Exploring Bacterial Plasmids in Genetic Engineering
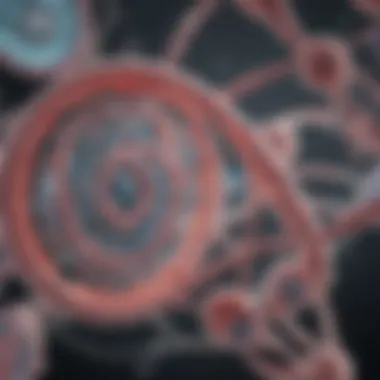
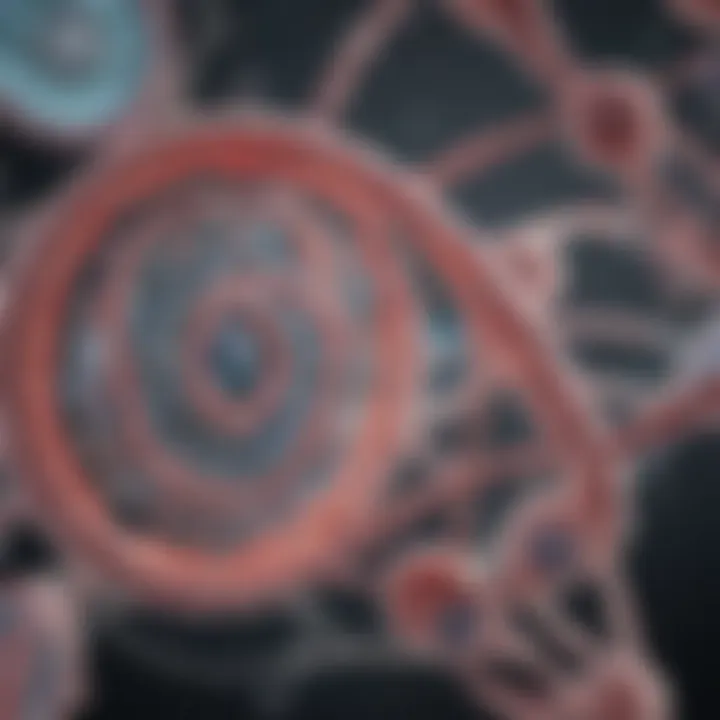
Intro
Bacterial plasmids often fly under the radar in discussions about genetic engineering, yet their impact is profound. These small, circular DNA molecules work independently of the chromosomal DNA found in bacteria. It’s remarkable how such tiny structures can hold massive significance in the realm of genetics. Imagine a toolbox with versatile tools—this is what plasmids represent in biotechnology. They facilitate various processes, making them essential for scientists and genetic engineers alike.
In exploring the role and functions of plasmids, we’ll peel back the layers, so to speak, and examine their structures, functions, and practical applications. The journey will take us through the nuances of how these molecular entities contribute to genetic diversity and antibiotic resistance. It’s a complex interplay, presenting both opportunities and challenges.
Through this narrative, I aim to highlight the importance of plasmids and how they act as critical components in cloning, expression systems, and gene delivery. By synthesizing findings from current studies, the goal is to provide a comprehensive overview that resonates not only with researchers and educators but also with enthusiasts keen on understanding modern genetics and microbiology.
Indeed, as we dive deeper, the intricate world of microbial genetics will unfold, illuminating the myriad ways in which plasmids shape the future of genetic engineering.
Intro to Bacterial Plasmids
In the realm of microbiology and genetic engineering, bacterial plasmids play a pivotal role that can't be overlooked. These small, circular DNA molecules serve as the backbone of various genetic manipulations that scientists carry out in laboratories around the globe. Understanding their significance not only aids in the improvement of biotechnological applications but also unravels the complexities underlying genetic diversity and the mechanisms of evolution in bacteria.
Bacterial plasmids are like the Swiss Army knives of bacterial genetics. They carry genes that can confer advantageous traits to bacteria, such as antibiotic resistance or the ability to metabolize unusual substrates. This versatility is what makes them incredibly useful in research and industry, allowing for the exploitation of their functions in innovative ways. The ability to manipulate plasmids means researchers can create tailored organisms to produce specific proteins, develop new medications, or even create biofuels.
As we delve deeper into the defining features and historical context of plasmids, it’s essential to appreciate how their unique attributes have vast implications for modern science. The narrative of bacterial plasmids is not just about DNA; it's also a story about survival, adaptation, and the relentless pursuit of knowledge in the face of biological complexities.
"Bacterial plasmids open new doors in the world of genetics, giving scientists the ability to redefine the boundaries of innovation."
By clarifying what plasmids are and tracing their journey from discovery to their current applications, this article aims to provide a thorough understanding of their roles and functions in genetic engineering.
Defining Plasmids
Plasmids are essentially mobile genetic elements existing separately from the chromosomal DNA within bacterial cells. They can replicate independently and carry genes that are often beneficial for the bacteria. At their core, these plasmids consist of double-stranded DNA, typically ranging from a few thousand to several hundred thousand base pairs in length.
