Exploring Yeast Enzymes in Biochemical Processes
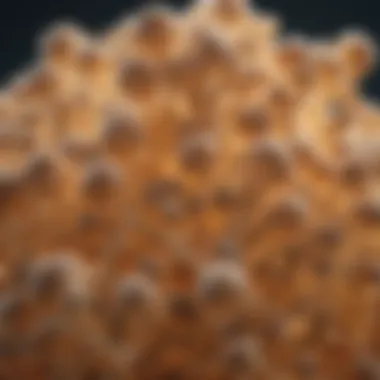
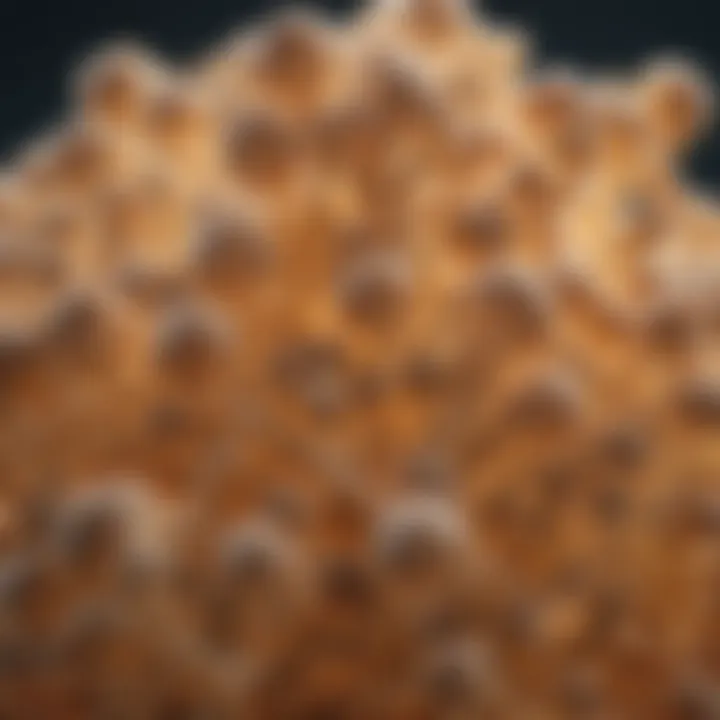
Intro
Yeast enzymes, often overlooked in the grand tapestry of biochemistry, play a pivotal role in numerous biochemical processes. They act as catalysts, speeding up reactions and enabling the myriad of transformations that sustain life—both in nature and in industrial applications. With the ability to influence fermentation, biosynthesis, and even waste treatment, these enzymes deserve a spotlight.
The Importance of Yeast Enzymes
In everyday terms, yeast enzymes are like the unsung heroes of the fermentation process. Take baking, for instance. When you mix dough, it’s not just a simple combination of flour and water. The yeast adds a rich layer of complexity through fermentation. This process breaks down sugars, producing carbon dioxide and ethanol, which give rise to that delightful fluffiness in bread. Without yeast enzymes, bakers would face challenges reminiscent of trying to climb a mountain without any gear.
In addition to their role in baking, yeast enzymes have made significant strides in the fields of biotechnology and pharmaceuticals. The ability to synthesize complex molecules has revolutionized how we approach drug development and biofuel production. Understanding these enzymes can lead to innovations that drive industries forward.
This article will navigate through the structure, function, and applications of yeast enzymes. It aims to provide a comprehensive outlook not just on their current significance but also on what the research community is shedding light on for the future.
"Yeast enzymes are more than catalysts; they're the heartbeat of many biochemical processes that define industries and scientific research."
As we embark on this journey, we’ll explore methodologies used in studying yeast enzymes, discuss their practical implications, and compare contemporary findings with previous research. Let's dig deeper into the world of yeast enzymes, where each tiny molecule can make a monumental difference.
Preface to Yeast Enzymes
Understanding yeast enzymes is key in the world of biochemistry. These enzymes don’t just play roles in making bread rise or fermenting beverages; they are catalysts for a slew of biochemical reactions that underpin many processes in both nature and industry. Their ability to facilitate reactions makes them invaluable in various applications—both practical and theoretical.
