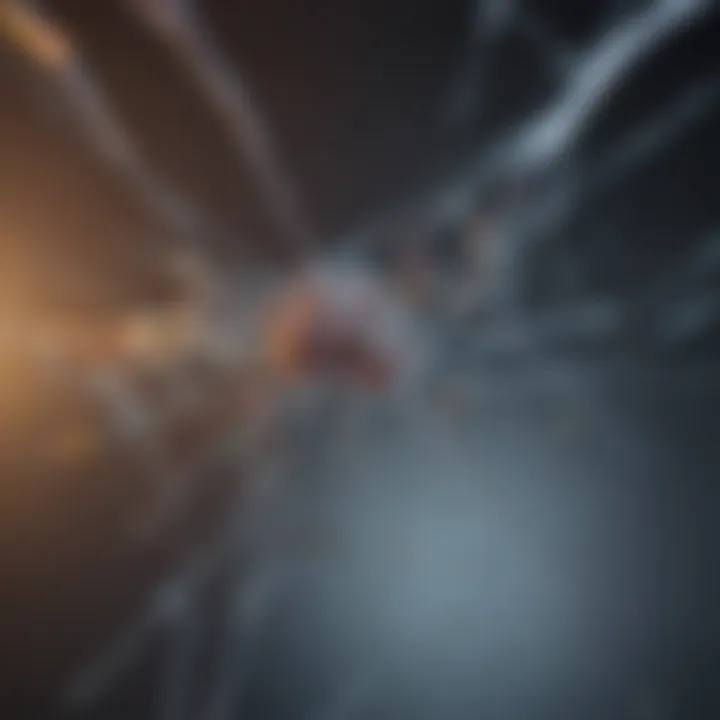
While non-viral methods offer significant advantages, they also have limitations, including potential cytotoxicity and variable transfection rates among different cell types. Researchers must weigh these factors when selecting the most suitable method.
The choice of delivery method can fundamentally alter the outcomes of shRNA experiments, emphasizing the need to evaluate each option rigorously.
In summary, both viral and non-viral delivery methods have their unique benefits and challenges. The effectiveness of shRNA applications in research and therapy substantially relies on selecting the appropriate delivery technique to achieve optimal gene silencing.
Applications in Research
The role of short hairpin RNA (shRNA) in research is pivotal. The ability to silence specific genes offers scientists powerful tools for understanding gene function and molecular mechanisms. This is particularly crucial in the realm of molecular biology, where unraveling the complexities of gene expression can lead to significant breakthroughs.
Gene Function Analysis
Gene function analysis is one of the primary applications of shRNA. Researchers utilize shRNA to systematically knock down specific genes, helping to determine their role in various biological processes. This method allows scientists to evaluate the effects of silencing a gene on cellular behavior, growth, and even apoptosis. By targeting genes of interest, researchers can uncover pathways that are vital for normal function or that contribute to disease.
For example, the study of cancer often involves knocking down oncogenes or tumor suppressor genes to analyze their impact on cell proliferation. The ability to observe changes at the phenotypic level following gene silencing enriches our understanding of tumor biology. Moreover, complex interactions among multiple genes can also be dissected, providing insight into regulatory networks.
Key considerations in gene function analysis include the selection of target sequences and the efficiency of the shRNA. Off-target effects need to be minimized to ensure that the observed phenotypes are indeed due to the intended gene knockdown. This necessitates careful design and validation of shRNA constructs.
Pathway Dissection
Pathway dissection is another critical application of shRNA in research. This involves understanding the signaling pathways that govern cellular functions and responses. By employing shRNA to silence genes within these pathways, researchers can delineate the roles of specific components in the signaling cascade.
For instance, silencing a particular kinase may help illustrate its effect on downstream signaling events. This can reveal how cellular responses to stimuli are modulated and the potential impact on disease states. The insights gained from pathway dissection can inform the development of targeted therapies and enhance our overall knowledge of cell biology.
Beyond just identifying signaling components, pathway dissection with shRNA allows for the investigation of complex interactions between different signaling cascades. Understanding how these pathways cross-communicate is essential for a comprehensive view of cellular behavior in health and disease.
"The systematic use of shRNA has transformed our approach to understanding gene function and molecular pathways, providing a clearer view of intricate biological processes."
In summary, the applications of shRNA in research are extensive. Through gene function analysis and pathway dissection, scientists can glean significant insights into the roles of genes and the signaling networks they operate within. Addressing the contemporary challenges, such as off-target effects and delivery methods, continues to refine the utility of shRNA, pushing the boundaries of what is possible in molecular biology.
