Exploring the DNA Star: Structure, Function, and Implications
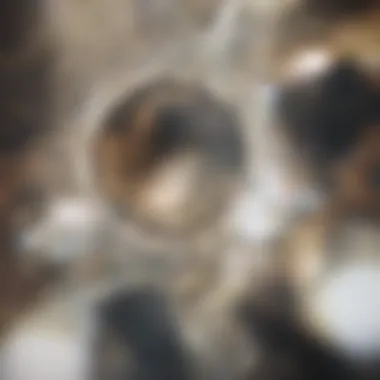
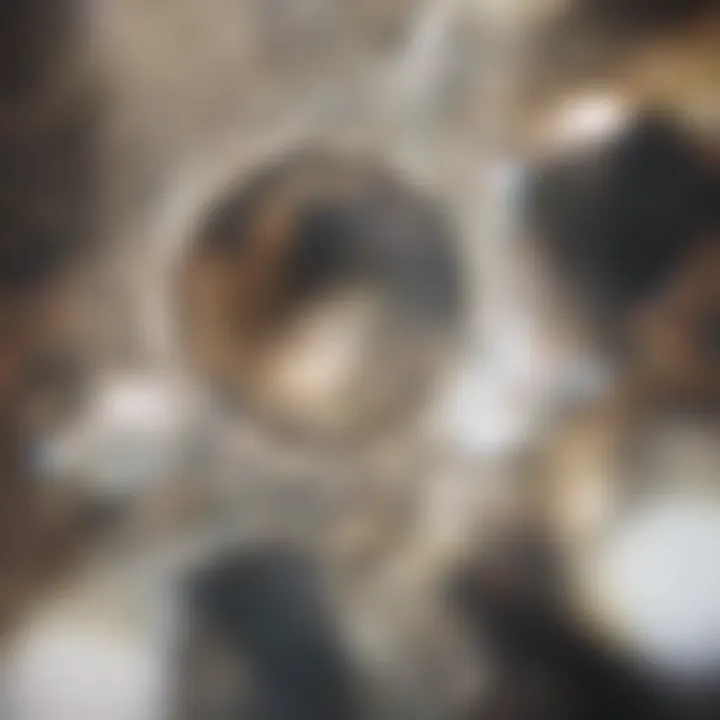
Intro
The DNA star epitomizes a fundamental element in the study of genetics and molecular biology. Its structure and function serve as crucial pillars in understanding how life operates on a cellular level. With the advent of biotechnological advancements, the implications of the DNA star have expanded, revealing its transformative potential in medicine, agriculture, and other fields. This article aims to explore these dimensions in-depth, offering insights that resonate with students, researchers, and professionals alike.
Methodologies
Description of Research Techniques
Exploring the DNA star requires a multifaceted approach. Common methodologies include X-ray crystallography, which provides detailed images of DNA structure, and Polymerase Chain Reaction (PCR), a technique essential for amplifying DNA segments for further analysis. These methods allow researchers to view intricacies within the DNA double helix, examining aspects like nucleotide sequences and structural variations.
Tools and Technologies Used
Several notable tools aid in DNA research. Sequencing technologies, such as the Illumina platform, enable high-throughput analysis of genetic material. Additionally, CRISPR-Cas9 has created revolutionary pathways in genetic editing, allowing for precise modifications at the DNA level. These technologies not only advance our understanding of genetic functions but also herald significant shifts in biotechnological applications.
Discussion
Comparison with Previous Research
Research concerning the DNA star has progressed significantly over the decades. Early studies established foundational understandings, whereas contemporary research builds upon these principles with advanced technologies. For example, previous research relied heavily on manual sequencing methods that were both slow and prone to error. Today's automated platforms provide rapid and accurate results, expanding the scope of genetic inquiries.
Theoretical Implications
The findings surrounding the DNA star raise essential questions regarding genetic engineering and synthetic biology. As we unravel the complexities of DNA, ethical considerations emerge regarding its use. Understanding the functions and implications of DNA ultimately shapes laws and policies that govern genetic research and its applications.
"The study of DNA offers a glimpse into the very essence of life. Its implications extend beyond science into ethics and society."