What sets plasmids apart from chromosomal DNA is their ability to exist in multiple copies per cell and their ease of transfer between bacteria. This is particularly relevant in natural settings, where they can carry traits that allow for rapid adaptation to changing environments. Some essential characteristics of plasmids include:
- Independence: They can replicate without relying on the bacterial chromosome.
- Mobility: They can move from one bacterium to another through processes such as conjugation, transformation, or transduction.
- Diversity of Functions: Depending on their structure and gene content, plasmids can serve various roles, from antibiotic resistance to metabolic capabilities.
Historical Context
The story of plasmids began in the early 1950s when discovery and exploration of these DNA elements revolutionized our understanding of genetics. The first plasmid, known as R plasmid, was discovered in a strain of Escherichia coli in an investigation of antibiotic resistance. This sparked tremendous interest in bacterial genomics, as people started to recognize the importance of plasmids in natural selection and adaptation.
As research progressed, scientists established plasmids as essential components in molecular biology. The practical applications began to unfold in the 1970s when recombinant DNA technology emerged. This led to the development of plasmid vectors that facilitated the cloning of genes, allowing scientists to manipulate DNA more efficiently.
The increasing awareness of plasmids' roles in gene transfer and expression solidified their importance in biotechnological advancements. Over the decades, although the technology has evolved, bacterial plasmids remain integral to genetic engineering, enabling scientists to explore new frontiers such as synthetic biology and gene therapy.
In summary, the journey of bacterial plasmids reflects the dynamic nature of scientific inquiry and the continuous quest for understanding complex biological systems. As we explore their structural characteristics, biological functions, and various applications in biotechnology, we aim to provide a complete perspective on why plasmids are indispensable tools in genetic engineering.
Structural Features of Bacterial Plasmids
Understanding the structural features of bacterial plasmids is fundamental for comprehending their roles and applications in genetic engineering. These tiny circular DNA fragments, distinct from chromosomal DNA, are vital for the adaptability of bacteria. Their structure enables a variety of functions, and their nuances set them apart, making them indispensable in biotechnology and many scientific pursuits.
Basic Structure
Plasmids generally consist of two essential components: the origin of replication and the antibiotic resistance genes, along with other accessory genes that play specific roles. The origin of replication is crucial because it dictates how the plasmid duplicates itself within the host cell. The ease of replication is what gives plasmids their unique edge— they can replicate independently of the bacterial chromosomal DNA, allowing them a level of autonomy.
Additionally, plasmids can contain multiple genes, which can code for various functions, including those that help the host withstand antibiotics or enable the breakdown of unusual substrates. This complexity in their design allows plasmids to adapt swiftly to environmental changes and bacterial competition, making them a focal point in the study of genetic manipulation.
Types of Plasmids
Plasmids are not all cut from the same cloth; they come in different shapes and sizes, each tailored to specific tasks. Understanding these types is key when applying them to genetic engineering. Here are the primary categories:
Conjugative Plasmids
Conjugative plasmids are pivotal in the transfer of genetic material between bacteria. They possess genes that facilitate the process of conjugation, a kind of bacterial mating that allows one bacterium to transfer plasmid DNA to another through direct contact. This method of transfer is especially important for spreading advantageous traits, such as antibiotic resistance, within microbial populations.
A notable characteristic of conjugative plasmids is the presence of pilus formation genes, which assist in attaching to recipient cells. This feature is beneficial because it promotes genetic diversity among bacteria, making these plasmids extremely popular in genetic engineering, where transferring genes between organisms is often desired. However, a downside may be the potential for creating multi-resistant bacterial strains, raising significant public health concerns.
Non-Conjugative Plasmids
On the flip side, non-conjugative plasmids lack the machinery necessary for self-transfer. While they cannot spread through conjugation, they can still replicate within a host cell. The lack of transfer capability might seem like a disadvantage, but it also allows these plasmids to remain stable in various environments, as they do not contribute to the rapid distribution of drug resistance across different bacterial strains.