Yeast enzymes have a rich and varied history, linking ancient practices with the modern scientific approach. Their story takes us from traditional brewing methods to cutting-edge biotechnological advancements. This understanding bridges the gap between historical practices and modern applications.
Now let’s get into the nitty-gritty. They function by accelerating biochemical reactions at a cellular level. This means they are crucial in processes such as fermentation, where sugars are converted into alcohol and carbon dioxide. Beyond fermentation, yeast enzymes engage in metabolic pathways that help to break down complex molecules, thus making nutrients more accessible.
Historical Background
The early uses of yeast can date back thousands of years. Ancient civilizations like the Egyptians were already leveraging these microorganisms to brew beer and make leavened bread. However, the detailed understanding of their enzymatic functions didn’t emerge until much later. It wasn't until the 19th century, with the advent of microbiology, that scientists like Louis Pasteur began exploring yeast in depth.
The term "enzyme" was introduced later on, referring to the proteins that act as catalysts in biological reactions. Even then, knowledge surrounding yeast enzymes was scant. It took years of diligent research to unravel the complex roles they played beyond mere fermentation.
Fundamental Concepts
At its core, the function of yeast enzymes rests on a few solid principles. The most basic of these is the idea of specificity. Different enzymes work on specific substrates—molecules that enzymes react with. For example, amylases target carbohydrates, while proteases focus on proteins. This specific targeting ensures efficiency in biochemical processes.
Understanding enzyme kinetics—the study of the rates of enzyme-driven reactions—is also critical here. Factors such as temperature, pH, and substrate concentration can significantly influence enzyme activity.
In addition, the concept of enzyme regulation plays a major role. Enzymes can be activated or inhibited by various factors, including other molecules present in the solution. This dynamic regulation means that cells can finely tune their biochemical processes based on current needs—like a conductor guiding an orchestra.
To sum it up, yeast enzymes are multi-faceted tools in biochemical processes. They have been integral to human food production for eons, and their functions extend into countless applications in industry and research. Their ability to catalyze reactions forms the bedrock of fermentation and numerous industrial processes today.
Types of Yeast Enzymes
Yeast enzymes are the unsung heroes of various biochemical processes. They play critical roles in breaking down complex molecules and transforming them into simpler compounds that our cells can utilize. Understanding the different types of yeast enzymes is essential, as each type has unique functions, benefits, and applications.
Proteases
Proteases are enzymes that break down proteins into smaller peptides or amino acids. They are crucial in various biological processes, including digestion and cell regulation. In brewing, for instance, proteases affect the clarity and stability of beer. When yeast ferments sugars, it also produces proteases that help in breaking down the proteins in malt. This activity is important because it influences the taste and mouthfeel of the final product. Protease activity can also enhance the nutritional value of food by freeing amino acids, making them more accessible for absorption.
"Proteases are not just for digestion; they touch every corner of our metabolism, helping us harness the proteins we consume."
Amylases
Amylases are another vital group of yeast enzymes, primarily responsible for breaking down starches into sugars. There are two main types: alpha-amylase and beta-amylase. Alpha-amylase cuts the starch chains into shorter segments, while beta-amylase works on those smaller chains to produce maltose. In the world of baking and brewing, amylases play an instrumental role. They ensure that the yeast has a steady supply of fermentable sugars to work with, which is crucial for effective fermentation. Without amylases, the fermentation process would stall, resulting in less alcohol production.