Intro to DNA
Understanding DNA is paramount for grasping the foundations of molecular biology and genetics. This section lays the groundwork for the subsequent exploration of the DNA star. By providing a detailed overview of the definition, historical context, and its vital role in biological systems, we illuminate why DNA is not just a biological molecule but a cornerstone of life itself.
Definition and Composition
DNA or deoxyribonucleic acid is the hereditary material found in all living organisms and many viruses. Its structure consists of two long strands forming a double helix, which is stabilized by base pairs. These bases—adenine, thymine, cytosine, and guanine—pair specifically: adenine with thymine and cytosine with guanine. This pairing mechanism is fundamental as it enables the accurate replication of genetic information. The backbone of DNA is composed of sugar and phosphate groups. This arrangement allows for the molecular stability necessary for the integrity of genetic information across generations.
Historical Context of DNA Research
DNA research has a rich historical context that spans several decades. The discovery of the structure of DNA by James Watson and Francis Crick in 1953 was a pivotal moment in genetics. This breakthrough was built upon the earlier works of many scientists, including Rosalind Franklin, who provided critical X-ray diffraction images of DNA. The understanding of DNA's role in heredity emerged slowly but steadily through the 20th century. Each discovery paved the way for advancements in genetic research, ultimately leading to significant milestones, such as the Human Genome Project, which mapped the entire human DNA sequence.
Importance in Biological Systems
The significance of DNA in biological systems cannot be overstated. It carries the genetic blueprint that dictates cellular function, development, and behavior of an organism. Without DNA, there would be no proteins, enzymes, or cellular structures that maintain life. Furthermore, DNA is crucial for evolution through mutation and genetic variation. It enables adaptation and survival in changing environments. Understanding DNA's functions hence allows researchers to delve deeper into topics like genetics, biotechnology, and medicine.
"The study of DNA has transformed our understanding of biology, medicine, and even ancestry, revealing connections that were previously obscured."
In summary, this section delineates the essentials of DNA, preparing the reader for a deeper discussion on the DNA star and its implications across various scientific fields.
Understanding the DNA Star Concept
The concept of the DNA star is a pivotal aspect in the field of molecular biology and genetics. Understanding this structure is essential for grasping how DNA functions within living organisms. The DNA star serves as a metaphorical depiction of the intricate interactions within the molecular framework that give rise to genetic expression and function. By delving into this concept, researchers can better comprehend the role that DNA plays in various biological processes, from inheritance to cellular regulation.
Studying the DNA star also helps bridge gaps between theoretical models and empirical observations. It encourages interdisciplinary research, integrating insights from bioinformatics, molecular biology, and genetics. By fostering collaboration among these fields, the understanding of the DNA star can lead to breakthroughs in biotechnology and medicine. The implications of such research are vast, affecting everything from gene therapy to agricultural advancements.
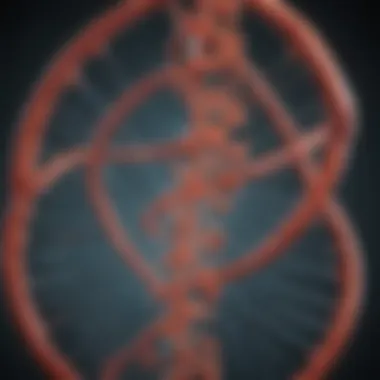
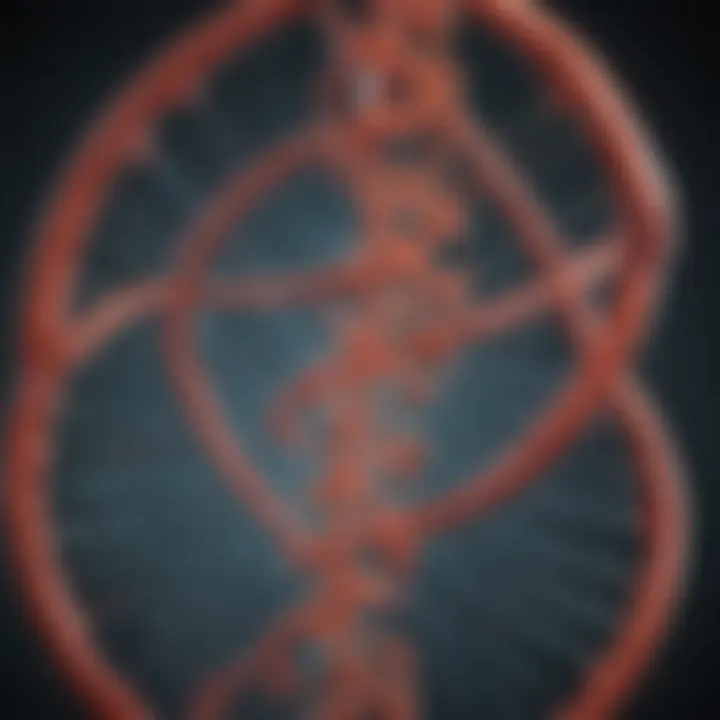
What is a DNA Star?