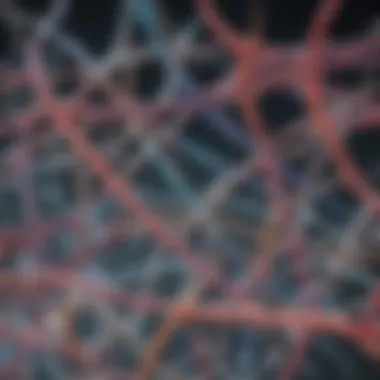
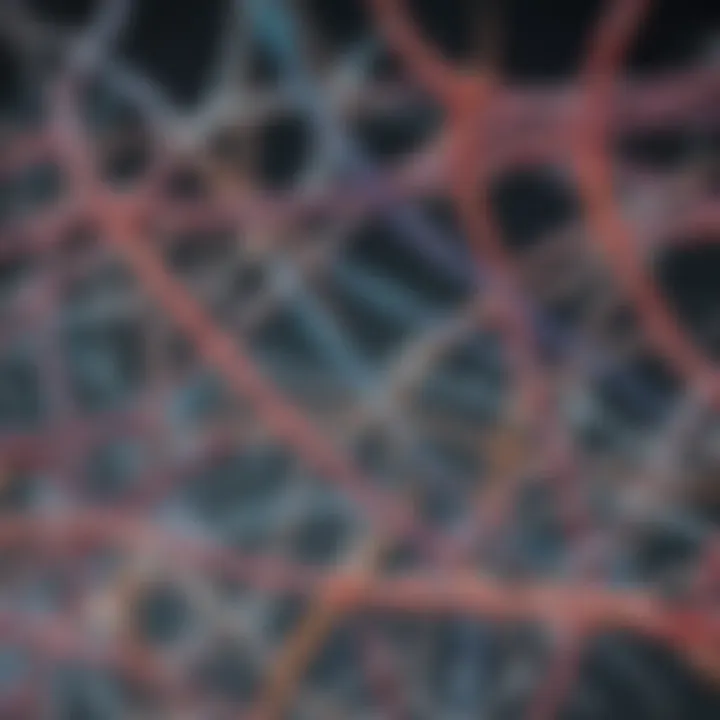
In this article, non-conjugative plasmids are highlighted for their utility in research and therapeutic applications, as they can be used to carry genes without risking the rampant spread of antibiotic resistance. They’re particularly useful in controlled laboratory settings, where researchers want to study specific genes without unintended consequences.
R Plasmids
R plasmids, short for resistance plasmids, are perhaps one of the most well-known types among bacterial plasmids due to their role in conferring antibiotic resistance. These plasmids often carry multiple resistance genes, making them formidable foes against antibiotic treatments. The characteristic that sets R plasmids apart from others is their ability to evolve quickly, often acquiring genes from surrounding bacteria, which makes them particularly challenging to control in clinical settings.
One significant advantage of R plasmids is their rapid adaptability to environmental pressures, such as the presence of antibiotics. However, this adaptability comes at a cost; their spread can lead to difficult treatment outcomes for infections, raising concerns not just in medicine but also in public health.
In summary, the structural features of bacterial plasmids are not merely academic curiosities; they provide the foundation for understanding how bacteria develop and share traits that can impact human health and biotechnology. Each type of plasmid, whether conjugative, non-conjugative, or R plasmids, highlights the versatility and importance of these genetic tools in the ongoing advancements in genetic engineering.
Biological Functions of Plasmids
Bacterial plasmids perform an array of biological functions that are critical not only to the survival of the bacteria that harbor them but also to the applications in genetic engineering and biotechnology. Understanding these functions can give us insight into how plasmids can be manipulated for various uses in medicine and research. Their significance can’t be overstated—plasmids are the unsung heroes of genetic diversity and adaptability in microbial populations.
Plasmids contribute primarily through two major functions: granting antibiotic resistance and enhancing metabolic diversity among bacteria. These functions play an essential role in natural selection, allowing bacteria to thrive in changing environments where resources or threats are unpredictable. Both factors have major implications for human health and biotechnology.
Role in Antibiotic Resistance
Plasmids are often the carriers of genes that confer antibiotic resistance, a major concern in treating bacterial infections. When plasmids carry these resistance genes, they can be transferred from one bacterium to another through processes such as conjugation, transformation, or transduction.
Mechanisms that describe how plasmids confer antibiotic resistance include:
- Gene Transfer: When a bacterium containing a resistant plasmid encounters a susceptible one, it can transfer the plasmid through physical contact (conjugation). This means resistance can spread rapidly across bacterial populations.
- Efflux Pumps: Some plasmids encode proteins that can pump antibiotics out of the cell, effectively reducing drug concentration inside the bacterium.
- Modification Enzymes: Certain plasmids produce enzymes that chemically modify antibiotics, rendering them ineffective.
The rapid dissemination of antibiotic resistance through plasmids has serious implications for public health. Notably, the emergence of multi-drug resistant strains of bacteria has led to public health crises worldwide. Thus, research into the behaviour of plasmids in antibiotic scenarios is paramount in developing new strategies to combat bacterial infections.
"Understanding and controlling plasmid transfer and antibiotic resistance is crucial for future strategies in public health management and medical treatments."
Contribution to Metabolic Diversity
Another fascinating aspect of plasmids is their role in promoting metabolic diversity among bacteria. Many plasmids harbor genes that enable their bacterial hosts to metabolize a wider range of substrates than they could without the plasmid. This get's particularly interesting in environments like soil and the human gut, where varying nutrient sources exist.
- Degradation of Pollutants: Plasmids can encompass genes that allow bacteria to break down toxic substances, such as heavy metals or hydrocarbons, effectively turning bacteria into bioremediators. This bioremediation potential has led to practical applications involving the cleanup of contaminated environments.