Lipases
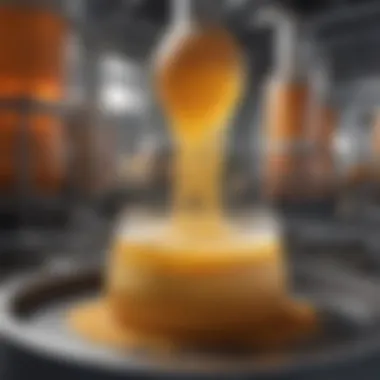

Lipases are enzymes that catalyze the breakdown of fats into fatty acids and glycerol. In yeast, lipases contribute to lipid metabolism, facilitating energy production. They are particularly important for the fermentation process in the production of certain alcoholic beverages, as they can break down the fats present in yeast and other components of the fermentation mixture. This action can enhance the flavor profile of products, leading to a richer and more complex final product. Additionally, lipases serve a protective role, helping to maintain the integrity of cell membranes in yeast cells during stressful conditions.
Cellulases
Cellulases are enzymes that break down cellulose, a major component of plant cell walls. While yeast grows predominantly on sugars extracted from starches or simple sugars, cellulases may come into play when yeast is exposed to complex carbohydrate sources. In such cases, cellulases allow yeast to access these carbohydrates, expanding their nutrient sources beyond simple sugars. This enzymatic action has implications for biofuel production, where yeast can ferment plant materials that would otherwise be difficult to digest. By breaking down cellulose, yeast becomes more versatile in its carbohydrate metabolism, allowing for a broader range of substrates for fermentation.
Biochemical Mechanisms
Understanding the biochemical mechanisms surrounding yeast enzymes is vital for grasping their multifaceted roles in various biological processes. At the core of this understanding lies enzyme activity regulation and substrate specificity. Both aspects are intertwined, influencing how enzymes interact with their surroundings and contribute to the organism's biochemical pathways.
Enzyme Activity Regulation
Regulating enzyme activity is not merely a cellular whim; it’s a fundamental aspect of metabolism, ensuring that yeast can adapt to environmental changes. Enzymes function much like trusty compasses, guiding the biochemical reactions that fuel cellular processes. There are several mechanisms at play in this regulatory scheme:
- Allosteric Regulation: This involves the binding of molecules at sites other than the active site, altering the enzyme's shape and, consequently, its activity. Think of it as adjusting the volume on a radio; small tweaks can lead to significant changes in performance.
- Feedback Inhibition: It’s a classic case of a system keeping itself in check. When the product concentration rises to a certain point, the pathway is turned off to prevent overproduction. This is akin to a thermostat controlling room temperature, ensuring homeostasis is maintained.
- Covalent Modification: Enzymes can be activated or deactivated through the addition or removal of certain chemical groups. Phosphorylation, for instance, can serve to "switch on" enzymes, playing a crucial role in metabolism.
These mechanisms collectively ensure that yeast enzymes operate efficiently under various conditions, optimizing energy consumption or metabolic output as needed. Consequently, understanding these regulations can provide insights into how yeast can thrive in fluctuating environments, making them suitable for industrial applications.
Substrate Specificity
Substrate specificity is the hallmark of enzyme functionality. Enzymes do not play the field, so to speak; they are picky about which substrates they will act upon. This specificity is essentially the lock-and-key model, where only the correct key (substrate) can unlock the door (enzyme). Factors influencing this specificity include:
- Active Site Configuration: The shape and chemical environment of an enzyme's active site directly determine which substrates can bind. A mismatched shape can lead to non-productive binding, which, you could say, is like trying to fit a square peg in a round hole.
- Chemical Properties of Substrates: Different substrates possess unique chemical characteristics that enzymes recognize. Hydrophobicity, charge, and functional groups all play a role in the interaction.
- Environmental Conditions: pH levels and temperature can also affect the binding. An ideal environment may enhance the affinity between an enzyme and its substrate, while extreme conditions may distort the enzyme structure, hindering specificity.
In sum, the subtle dance between enzyme and substrate not only fuels yeast metabolism but also underscores the precision required for biochemical reactions to proceed efficiently. This precision is crucial for various applications, whether in fermentation processes or broader biotechnological innovations.
"A thorough understanding of these biochemical mechanisms lays the groundwork for advancements in fields relying on yeast enzymes, influencing everything from food production to pharmaceuticals."
By delving into enzyme activity regulation and substrate specificity, we unravel the complexities behind yeast enzymes, opening gateways to greater innovations and applications in science and industry.