A DNA star is not an official term in the scientific literature but is often used to illustrate intricate DNA structures and their functionalities. It represents the concept of how the DNA double helix can be perceived as a star-like structure at a molecular level, when viewed from certain angles and with specific modifications or arrangements.
In essence, the DNA star underscores the complexity of genetic material. It emphasizes how various structural components—like nucleotides, base pairs, and helical formations—converge to form a cohesive and functional unit. This imagery or framework aids students and researchers in visualizing the interconnectedness of DNA’s roles.
Theoretical Framework Behind the DNA Star
Theoretical models surrounding the DNA star combine various scientific principles ranging from chemistry to genetics. These models help elucidate the structural dynamics of DNA and its operating mechanisms. For example, the sequence of nucleotides in DNA dictates how it folds and interacts with proteins, influencing gene expression.
- Structural Biology: Understanding molecular structures aids in predicting how changes in DNA sequences may affect functionality.
- Bioinformatics: Computational tools analyze DNA sequences and structures, revealing patterns that correlate with biological functions.
- Genetic Theory: This highlights how mutations or variations in DNA can result in differences in phenotype and biological trait expression.
Role of the DNA Star in Genetic Expression
The DNA star, through its complex structure, plays a critical role in genetic expression. Genetic expression refers to the process by which information from a gene is used to synthesize functional gene products, like proteins. The arrangement and interaction of DNA within the star framework influence how genes are activated or silenced.
Through several mechanisms, the DNA star contributes significantly to gene regulation:
- Promoter Regions: These areas on the DNA are essential for initiating transcription, affecting how genes express themselves.
- Enhancers: These are sequences that can increase the likelihood of transcription when bound by specific proteins, allowing greater versatility in gene expression.
- Repressors: These proteins can bind to DNA regions to inhibit transcription, demonstrating the fine balance required for proper cellular functioning.
Understanding how the DNA star influences genetic expression is vital for advancements in genetic engineering, medicine, and biotechnology. As research progresses, insights derived from studying the DNA star can lead to innovative strategies to manipulate gene expression, ultimately benefiting various fields of science.
Structural Characteristics of DNA
Understanding the structural characteristics of DNA is essential in grasping its function and implications. The architecture of DNA not only determines how information is stored but also dictates how it is expressed and regulated within living organisms. The unique properties of DNA facilitate complex biological processes, including replication and transcription. By investigating how its structure contributes to these functions, one gains insight into its central role in molecular biology and its potential applications in biotechnology.
Double Helix Formation
The double helix is one of the most iconic structures in biology. Discovered by James Watson and Francis Crick in 1953, this winding ladder-like formation is shaped by two strands of nucleotides that coil around each other. Each nucleotide consists of a deoxyribose sugar, a phosphate group, and a nitrogenous base. The bases pair specifically—adenine with thymine, and guanine with cytosine—through hydrogen bonds.
The stability of the double helix is significant. This stability allows the DNA to maintain its structure while being subjected to various cellular processes. Moreover, the helical nature of DNA ensures that the strands can separate during replication and transcription without significant disruption. The introduction of supercoiling allows DNA to fit inside the nucleus while remaining tightly packaged.