- Synthesis of Nutrients: Some plasmids carry genes responsible for synthesizing vitamins and other essential nutrients, enabling bacteria to survive in nutrient-poor environments while benefiting surrounding microbial communities.
These functions not only illustrate the adaptive capacity of bacteria but also spark interest in harnessing plasmids for biotechnological innovations. By manipulating plasmids, researchers can create microbes that efficiently produce biofuels or other valuable compounds from waste materials.
The exploration into how plasmids augment microbial metabolism and adaptability holds endless possibilities for future research and applications in biotechnology, ultimately contributing to our ability to manage ecological and health-related challenges.
Mechanisms of Plasmid Transfer
Understanding the mechanisms of plasmid transfer is pivotal in the intricate dance of genetics that underlies both natural and engineered scenarios. Bacterial plasmids are not just simple hitchhikers; they serve as vital vehicles for carrying genetic information across different bacterial strains and species. This transfer can significantly influence bacterial evolution, enhance adaptability, and ultimately facilitate gene manipulation in the field of biotechnology. Grasping how plasmids transfer is not merely academic; it holds substantial implications for antibiotic resistance, genetic modification techniques, and advancements in genetic engineering.
Conjugation Processes
Conjugation stands as the primary pathway for plasmid transfer, somewhat akin to a handshake between two bacteria. In this process, a donor bacterium extends a physical connection to a recipient bacterium through a specialized structure called a pilus. This appendage allows for the transfer of plasmid DNA, often accompanied by chromosomal components. The mechanics are not unlike a game of telephone where genetic information is relayed between players.
- Initiation: The donor bacterium recognizes a compatible recipient. This specificity is key and relies heavily on the composition of surface proteins.
- Pilus Formation: The donor extends a pilus to the recipient, establishing a temporary structure that facilitates direct contact.
- DNA Transfer: Once the connection is made, enzymes from the donor prepare the plasmid for transfer. The plasmid often gets nicked, allowing one strand to enter the recipient cell while the complementary strand remains behind.
- Completion: Following the transfer, both bacteria end up with a complete plasmid; the recipient may now express new traits that can include antibiotic resistance or enhanced metabolic capabilities.
This natural process not only enhances genetic variability but also introduces challenges in managing antibiotic resistance in pathogens. The transfer of R plasmids, which carry genes conferring antibiotic resistance, can escalate treatment difficulties across various bacterial populations.
Transformation and Transduction
While conjugation is a prominent method, plasmid transfer can also occur through transformation and transduction, each with its own quirks.
Transformation involves the uptake of naked plasmid DNA from the environment by a competent bacterium. This can happen when bacteria die and release their genetic material—an example of nature’s recycling. Successful transformation hinges on the specific conditions: temperature, competence factors, and the physical state of the DNA. The new bacterial resident may adopt traits embedded in the plasmids it absorbs, leading to unexpected biological properties.
Transduction, on the other hand, is a bit of a sleight of hand. In this process, bacteriophages (viruses that infect bacteria) play the role of genetic messengers. When a bacteriophage infects a bacterial cell, it may inadvertently incorporate a portion of the host’s plasmid DNA into its own genetic material. Subsequent infection of another bacterium by this phage can lead to the transfer of plasmid DNA to the new host. This method underscores how mediators can influence genetic exchange, widening the scope for innovation and adaptation among bacteria.
"The mechanisms of plasmid transfer illuminate the underlying connectivity in bacterial populations, shaping their survival and adaptation strategies."
Applications of Plasmids in Biotechnology
Bacterial plasmids have carved a niche for themselves in the realm of biotechnology, acting as critical players in various applications that stretch the capabilities of modern science. It's hard to underscore the importance of these molecular tools, as they have become integral for researchers who are pushing the boundaries of genetic manipulation and biopharmaceutical development. The advantages that come with the utilization of plasmids are manifold; from streamlining cloning procedures to enhancing the efficacy of gene delivery, they hold immense potential to serve a variety of needs across laboratories worldwide.