Yeast Enzymes in Fermentation
Yeast enzymes play a pivotal role in fermentation, a process that encompasses not only the delightful transformation of raw ingredients into delectable products but also underlines many biochemical pathways essential for industrial applications. These enzymes drive metabolic reactions, restoring energy and generating substances that are crucial for producing alcohol, carbon dioxide, and various flavor compounds. Their functions enable the conversion of sugars from raw materials into ethanol and carbon dioxide, which form the backbone of many fermentation processes.
The significance of yeast enzymes in fermentation can be seen in their ability to catalyze reactions under conditions that might be unfavorable for other types of enzymes. For instance, certain yeast strains can thrive in high-sugar and low-oxygen environments, employing enzymes such as zymase to enhance fermentation efficiency. This capability not only boosts the yields of alcoholic beverages but also contributes to the unique flavor profiles found in different products, enabling consistency and quality across batches.
Role in Alcoholic Fermentation
Alcoholic fermentation is primarily facilitated by yeast species, most notably Saccharomyces cerevisiae. The core process involves the breakdown of glucose into ethanol and carbon dioxide, helping to produce various alcoholic drinks.
The fermentation begins with the yeast recognizing and consuming the available sugars. Here, enzymes like hexokinase and phosphofructokinase come into play, initiating glycolysis.
- Glycolysis: In this step, enzymes convert glucose into pyruvate.
- Decarboxylation: The pyruvate is then decarboxylated, producing acetaldehyde and releasing carbon dioxide.
- Alcoholic fermentation: Ultimately, acetaldehyde is converted into ethanol through the action of alcohol dehydrogenase.
This enzymatic pathway not only emphasizes the role of yeast as a vital agent in fermentation but also exemplifies how different enzymes integrate to make the process vibrant and efficient. The generation of carbon dioxide is particularly important for baking, as it leads to the leavening of dough, showcasing the crossover between brewing and baking applications.
Applications in Brewing and Baking
In the brewing industry, yeast enzymes are indispensable for producing beer, cider, and other fermented beverages. The variety of yeast strains used, each with distinct enzymatic profiles, leads to a diversity of flavors and textures in the finished product. For instance, lager yeast might impart a clean taste, while ale yeast can add fruity notes, all due to the differing enzymes engaging in metabolic activities.
Similarly, in baking, yeast creates bubbles of carbon dioxide through fermentation, yielding a light and airy texture in bread and pastries. This dual functionality makes the enzymes essential not just for flavor and alcohol production but also for the quality of baked goods.
Furthermore, both brewing and baking benefit from the manipulation of fermentation conditions—temperature, pH, and nutrient availability—to optimize the activity of yeast enzymes, tailoring them for specific flavor profiles or product qualities.
"The intricate dance of enzymes during fermentation is where the magic of flavor and texture is born, illustrating the profound connection between science and culinary arts."
In summary, the role of yeast enzymes in fermentation is unmissable; they are the unsung heroes that catalyze biochemical reactions, leading to the creation of beverages and baked goods that are truly loved across cultures. The more we understand these processes, the better we can leverage their potential for innovation across various industries.