Nucleotide Composition and Function
Nucleotides are the building blocks of DNA. Each nucleotide includes a phosphate, a sugar, and a base. The order of these bases encodes genetic information. There are four types of nitrogenous bases: adenine, thymine, guanine, and cytosine. The specific arrangement of these bases creates a unique sequence that can represent instructions for building proteins and other molecules essential for life.
The function of nucleotides extends beyond mere structural contribution. They play roles in signaling pathways and energy transfer processes, such as ATP, which is a nucleotide-derived energy carrier in cells. In addition, certain nucleotides serve as precursors in the synthesis of RNA and other important biological molecules.
Gene Localization within the Structure
Genes are segments of DNA that contain the instructions for producing proteins. Their localization within the DNA structure is essential for proper gene expression and regulation. Each gene is located at a specific site within the double helix. This spatial organization allows for efficient regulation and interaction with other cellular molecules.
Regulatory structures adjacent to genes, known as promoters and enhancers, help control when and how genes are expressed. This organization ensures that genes can be activated or silenced in response to cellular signals. Furthermore, epigenetic modifications, such as methylation, can influence gene localization and accessibility, ultimately affecting gene activity without altering the underlying DNA sequence.
In summary, the structural characteristics of DNA are fundamental to its functionality in biological systems. The double helix formation serves as a protective framework, the nucleotide composition encodes information, and gene localization facilitates regulation and expression. Understanding these aspects contributes to advancements in genetic engineering and biotechnological applications.
Functionality of DNA
The functionality of DNA is fundamental to understanding its role in life. DNA is not merely a passive repository of genetic instructions; it actively engages in numerous processes vital for cellular integrity and organismal development. This section explores the mechanisms through which DNA operates, emphasizing its significance in replication, gene expression, and the regulation of cellular activities. By examining these elements, we can appreciate the breadth of DNA's contributions to biological systems and their implications for advancements in science and medicine.
DNA Replication Process
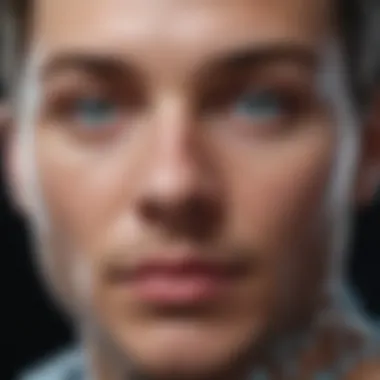
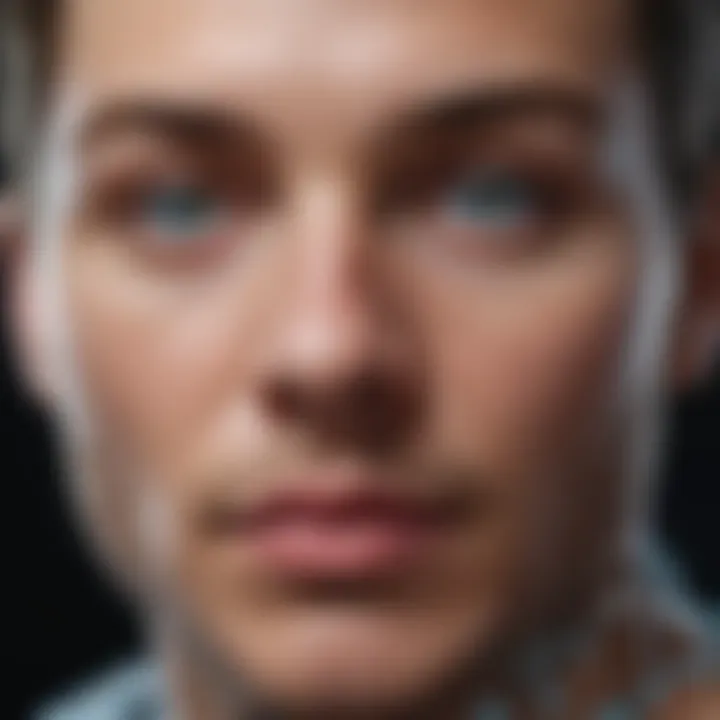
The replication of DNA is a crucial process that ensures genetic information is accurately passed down from one generation to the next. This process occurs during the S phase of the cell cycle and involves the unwinding of the double helix structure. Enzymes such as DNA polymerase play an essential role in synthesizing new strands of DNA by adding complementary nucleotides to each template strand.