Plasmids in Cloning
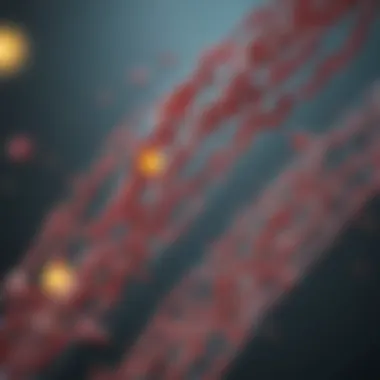
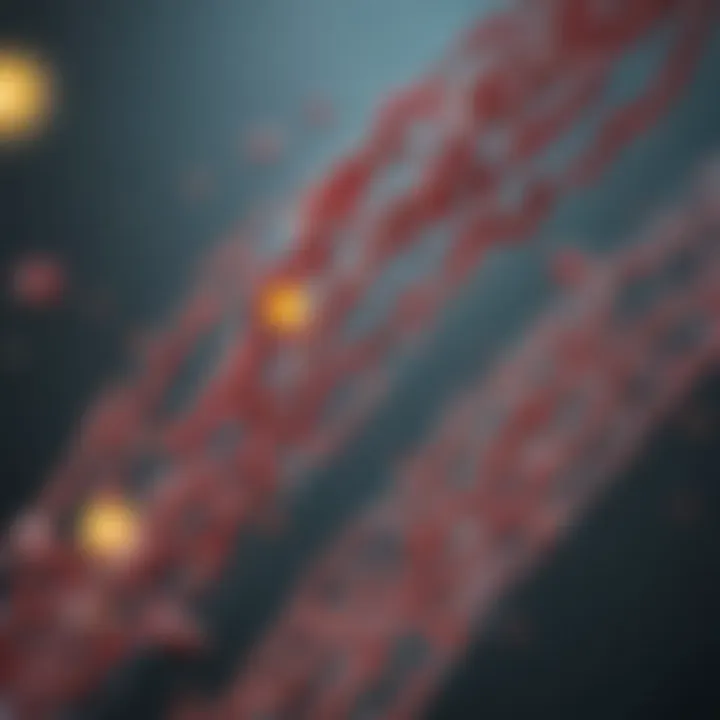
Cloning, at its core, is about copying genetic material, and plasmids are like the Swiss Army knives of this process. They facilitate the insertion of foreign DNA into host organisms, helping in the replication of this inserted material on a grand scale. Using plasmids in cloning simplifies the entire procedure. The first step often involves preparing the plasmid by cutting it with restriction enzymes, allowing the insertion of a specific gene that researchers aim to clone. These vector plasmids, once engineered, can not only carry the desired gene but also contain unique markers that help identify successful clones later on.
Some of the notable features of plasmids that enhance their role in cloning include:
- Origin of replication: This ensures that the plasmid is replicated independently within a bacterial cell.
- Selectable markers: Commonly including antibiotic resistance genes, these allow scientists to distinguish between transformed and non-transformed cells easily.
- Promoter regions: These are critical for driving the expression of genes inserted into the plasmid.
By leveraging the unique features of plasmids, cloning becomes not only feasible but quantitatively efficient, contributing significantly to fields like gene therapy and vaccine development.
Gene Expression Systems
In the landscape of genetic engineering, plasmids serve as vital components for gene expression systems. The ability to use these molecular vectors to drive the expression of particular genes has advanced our understanding of proteins and their functions considerably. In this context, plasmids facilitate the creation of proteins that can be used as research tools, diagnostics, or even therapeutic agents.
An effective gene expression system must ensure that the foreign gene is translated and folded properly. Plasmids, flexibly engineered to include appropriate promoter sequences—such as the T7 or lac promoter—enable high levels of gene expression in host organisms. The adoption of plasmids in gene expression comes with its own set of parameters that researchers must consider:
- Host strain compatibility: Different bacterial strains have different efficiencies for expressing proteins from plasmids.
- Post-translational modifications: Eukaryotic proteins often require certain modifications that bacteria may not provide.
- Protein solubility: Some proteins may misfold in host cells, necessitating careful design of the plasmid to assist in proper folding.
Collectively, these considerations illustrate how plasmids aid in not just expressing but also purifying proteins, a necessity for applications in drug development and therapeutic interventions.
Gene Delivery Mechanisms
Understanding how to deliver genetic material into a target cell is crucial in the world of genetic engineering, and here, plasmids shine again. They serve as efficient delivery vehicles for genes that may encode therapeutic proteins or for applications in gene therapy, where introducing new or repaired genes into patients could be a game changer. The delivery methods can vary based on the goal, and plasmids can be tailored to fit these roles effectively.
Various techniques for using plasmids in gene delivery include:
- Electroporation: In this method, an electrical field increases cell membrane permeability, allowing plasmids to slip inside.
- Microinjection: Here, a fine needle injects the plasmid directly into the target cell, offering precision.
- Viral vectors: In some instances, plasmids can be modified to create viral-like particles to enhance the efficacy of transfer.
These gene delivery strategies highlight the versatility of plasmids in medical applications, not only enhancing safety compared to viral approaches but also offering avenues for personalized medicine initiatives.
By understanding and optimizing the roles of plasmids in cloning, expression systems, and gene delivery, we position ourselves closer to revolutionary breakthroughs in biotechnology.
In summary, the applications of plasmids in biotechnology are undeniably expansive and continue to shape the landscape of genetic research and therapeutic development.
Plasmids and Genetic Engineering
Bacterial plasmids play a crucial role in the field of genetic engineering. Their significance lies in their ability to carry genetic material that can be manipulated and introduced into different organisms. This adaptability has made plasmids an invaluable tool in synthetic biology and biotechnology. Their usage has reshaped the landscape of genetic research and paved new paths for therapeutic interventions. Through plasmids, scientists are not just thinking outside the box; they're redesigning it.