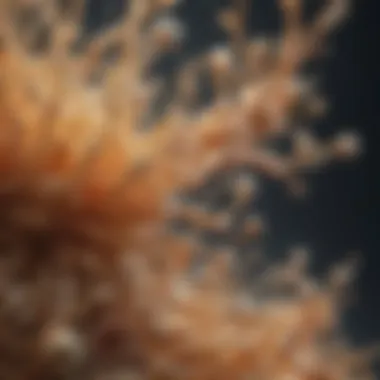
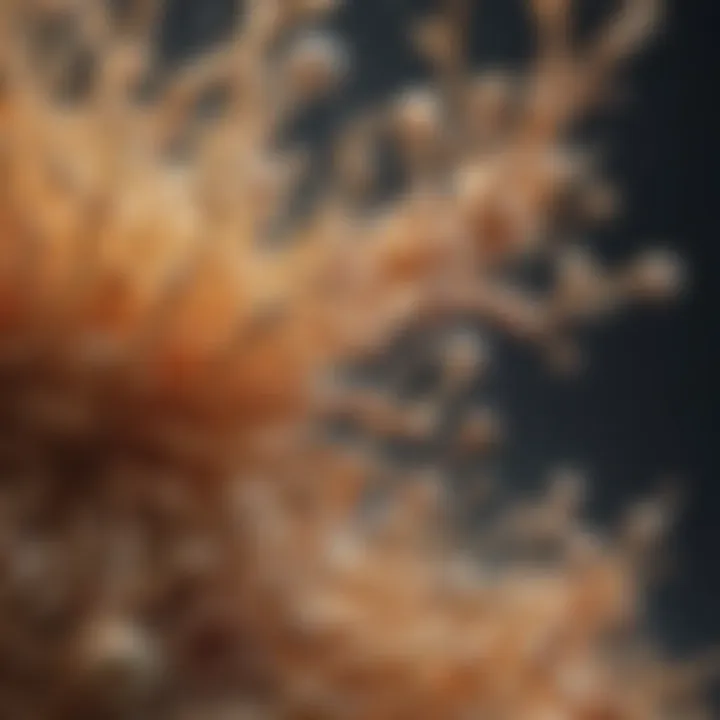
Industrial Applications of Yeast Enzymes
The industrial applications of yeast enzymes represent a cornerstone where biochemistry intersects with varied sectors. These enzymes, hailed for their versatility, facilitate numerous processes, enhancing efficiency while reducing costs. In this section, we shall unveil the nuances of how these enzymes play pivotal roles in biotechnology and pharmaceuticals. Their capabilities stretch across fermentation to biosynthesis, making them indispensable assets in contemporary industrial practices.
Biotechnology Innovations
Biotechnology has taken giant leaps forward, owing largely to the infusion of yeast enzymes. A notable area of impact lies in the development of biofuels. Here, cellulases derived from yeast are instrumental. By breaking down cellulose in plant biomass, these enzymes help in converting agricultural waste into fermentable sugars. This not only offers a sustainable energy source but also addresses waste management challenges.
Moreover, enzymes such as proteases and amylases are paving the way for food biotechnology. They enhance flavor profiles and improve texture in food products. For instance, the application of amylases in brewing transforms starches into sugars, enabling a more robust fermentation process. This results in higher alcohol content and improved taste in beers.
In addition, yeast enzymes contribute significantly to the production of various bioproducts, including organic acids and amino acids. In the context of food preservation, certain enzymes assist in inhibiting spoilage, thus extending shelf life. These biotechnological innovations herald a new era of efficiency and sustainability in multiple industries.
Pharmaceutical Uses
The pharmaceutical industry has also felt the positive ripple effects of yeast enzyme research. Enzymes are employed in drug formulations, ensuring a more effective delivery mechanism of active ingredients. For instance, invertase, an enzyme extracted from yeast, is used in the creation of syrupy forms of medications. This enhances the bioavailability of compounds, allowing for better absorption in the body.
Furthermore, yeast enzymes play a part in biosensing applications. They assist in the development of diagnostic tools that rely on enzyme reactions to detect specific biomolecules. This capability is crucial for developing rapid tests for various illnesses, proving essential in health monitoring.
There’s also an ongoing exploration into the unique properties of yeast-derived enzymes for producing therapeutic compounds. Research is focusing on their potential in synthesizing drug intermediates, which can reduce the steps involved in complex chemical synthesis.
In summary, the uses of yeast enzymes in biotechnology and pharmaceuticals underscore their importance. As industries move toward more efficient, sustainable practices, these natural catalysts will undoubtedly remain at the forefront, driving innovation and expansion across numerous fields.
Role of Yeast Enzymes in Nutritional Biochemistry
Yeast enzymes play a significant role in nutritional biochemistry by influencing the availability of nutrients in food. They are not merely biochemical tools, but rather essential agents that interact with a wide variety of substrates. This section aims to highlight their importance in nutrient availability and bioavailability, two critical factors that impact overall health.