- Initiation: Specific sites known as origins of replication are recognized by initiator proteins. These proteins assist in unwinding the DNA.
- Elongation: DNA polymerases add nucleotides to the growing daughter strands. The new strands are synthesized in a 5' to 3' direction, creating two identical copies of the original DNA molecule.
- Termination: Once the entire DNA molecule is duplicated, replication halts, and the result is two double helices, each containing one old strand and one newly synthesized strand. This process is referred to as semiconservative replication.
Understanding DNA replication is critical, as errors can lead to mutations, which may have dire consequences for the organism.
Transcription and Translation Mechanisms
Transcription and translation are two vital processes that illustrate how DNA governs protein synthesis.
- Transcription occurs in the nucleus, where a particular segment of DNA is transcribed into messenger RNA (mRNA). This initiation occurs at specific promoter regions of active genes. RNA polymerase is the key enzyme that synthesizes RNA by reading the template strand of DNA. The mRNA then undergoes modifications such as splicing and capping to become mature mRNA, ready for translation.
- Translation takes place in the cytoplasm, where ribosomes read the mRNA sequence and synthesize proteins. Transfer RNA (tRNA) molecules bring the corresponding amino acids to the ribosome, allowing the assembly of proteins based on the mRNA sequence. The sequence of nucleotides dictates the sequence of amino acids, ultimately determining the structure and function of the resulting protein.
These processes exemplify DNA's role as a blueprint, guiding the synthesis of proteins that perform various functions across biological systems.
Role in Cellular Regulation
DNA not only encodes genetic information but also plays a pivotal role in regulating cellular processes. Gene expression is tightly controlled by various factors, including transcription factors and regulatory elements such as enhancers and silencers. These elements ensure that genes are expressed at the right time and level, adapting cellular functions to internal and external stimuli.
- Homeostasis: Through feedback mechanisms, DNA expression patterns adjust cellular actions to maintain homeostasis within the individual.
- Response to Stress: Under stress conditions, certain genes can be activated or repressed to help the cell cope with the challenge, highlighting DNA's role in survival.
"Understanding how DNA regulates cellular processes reveals insights into diseases and potential therapeutic targets."
Ultimately, the functionality of DNA encompasses complex processes that are essential for life. Through its intricate systems of replication, transcription, translation, and regulation, DNA serves as the cornerstone of biological organization and adaptive capacity.
Implications of DNA Research
The realm of DNA research extends far beyond basic understanding; it influences a variety of fields ranging from medicine to agriculture. The implications of this research can reshape our society and have significant ramifications on how we perceive and interact with life itself. Understanding the implications of DNA is crucial, as it can lead to enhanced health outcomes, revolutionize agricultural practices, and spark debate regarding ethical standards in biotechnology.
Applications in Biotechnology
Biotechnology thrives on the foundation laid by DNA research. The ability to manipulate DNA allows scientists to create genetically modified organisms (GMOs), which can result in crops that are pest-resistant or have enhanced nutritional profiles. Such advancements address food security concerns, especially in regions facing climate change effects.
DNA applications continue to evolve in medical biotechnology as well. Techniques such as CRISPR-Cas9 enable precise genetic modifications that can correct genetic disorders. Gene therapy is transforming the treatment of diseases, offering potential cures for previously incurable conditions. The impact of these applications is profound, offering tools for fighting diseases at their genetic causes.
Advancements in Genetic Engineering
Genetic engineering is a vital branch of DNA research, marked by rapid innovations. One of the most notable advancements is the development of synthetic biology, which allows for the design of biological systems that do not exist in nature. This can lead to the production of biofuels, pharmaceuticals, and even materials with unique properties.