One of the most notable benefits of employing plasmids in genetic engineering is the ease with which they can be modified. Unlike traditional chromosomal DNA, plasmids allow for quicker genetic alterations which can accelerate the development of new genetic tools. This ability to facilitate rapid changes is vital when considering the pace of scientific advancements nowadays.
In addition, plasmids can sustain different genes simultaneously, offering researchers a unique platform for multi-gene engineering. This feature can yield organisms with more complex traits that would be unattainable through conventional breeding techniques. Think of the potential for crops that can resist multiple pests or withstand drastic weather conditions. The possibilities are practically limitless.
Another critical aspect is how plasmids contribute to the efficiency of gene delivery systems. They serve as vectors in the insertion of genes into target cells, which is often the first step in gene therapy and other applications. For example, the use of plasmid vectors in vaccines, where they can help to elicit an immune response against diseases, illustrates this point well. The incorporation of plasmids into vaccination strategies is actively being tested, and it brings hope for controlling various pathogens.
However, the journey is not without its bumps. The manipulation of plasmids for these innovative purposes must be approached with caution. Ethical considerations abound, and the complexities of plasmid behavior within host organisms can lead to unpredictable consequences. The dynamics between engineered plasmids and natural systems are still a work in progress and require careful examination.
"The power of plasmids lies not just in their genetic material, but in the challenges they present for responsible use and management."
As we explore further into the specifics of plasmid dynamics and the hurdles associated with their engineering, it’s crucial to maintain a balanced view. Plasmids represent both the promise of groundbreaking treatments and the potential for unforeseen alterations in ecosystems and human health. Discerning the line between innovation and ethical responsibility is key to harnessing the true power of bacterial plasmids in genetic engineering.
CRISPR and Plasmid Dynamics
The advent of CRISPR technology has revamped the ways researchers utilize plasmids in genetic engineering. CRISPR, standing for Clustered Regularly Interspaced Short Palindromic Repeats, enables precise alterations in DNA sequences. Plasmids serve as carriers of CRISPR components, facilitating the delivery of gene-editing tools into target cells. This synergy between plasmids and CRISPR has made it possible to target specific genes with unprecedented accuracy.
In practice, plasmids can be engineered to carry not just the genes of interest but also the CRISPR-associated protein Cas9, along with guide RNA sequences. The plasmid constructs can then be transfected into various cell types, directing the Cas9 to the specific DNA locations that need alteration. This process is increasingly being utilized in therapeutic contexts, such as cancer treatment, where precise gene modification can correct mutations that drive the disease.
Yet, the introduction of CRISPR technology into bacterial plasmid dynamics doesn’t come without challenges. The efficiency and stability of plasmid uptake can vary significantly among different bacterial strains. Moreover, the potential for off-target effects—where CRISPR inadvertently alters unintended regions of the genome—remains a valid concern that scientists are only beginning to understand and mitigate.
Challenges in Plasmid Engineering
Despite all the advantages, plasmid engineering faces several challenges that can hinder its efficacy in genetic engineering. The first notable issue is their instability in host organisms. Plasmids can sometimes lose their ability to replicate or may even be eliminated from bacteria over time. This phenomenon can be notably problematic for long-term experiments or therapeutic applications that rely on stable gene expression.
Further complicating matters is the variability of the host environment. Different bacterial species have unique mechanisms that may affect how they accommodate and express plasmid DNA. This variability means that what works well in one type of bacteria may fail in another. Researchers often find themselves navigating uncharted waters, requiring pilots with a steady hand and keen observation.
Moreover, regulatory hurdles often stymie the pace of innovation. Plasmid-based therapies are subject to scrutiny, and ensuring compliance with safety and efficacy standards can be a lengthy process. Striking a balance between rapid advancements in genetic engineering and the necessary regulatory frameworks can seem like trying to tame wild horses.