Impact on Nutrient Availability
Yeast enzymes contribute directly to the availability of nutrients during various biochemical processes. For instance, enzymes like amylases break down starch into simpler sugars. This breakdown makes carbohydrates more accessible for digestion.
When yeast engages in fermentation, it not only produces alcohol but also enhances the breakdown of complex molecules. By transforming these larger compounds into smaller, more digestible units, yeast can play a pivotal role in the nutritional profile of food products. For example, in the production of sourdough bread, certain yeast strains help degrade complex carbohydrates, making the bread itself easier to digest. Nutrient absorption can be compromised when substrates remain too complex, leading to lower health benefits.
Furthermore, yeast can work alongside other microorganisms to facilitate nutrient transfer. This symbiotic behavior enhances the nutritional quality of foods, especially in fermentation processes applied to dairy and plant-based products.
"The presence of yeast enzymes can take a meal from merely edible to richly nourishing."
Enhancing Bioavailability
Bioavailability, the term used to describe how well nutrients are absorbed and utilized by our bodies, can be significantly influenced by yeast enzymes. For instance, when yeast ferments foods, it can increase the solubility of minerals and vitamins, effectively enhancing their absorption.
Yeast enzymes can also help in releasing bound nutrients. Some vitamins, like B vitamins, are often integrated into complex matrices that make them challenging for our bodies to utilize. Through fermentation, yeast can hydrolyze these compounds, releasing the nutrients for better absorption.
Key points regarding the enhancement of bioavailability through yeast include:
- Vitamin Production: Certain yeasts produce vitamins as a metabolic byproduct, enriching the food rapidly.
- Mineral Solubilization: Enzymatic activity can free up minerals that are otherwise locked within food structures, leading to greater nutritional benefits.
- Protein Digestibility: Yeast proteases lay the groundwork for breaking down proteins into amino acids, improving overall nitrogen balance in food.
By recognizing the synergy between yeast enzymes and nutritional biochemistry, one can appreciate not just their functional roles, but their broader implications for health and wellness. Understanding these functions opens doors to innovative approaches in food preparation and dietary supplementation.
Research Trends in Yeast Enzyme Study
The study of yeast enzymes is more than an academic exercise; it serves as a catalyst for innovation across multiple scientific fields. As society looks toward sustainable methods of production and utilization of resources, the focus on yeast enzymes has never been more salient. These enzymes not only assist in fermentation processes but also contribute extensively to the biotechnology sector, enhancing both industrial and medicinal applications.
Advanced Techniques in Enzyme Analysis
With technological advancements, the analysis of yeast enzymes has undergone a sea change. Methods like high-throughput screening, enzyme-linked immunosorbent assays (ELISA), and real-time PCR are now staples in labs aiming to dissect enzyme action at a micro-level. These techniques enable researchers to scrutinize enzyme activity, stability, and interaction with substrates under varying conditions. Through such detailed analysis, fresh insights into enzyme kinetics and structural biology emerge.