Moreover, the advent of genome sequencing technologies has dramatically lowered the cost and time required to sequence DNA. This accessibility has propelled personalized medicine, whereby treatments can be tailor-made for individual genetic profiles. The importance of these advancements cannot be overstated, as they offer pathways to enhance survival and quality of life.
Ethical Considerations in DNA Manipulation
As we delve into DNA manipulation, ethical concerns become prominent. The power to modify genetic material raises questions about the potential for designer babies and the unforeseen consequences on future generations. Decisions made in the lab can have societal-wide implications, influencing genetic diversity and altering the human gene pool.
Ethics in DNA manipulation also concerns the ownership of genetic information. Issues arise on who has the right to access and use one’s genetic data, bringing up concerns of privacy and consent. Furthermore, potential misuse of genetic technologies in biowarfare or discrimination based on genetic attributes is a real and alarming thought.
"When we discuss DNA research, we are not just talking about science; we are addressing the very fabric of life itself."
Future Directions in DNA Research
The field of DNA research is continually evolving. Scientists and researchers are constantly exploring new avenues that hold potential for groundbreaking discoveries. In this section, we will explore several critical aspects of future directions in DNA research, focusing on emerging technologies, the understanding of genetic diseases, and enhancing agricultural practices. Each of these elements carries significant implications for medicine, biotechnology, and environmental sustainability.
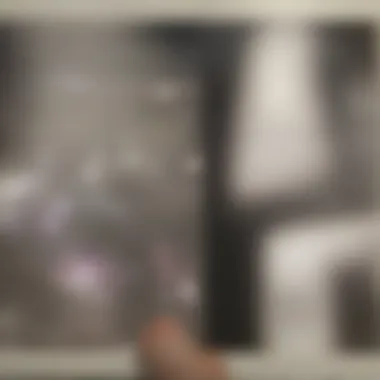
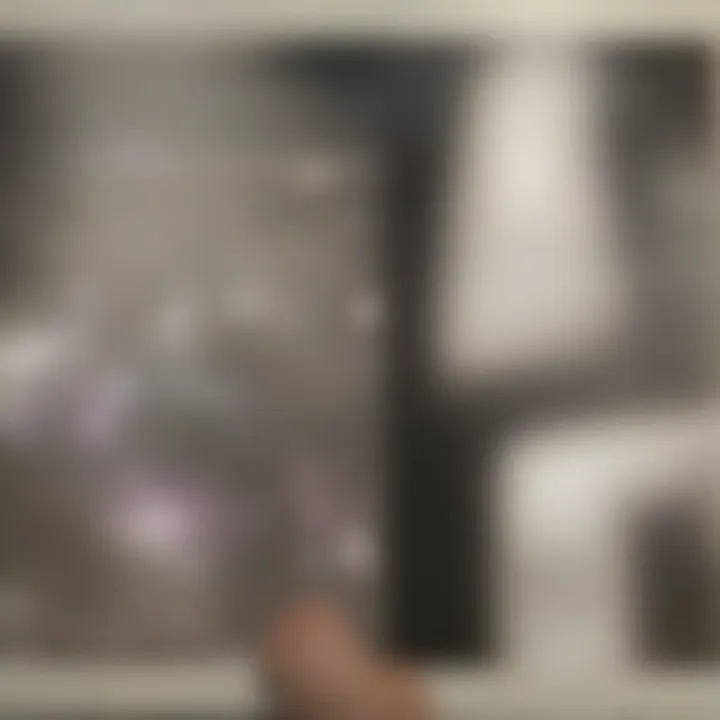
Emerging Technologies in Gene Studies
Recent advancements in technology are shaping the future of gene studies. New tools and methods are becoming available, streamlining genetic research. For example, CRISPR-Cas9 technology has revolutionized gene editing, providing precise alterations to DNA sequences. This allows researchers to study gene functions more efficiently.
Several other technologies are also on the horizon:
- Next-generation sequencing (NGS): This method offers rapid sequencing of DNA, significantly reducing the time and cost associated with traditional sequencing methods.
- Single-cell sequencing: This technology permits the analysis of individual cells, allowing scientists to understand cellular diversity better and identify rare genetic variants.
- Artificial intelligence in genomic analysis: AI algorithms can sift through vast data sets, identifying meaningful patterns and relationships in genetic data.