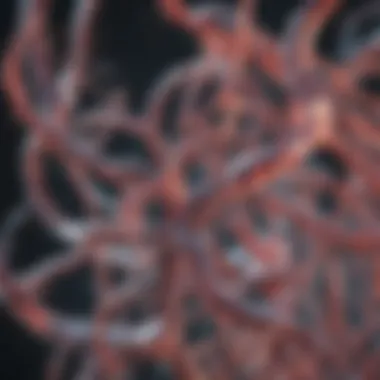
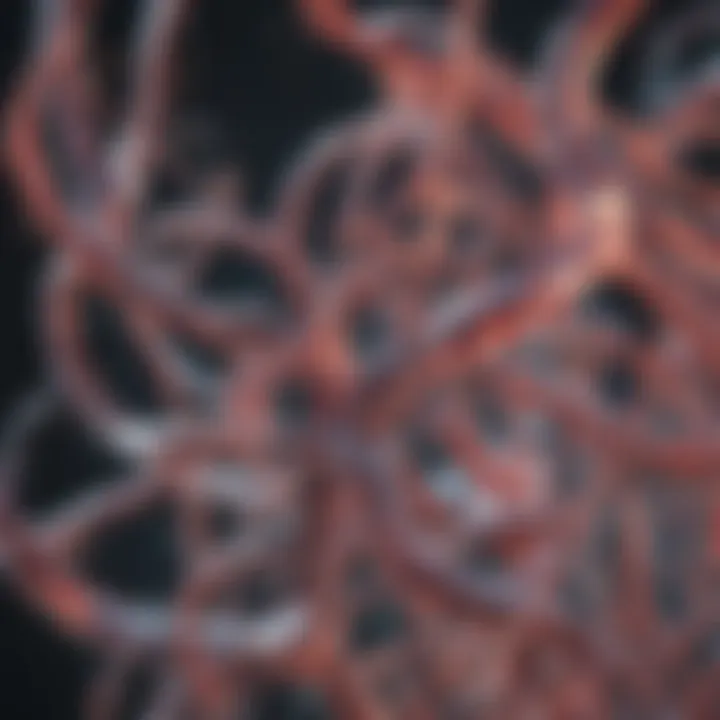
As we look to the future, it is clear that overcoming these challenges is vital to unlock the full potential of bacterial plasmids. The ongoing research will not only enhance our understanding but will also guide sustainable practices. The road may be rocky, but the promise that plasmids hold in genetic engineering remains a beacon for future innovation.
Ethical Considerations and Risks
The exploration of bacterial plasmids within genetic engineering not only heralds remarkable advancements in science, but it also brings along a considerable ethical baggage. As we navigate through the waters of biotechnology, understanding the implications of harnessing these biological agents is crucial. The dialogue around bioethics is increasingly relevant, as researchers, educators, and policymakers must grapple with the complexities arising from manipulations at the molecular level. Ethical considerations serve as guiding principles when engaging with plasmid technology, keeping both progress and responsibility in balance.
Biosecurity Risks
When it comes to genetic engineering, biosecurity cannot be an afterthought. As plasmids can confer advantageous traits, such as antibiotic resistance, they can become a double-edged sword. If these plasmids are introduced to wild populations of bacteria, the consequences could be catastrophic. One might say it's like handing a loaded weapon to someone without proper training; the risks involved require serious contemplation.
Key points include:
- Uncontrolled Spread: The potential for engineered plasmids to escape laboratory settings can lead to wild strains that possess unforeseen capabilities.
- Dual-Use Dilemma: What is beneficial research today could morph into a troubling threat tomorrow, particularly if such knowledge is weaponized.
- Regulatory Frameworks: Strong policies and safeguards are paramount to ensure that plasmid manipulation doesn't spiral out of control.
"The biosecurity risks associated with plasmid technology require attention and diligence from every stakeholder involved."
Taking proactive measures, like establishing stringent containment protocols, is essential to mitigate risks. It’s not merely about preventing accidents, but also about upholding public trust in biotechnological advancements.
Environmental Impact
As we shift gears to the environmental impact, the consequences of releasing engineered plasmids into natural ecosystems can't be brushed aside. The interconnectivity of biological systems is such that an alteration in one component—like a plasmid—can resonate throughout the ecosystem. Consider, for instance, the implications of bacteria equipped with plasmids designed for better decomposition. While it might seem beneficial, introducing such organisms could disrupt existing ecological balances.
Critical factors to evaluate include:
- Loss of Biodiversity: Introducing modified bacteria might give rise to competitive advantages that could outcompete native species, leading to a reduction in biodiversity.
- Unintended Consequences: Altered metabolic pathways can generate by-products that might be toxic or disruptive to the surrounding organisms that rely on the pre-modification state.
- Ecosystem Resilience: Healthy ecosystems have built-in resilience; any interference can compromise their ability to adapt to changes or recover from disturbances.
In essence, addressing the ethical dimensions of plasmid use offers the potential to construct a more secure and sustainable path forward. Researchers and practitioners in the field of genetic engineering must thread carefully, balancing innovation against the backdrop of ethical responsibility, considering not just what we can do, but what we should do.
Future Prospects of Bacterial Plasmids
The conversation surrounding bacterial plasmids is not just a historical or theoretical exposition; it is an ongoing exploration into the future of genetic engineering. The increasing understanding of plasmids opens avenues for innovations that could potentially revolutionize aspects of biotechnology, medicine, and environmental science. As we delve into this realm, it's crucial to spotlight specific benefits, considerations, and emerging ideas that make the future of plasmid research so promising.
Innovations in Research
Understanding how to manipulate plasmids has led to remarkable strides in scientific research. One area seeing exciting developments is synthetic biology, where researchers engineer new plasmids tailored for specific applications. These newly crafted plasmids can carry gene-editing tools to create organisms that can produce pharmaceuticals or biofuels more efficiently.