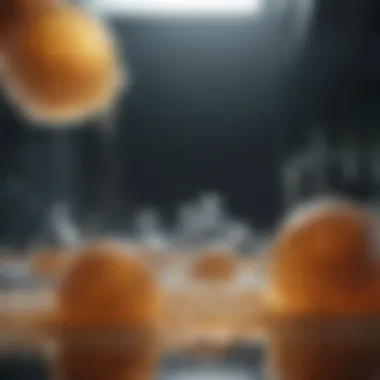
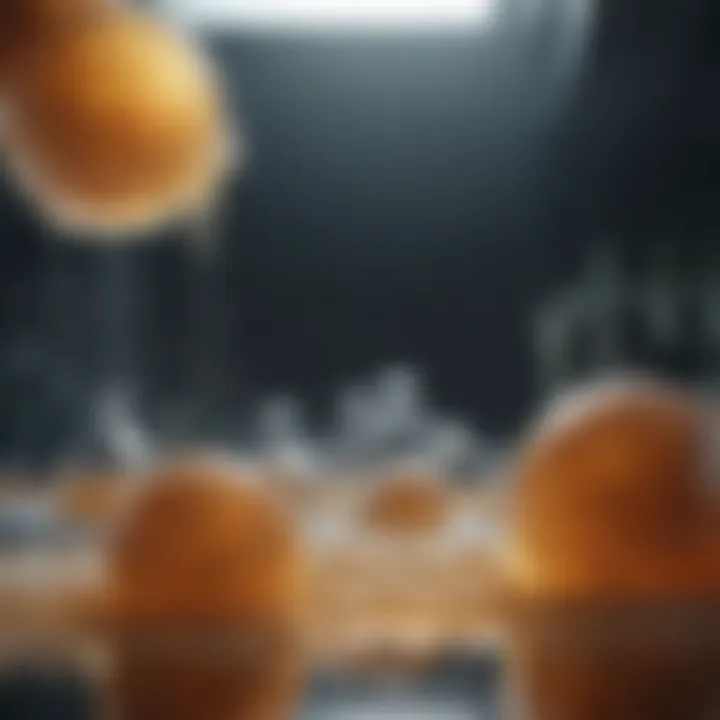
Moreover, proteomics and metabolomics provide complementary perspectives that bring forth a wider understanding of how yeast enzymes operate in context. By analyzing the protein expressions and metabolic pathways, we can see how enzymes adapt and function in diverse environments. The rise of bioinformatics tools to model enzymatic reactions further supports this research, allowing for predictions about enzyme behavior that weren't possible before.
Future Directions in Research
Looking to the future, a number of promising avenues beckon for exploration. First, advancements in synthetic biology may lead to the creation of engineered yeast strains with enhanced enzymatic capabilities. Imagine specialist yeasts that can remodel their enzymatic profiles in response to environmental changes, optimizing the conversion of raw materials into valuable products, such as biofuels and pharmaceuticals.
Additionally, focusing on the environmental impact of yeast enzymes could reveal new applications in bioremediation, where enzymes break down pollutants in soil and water. This leads to the enticing research question: can yeast enzymes be harnessed to clean up oil spills? Such inquiries will remain pivotal in addressing global challenges.
Furthermore, the integration of machine learning into enzyme research holds the potential to unveil hidden patterns in enzyme behavior. As data science and enzyme biology converge, we may find ourselves on the brink of breakthroughs that could redefine how we understand metabolic processes.
"The interplay between yeast enzymes and synthetic biology will likely illuminate pathways that are currently shadowed, urging a re-evaluation of their applications across industries."
In summary, the exploration of yeast enzymes remains not just a quest for biochemical understanding but a journey towards sustainable solutions. Endlessly rich in potential, the trends observed in current studies suggest that the next decade could witness significant breakthroughs, molding a new era in biotechnology.
Challenges and Limitations
When discussing yeast enzymes, it's crucial to recognize the challenges and limitations that permeate their application and functionality in various biochemical processes. Although yeast enzymes offer great potential, understanding these hurdles is essential for advancing both research and practical applications. Here, we break down two prominent concerns: enzyme stability and regulatory considerations, which shape the landscape for future innovations.
Enzyme Stability Issues
Stability of enzymes is a fundamental factor influencing their effectiveness in biochemical reactions. Yeast enzymes, like any biological elements, are susceptible to denaturation under adverse conditions, such as extreme temperatures and pH levels. The integrity of their three-dimensional structures can be compromised, impacting their catalytic abilities.
Enzyme stability can be affected by several variables:
- Temperature Fluctuations: Higher temperatures typically increase reaction rates, but they also risk denaturing enzymes. Striking a delicate balance is crucial for maintaining enzyme activity.
- pH Levels: Enzymes operate optimally within specific pH ranges. Deviating from these thresholds often leads to diminished function.
- Inhibitory Compounds: Presence of inhibitory substances can noticeably compromise enzyme activity. Awareness and mitigation of such inhibitors can enhance overall enzyme efficiency.