Therapeutic Implications
The development of short hairpin RNA (shRNA) technology is pivotal in addressing various diseases through targeted gene silencing. Its therapeutic implications extend across multiple domains of medicine, especially in oncology and genetic disorders. Understanding the specific benefits of shRNA in therapeutic contexts is crucial for researchers and professionals aiming to enhance treatment efficacy and safety. This section discusses the capacity of shRNA to selectively silence oncogenes and its application in gene therapy approaches.
Targeting Oncogenes
Oncogenes contribute significantly to cancer progression by promoting uncontrolled cell growth. Utilizing shRNA to target these genes offers a novel strategy for cancer treatment. By designing shRNA that effectively inhibits the expression of specific oncogenes, researchers can potentially curb tumor growth and reduce metastasis.
shRNA can be tailored to match the sequence of the target oncogene. This specificity reduces the risk of affecting other genes, enhancing the treatment's safety profile. For instance, Bcl-2 is an oncogene associated with apoptosis inhibition in several cancers. Targeting Bcl-2 with shRNA allows for restoring the apoptotic process in cancerous cells.
In practice, shRNA can be delivered via viral vectors, enhancing cellular uptake and gene silencing efficiency. This method has shown promise in preclinical studies, with significant tumor regression observed in animal models when oncogenes are effectively silenced.
"The ability to selectively silence oncogenes presents a significant advancement in cancer therapeutics, offering hope in the fight against therapies resistant cancers."
This approach not only may improve patient outcomes but also enable the personalization of cancer therapy based on an individual's tumor profile. Additionally, using shRNA targeting oncogenes can support adjunctive therapies to enhance overall treatment effectiveness.
Gene Therapy Approaches
Gene therapy has emerged as a transformative approach in treating genetic disorders. shRNA plays a crucial role in this paradigm by providing a means to silence pathogenic genes responsible for disease manifestation.
For example, in conditions like cystic fibrosis, which results from mutations in the CFTR gene, shRNA can inhibit the expression of the mutant allele. This inhibition can help restore normal gene functions in affected tissues. Moreover, shRNA-based strategies can be tailored for various genetic disorders, providing a versatile tool in personalized medicine.
The concept of combining shRNA with existing gene therapy strategies has opened new avenues for treatment. In some protocols, shRNA is used to enhance the efficacy of gene editing techniques like CRISPR. Here, shRNA can be employed to downregulate compensatory pathways that might impede the success of gene editing.
As more insights are gained into the molecular underpinnings of genetic disorders, shRNA can be more accurately deployed. The integration of shRNA in gene therapy ultimately leads to more effective therapies, reducing disease burden and improving quality of life for patients.
Challenges and Limitations
In the fast-evolving field of molecular biology, challenges and limitations related to short hairpin RNA (shRNA) knockdown are critical to understand. As researchers explore shRNA's potential in gene regulation and therapeutic applications, it is essential to acknowledge the obstacles that could hinder its effectiveness. Identifying these challenges enables the scientific community to refine strategies, improve methodologies, and enhance overall outcomes in research and clinical settings.
Off-Target Effects
One significant issue with shRNA knockdown is off-target effects. These unintended interactions can lead to the silencing of genes other than the intended target. Off-target activity occurs when shRNA or its associated complexes bind to complementary RNA sequences, resulting in unpredictable biological consequences. Such effects can complicate the analysis of gene function and disrupt normal cellular pathways.
Effective design strategies can lessen off-target impacts. For instance, using algorithms to predict potential off-targets during the design phase helps researchers create more specific shRNA sequences. Additionally, testing shRNA in different contexts can identify potential unintended silencing. By addressing off-target effects, researchers can improve the reliability of findings and contribute to the safer application of shRNA in gene therapy.