- CRISPR Technology: One of the best examples of innovation is the application of CRISPR in conjunction with plasmids. By leveraging plasmids as vectors for distributing CRISPR elements, scientists can target specific genes in various organisms, paving the way for precise modifications. This combination allows quicker advancements such as gene therapy, crop improvements, and even microbial remediation.
- Plasmids in Vaccinology: The potential of plasmids in vaccine development cannot be understated. They offer a method to deliver DNA vaccines, which have shown promise in stimulating a robust immune response. The rapid adaptation of this technology is particularly crucial in response to emerging infectious diseases.
In essence, innovative research is contributing to new frameworks for understanding genetic functions and interactions that could yield life-saving solutions.
Potential for New Therapeutics
The therapeutic prospects that stem from the manipulation of bacterial plasmids are multifaceted. They are not merely tools for genetic engineering; they might become cornerstones of modern medicine.
- Targeted Gene Therapy: Research is ongoing into using plasmids for targeted gene therapy in treating genetic disorders. By designing plasmids that carry corrective genes, the hope is to remedy conditions caused by gene mutations. This approach has gained traction due to the successes observed in clinical trials for diseases like cystic fibrosis and muscular dystrophy.
- Antibiotic Alternatives: As antibiotic resistance burgeons, plasmids might provide alternative pathways for treatment. Investigations are underway to harness plasmids to transfer genes that can either enhance the host bacteria’s ability to resist pathogens or modify them so they don’t produce harmful effects on humans. This unexplored avenue could redefine how we address bacterial infections.
- Bioremediation Strategies: Utilizing plasmids to implant specific metabolic pathways into microbes poses a significant opportunity for environmental recovery. Engineers can design plasmids carrying genes that allow microorganisms to break down pollutants in contaminated environments effectively. This bioengineering approach is both sustainable and eco-friendly.
In summary, the intersection of plasmid technology with innovative practices signifies a critical juncture in genetic engineering. Leveraging plasmids facilitates advancements that hold promise for both academic research and practical applications.
As we look forward, the potential for bacterial plasmids to contribute to new therapeutics underscores their significance in addressing current health challenges and environmental issues. Embracing these opportunities will require strides in regulatory frameworks and ethical guidelines to ensure safe progress. Overall, the horizon is bright for research into bacterial plasmids, which are poised to remain at the forefront of genetic engineering and biotechnology.
Ending
Key elements regarding the significance of plasmids include their ability to replicate independently, which allows them to carry essential genes that can be transferred between different bacterial strains. Recognizing how these elements function in genetics opens doors for innovations in medical treatments, agriculture, and environmental management. Specifically, plasmids provide a means for researchers to introduce desirable traits into organisms, thus improving crop resistance, augmenting vaccine development, and possibly creating pathways for novel therapies against various diseases.
Furthermore, the practical applications of plasmids in gene cloning and expression systems enable scientists to produce proteins that can lead to breakthroughs in disease treatment. This capacity for manipulation underscores the need for continuous research, particularly in understanding the complexities and nuances of plasmid behavior in various contexts. As biotechnological techniques advance, the role of plasmids will likely expand, resulting in both opportunities and ethical challenges that must be carefully navigated.
"Bacterial plasmids serve as vital tools not just in the lab but in reshaping our understanding of genetics and its applications in everyday life."
Summary of Key Points
- Independent Replication: Plasmids are small, circular DNA molecules that can replicate independently from chromosomal DNA.
- Gene Transfer: They play a crucial role in transferring genes between bacteria, enhancing genetic diversity and adaptability.
- Biotechnological Applications: Used extensively in gene cloning, expression systems, and gene delivery mechanisms for various applications in medicine, agriculture, and research.
- Antibiotic Resistance: Many plasmids carry genes that confer resistance, complicating treatment protocols and highlighting their impact on public health.
- Ethical Considerations: The manipulation of plasmids raises important ethical considerations that must be addressed as technology evolves.
Final Thoughts on Future Research Directions
The landscape of genetic engineering is continually evolving, and the future prospects surrounding bacterial plasmids suggest exciting opportunities. Researchers are likely to delve deeper into the mechanisms through which plasmids govern gene expression and interaction across different environments. Improved understanding may catalyze the development of more precise gene editing techniques and enhancement strategies.
Potential avenues for future research may include:
- Enhanced Gene Delivery Systems: Investigating novel plasmid-based technologies that can increase the effectiveness of gene therapies.
- Synthetic Biology Applications: Creating custom plasmids tailored to specific tasks in synthetic biology could revolutionize how we approach ecosystem management and medicine.
- Combatting Resistance: Development of methods to counteract plasmid-mediated resistance in harmful bacteria is crucial for public health.
By exploring these directions, researchers have the chance to not only refine existing technologies but also design innovative solutions that address some of today’s pressing biological challenges.