Continuous research is necessary to explore strategies for improving stability. For instance, creating enzyme formulations that withstand varying conditions could bolster their efficacy in industrial applications. Additionally, implementing protein engineering techniques could produce stability-enhanced enzyme variants.
Regulatory and Ethical Considerations
The advancement of yeast enzymes brings with it a set of regulatory and ethical challenges that cannot be overlooked. Research and industrial implementation must adhere to guidelines that ensure safety and efficacy. As such, navigating the intricate web of regulations is paramount.
Key considerations include:
- Safety Assessments: Regulatory bodies like the FDA require stringent safety evaluations of enzymes before they're approved for use in food production or pharmaceuticals. This process can be time-consuming and resource-intensive.
- Ethical Concerns: Issues may arise regarding genetic modifications and their implications. For some communities, the use of genetically engineered yeast to optimize enzyme production fuels heated debates about natural vs. modified organisms and their impacts on ecosystems.
- Labeling Guidelines: Transparency in labeling is another regulatory aspect. Consumers increasingly seek awareness of the ingredients in their food. Companies must adhere to disclosure rules concerning the usage of genetically modified yeast, potentially influencing public perception and purchasing decisions.
"Enzyme stability and ethical considerations are the bedrock upon which successful yeast enzyme applications are built."
In synthesizing these insights, stakeholders must remain vigilant and proactive, as these complexities will shape the trajectory of yeast enzyme research and utilization in the forthcoming years.
The End and Implications
The exploration of yeast enzymes within biochemical processes holds pivotal importance not only for academic inquiry but also for practical applications across various industries. Understanding the intricate roles these enzymes play deepens our appreciation of biochemical dynamics while opening doors to novel innovations.
Key elements that underscore the relevance of this section include:
- Interconnectedness of Biochemical Processes: Yeast enzymes facilitate a crucial link between metabolism, fermentation, and biosynthesis. They catalyze transformations that are essential in industries from brewing to pharmaceuticals.
- Advancements in Enzyme Technology: As technology evolves, so does our ability to engineer yeast enzymes, which can enhance their stability and efficiency. This presents opportunities for more effective industrial applications.
- Health and Nutrition Implications: The enzymatic activity of yeast significantly impacts nutrient bioavailability. By better understanding these processes, we can possibly optimize dietary interventions and promote health.
The implications stretch wide. In commercial settings, for instance, enzyme optimization can lead to cost reductions and improved product quality, enhancing the overall market viability of products. From a research standpoint, the foundational insights gained from studying yeast enzymes can drive future explorations in realms like bioengineering and sustainable practices in food production.
"The potential of yeast enzymes transcends traditional boundaries, promising advancements in health and industry alike."
Summary of Key Insights
- Yeast enzymes are versatile catalysts, crucial for metabolic pathways, aiding in the conversion of substrates into necessary products.
- Their significance in practical applications, particularly in fermentation, leads to improved efficiencies in brewing and baking industries.
- Innovations in enzyme modifications can significantly enhance their applicability and stability.
- Comprehensive studies on yeast enzymes can inform better practices for nutrition and health, providing avenues for improved dietary formulations.
Potential for Future Research
Looking ahead, the field of yeast enzyme study presents a wealth of opportunities. Future research directions could include:
- Genomic and Proteomic Studies: Unraveling the genetic coding of enzymes may unveil novel enzyme classes or enhance existing ones, bridging the gap between yeast genetics and enzyme functionality.
- Enzyme Engineering: Age-old techniques could be complemented by cutting-edge methods, such as CRISPR-Cas9, to create tailored enzymes with extremely specific functionalities.
- Applications in Renewable Energy: Investigating yeast enzymes’ potential in biofuel production could lead to sustainable energy solutions, showcasing advantageous environmental impacts.
It's clear that tapping into the full capacity of yeast enzymes will not only bolster scientific knowledge but also expand their footprint across various industries, driving forward both scientific inquiry and practical applications.