Stability and Efficiency Issues
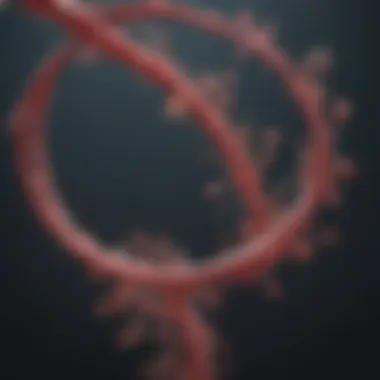
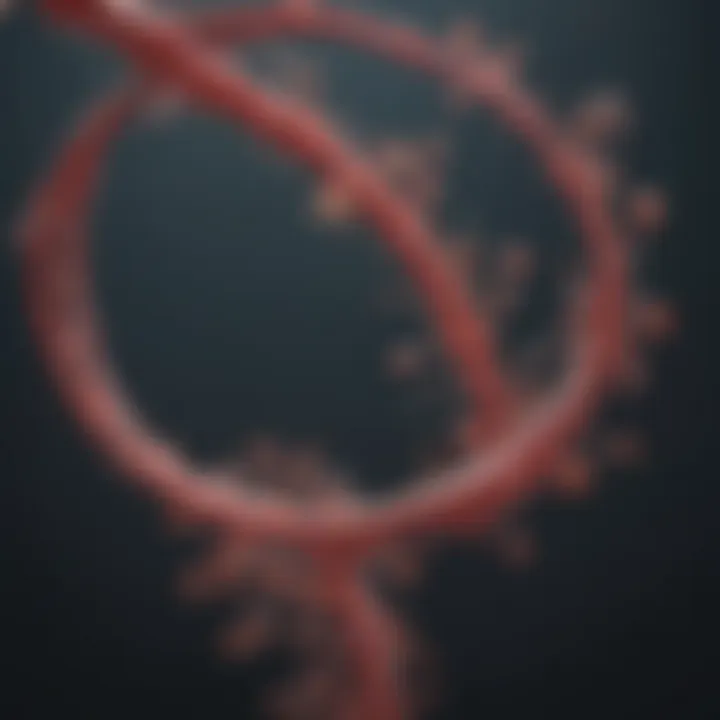
Another prominent challenge lies in stability and efficiency issues related to shRNA platforms. The stability of shRNA transcripts can be compromised in biological environments, leading to a reduced duration of gene knockdown. Factors influencing stability include the design of the loop region, the incorporation of stabilizing elements, and the method of delivery.
Efficiency is equally significant. The effectiveness of shRNA in knocking down a target gene varies widely, influenced by factors such as cellular context, expression levels, and tissue types. Variations can often result in inconsistent outcomes across experiments.
To enhance stability and efficiency, researchers are exploring novel design innovations and delivery methods. The incorporation of certain modifications, such as 2'-O-methyl or locked nucleic acid substitutions, can provide better stability. Additionally, optimizing delivery via viral vectors can ensure that the shRNA reaches its target efficiently, maximizing the therapeutic potential of gene knockdown strategies.
In summary, managing off-target effects and improving stability and efficiency are essential for advancing shRNA applications and ensuring successful outcomes in gene therapy.
By continuously tackling these challenges, the scientific community can foster a deeper understanding of gene regulation mechanisms, ultimately leading to more effective therapeutic interventions.
Future Perspectives
The exploration of short hairpin RNA (shRNA) technology holds significant promise for the future of genetic research and therapeutic development. This segment aims to address the key innovations expected in shRNA design and the emerging combinations of therapies that could enhance treatment efficacy. As the field continues to evolve, understanding these trends becomes crucial for scientists, researchers, and practitioners in molecular biology.
Innovations in Design
Innovations in the design of shRNA are crucial for improving gene silencing efficiency and specificity. Recent advancements focus on the development of more effective scaffolds, which can enhance the stability of shRNA and improve its delivery to target cells. One notable direction is the use of modified nucleotides. These modifications can boost the shRNA’s resistance to degradation while also enhancing its interaction with the RNA-induced silencing complex.
Other design approaches include the optimization of target sequence selection algorithms. Algorithms that predict potential off-target effects can help in designing shRNA with greater precision. Moreover, the use of computational modeling tools is becoming more prevalent, allowing for better pre-experimental evaluation of shRNA designs.
Incorporating shRNA into more sophisticated delivery mechanisms is vital as well. Nanoparticle-based delivery systems are gaining attention, as they can protect shRNA from degradation and facilitate targeted delivery. As research progresses, potential designs that combine shRNA with CRISPR technology may enable more robust gene-editing capabilities, leading to broader applications in genetic therapies.
Combination Therapies
Combination therapies involving shRNA are an emerging trend that offers potential advantages over traditional monotherapies. By integrating shRNA with other therapeutic modalities, including small molecule drugs, RNA-based therapies, or immunotherapy, researchers can tackle complex diseases more effectively.
Combining shRNA with chemotherapy has shown promise in oncological applications. For example, silencing specific oncogenes while concurrently administering chemotherapeutic agents may enhance the overall efficacy of cancer treatment. By reducing the expression of resistance genes, shRNA can sensitize cancer cells to the effects of chemotherapy.