These innovations will undoubtedly enhance our understanding of genetic mechanisms and the broader implications for health and disease.
The Quest for Understanding Genetic Diseases
The quest to understand genetic diseases remains a primary focus in DNA research. Identifying genetic mutations associated with various conditions can lead to significant breakthroughs in treatment and prevention. Research in this area aims to provide insights into the genetic basis of diseases like cystic fibrosis, sickle-cell anemia, and various forms of cancer.
The importance of this understanding includes:
- Personalized medicine: Knowing an individual's genetic makeup can help tailor medical treatments specific to their needs.
- Early detection: Identifying genetic risk factors can lead to earlier interventions, potentially preventing the onset of diseases.
- Gene therapy: Advances in gene editing provide the opportunity to correct mutations, offering hope for curing genetic disorders.
Continued investigation into the genetics of diseases offers a promising path to health breakthroughs.
Potential for Enhancing Agricultural Practices
Agricultural practices too can benefit from advancements in DNA research. With the increasing demand for food and the effects of climate change, improving crop resilience and yield is vital.
Key areas for agricultural enhancement include:
- Genetically modified organisms (GMOs): Genes can be modified to create crops that withstand pests, diseases, and environmental stresses.
- Marker-assisted selection: Researchers can identify desirable traits in plants using specific DNA markers, speeding up the breeding process.
- Synthetic biology: This emerging field aims to design and construct new biological parts to improve crop efficiency and produce sustainable food sources.
Enhancing agricultural practices through DNA research will be critical in ensuring food security and sustainability for future generations.
In summary, the future of DNA research is poised for significant advancements. The combined potential of emerging technologies, breakthroughs in understanding genetic diseases, and innovations in agricultural practices holds the promise of transformative impacts in various fields.
The End
The conclusion of this article serves as a vital reflection on the multifaceted nature of DNA, especially the concept of the DNA star. This section emphasizes key findings, their implications in various fields, and the thought-provoking questions regarding future research.
Summarizing Key Findings
Throughout this article, we have explored several critical aspects of DNA and its role within biological systems. The structural characteristics, such as the double helix formation, have been noted to provide not just stability but functional capabilities that are essential for genetic expression. We discussed the functionality of DNA in processes like replication and transcription, highlighting how it is integral in cellular activities.
- The DNA star concept introduces a new perspective in understanding genetic functionality.
- Structural elements of DNA contribute significantly to its stability and efficiency in carrying genetic information.
- Advancements in biotechnology reveal practical applications born from deep DNA research.
Importantly, the ethical considerations associated with DNA manipulation cannot be overlooked. The implications of altering genetic materials raise questions that society must address as technology advances.
Industry Implications
In industry, the ramifications of DNA research are profound. Fields such as biopharmaceuticals, agriculture, and forensic science benefit profoundly.
- Biotechnology: Enhanced techniques in genetic modifications help in developing better pharmaceuticals.
- Agricultural practices: Genetically modified organisms (GMOs) offer benefits like increased yield and reduced pesticides.
- Healthcare advancements: With the understanding of genetic diseases, targeted therapies are becoming a norm.
These advances offer tangible benefits, pushing industries toward innovative solutions, while also necessitating a careful approach towards ethical concerns.
Looking Ahead: The Future of DNA Research
Looking ahead, the future of DNA research holds vast potential. Emerging technologies, such as CRISPR-Cas9, are revolutionizing our ability to edit genetic material precisely. The quest for understanding genetic diseases is gaining momentum, with efforts focused on mapping entire genomes. Agricultural practices might also see transformations aimed at sustainability and resilience.
- Ongoing research will strive to unravel the complexities of genetic diseases, paving the way for more effective treatments.
- Sustainability in agriculture through genetic improvements may lead to food security.
- Interdisciplinary collaborations will foster advancements, integrating insights from molecular biology, ethics, and technology.
This exploration of DNA emphasizes the necessity for continuous research to optimize benefits while addressing the ethical dilemmas presented by such powerful tools. The implications of these studies extend beyond the laboratory, impacting our society and future generations.