Moreover, combining shRNA with immune checkpoint inhibitors holds the potential to boost anti-tumor immune responses. The dual approach could lead to better outcomes for patients, particularly those with resistant or advanced-stage cancers. As combination therapies unfold, ongoing clinical trials will be essential to evaluate their safety and efficiency.
"Future research should focus on the integration of shRNA into multi-pronged therapeutic strategies, combining shRNA with various modalities to enhance treatment outcomes and reduce side effects."
Ethical Considerations
Ethical considerations play a vital role in the development and application of short hairpin RNA (shRNA) technology. As researchers and scientists advance their understanding of gene regulation, it becomes increasingly important to address the ethical implications associated with manipulating genetic material. This section examines the regulatory framework and public perception surrounding shRNA technologies, as these factors significantly influence the practice of molecular biology and genetic engineering.
Regulatory Framework
The regulatory framework governing shRNA technology varies across regions and countries. Generally, it revolves around biosafety and bioethics. In the United States, the Food and Drug Administration (FDA) oversees the clinical applications, ensuring that gene therapies involving shRNA undergo thorough evaluation for safety and efficacy before approval. Similarly, in the European Union, complex guidelines exist that cover the use of genetically modified organisms (GMOs).
Regulations focus on aspects such as:
- Safety testing: Before any clinical use, thorough safety assessments are mandatory.
- Effectiveness: shRNA applications must be proven to target specific genes effectively, minimizing off-target effects.
- Public transparency: Involving public discourse helps in shaping attitudes toward new biotechnologies.
Thus, adherence to the regulatory frameworks not only mitigates risks but also builds public trust in shRNA technologies.
Public Perception
Public perception is another crucial element in the ethical landscape of shRNA applications. Genetic manipulation evokes diverse opinions, often shaped by cultural, ethical, and social factors. For some, the potential benefits of shRNA therapies in treating diseases like cancer outweigh ethical concerns. However, others may view these technologies as invasive or unpredictable.
Key factors influencing public perception include:
- Awareness and Education: Many individuals lack comprehensive knowledge about shRNA and its potential applications. Increased awareness can lead to more informed opinions.
- Media Representation: How biotech advancements are portrayed in the media can influence public attitudes, positive or negative.
- Ethical Considerations: Concerns about safety and the long-term effects of gene manipulation often heighten skepticism.
"Public opinion can significantly shape the direction of research and funding in the field of biotechnology. Understanding concerns can help align research with societal values."
Furthermore, the ethical dialogue around shRNA usage must consider diverse perspectives, engaging stakeholders in discussions that reflect social values and ethical norms. As the technology evolves, so must the understanding of its position within ethical norms.
Finale
The conclusion of this article emphasizes the significance of short hairpin RNA (shRNA) knockdown in scientific research and therapeutic applications. Understanding shRNA’s mechanisms provides insight into gene regulation, which is critical for both basic and applied biology. shRNA contributes to the landscape of molecular biology, offering researchers tools for gene silencing, leading to discoveries that can influence disease treatment and developmental biology.
Summary of Key Points
In summary, several key points outline the essential aspects of shRNA knockdown:
- Mechanisms of Action: Understanding how shRNA enters cells and silences genes is foundational. The RNA interference pathway is guided by components like Dicer and RISC, which facilitate gene regulation.
- Designing Effective shRNA: Effective shRNA requires careful selection of target sequences and potentially incorporating stabilizing elements to enhance stability and efficacy.
- Methods of Delivery: Both viral and non-viral methods play a role in delivering shRNA to target cells, each with distinct advantages and challenges.
- Applications in Research: shRNA has significant roles in gene function studies and dissection of biological pathways, proving to be a vital tool in molecular biology.
- Therapeutic Implications: Its potential in targeting oncogenes and innovative gene therapy approaches remains a fruitful area for exploration.
- Challenges: Recognizing limitations like off-target effects and stability issues is essential for developing more reliable shRNA applications.
- Future Perspectives: Continuous innovations in shRNA design and exploration of combination therapies signal a promising future in genetic engineering.
The Role of shRNA in Molecular Biology
shRNA plays a critical role in molecular biology by facilitating gene regulation. It serves as a powerful tool for scientists aiming to manipulate gene expression. The ability to create knockdown models enables deeper understanding of gene function, pathology, and therapeutic development. The applications are vast, ranging from basic research to potential treatment modalities for various diseases.
Through careful design and implementation, shRNA can significantly impact our understanding of genetic and molecular mechanisms. It presents opportunities and challenges, necessitating ongoing research to optimize its use in biological applications. As the field advances, shRNA is poised to remain an integral part of the toolkit for gene regulation, shaping the future of molecular biology and biotechnology.