The World of the Microscope: A Comprehensive Exploration
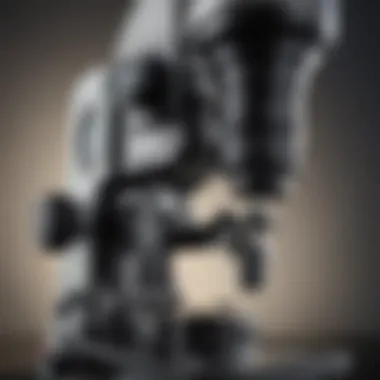
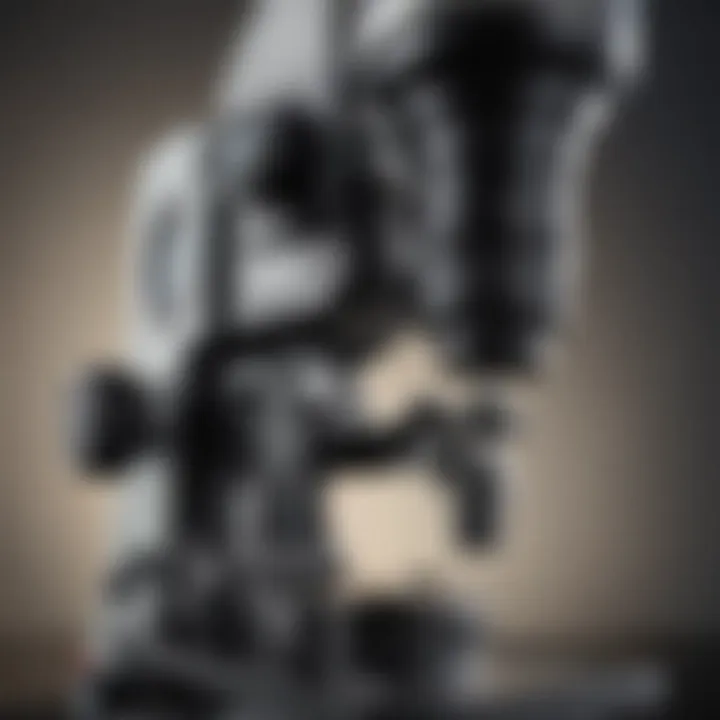
Intro
The microscope is a pivotal instrument that has transformed the landscape of scientific exploration. It enables the observation of objects that are too small for the naked eye, facilitating breakthroughs across various disciplines. The evolution of this technology illustrates humanity’s quest for knowledge and understanding in the microscopic world. As scientific fields like biology, chemistry, and physics develop, the role of the microscope adapts, increasingly shaping research methodologies and outcomes.
Methodologies
Description of Research Techniques
Utilizing a microscope encompasses various techniques that enhance the clarity and detail of observed specimens. Techniques such as optical microscopy enable scientists to visualize samples using visible light. Transmission electron microscopy (TEM) and scanning electron microscopy (SEM) have revolutionized the capacity to observe material at the nanoscale, providing insights into cellular structures and material properties.
Different staining methods are also vital, especially in biological contexts. For instance, histological staining enhances the contrast between different tissue types, allowing for more precise analysis of biological processes. These techniques collectively contribute to our understanding of microscopic phenomena and aid in the diagnosis of diseases.
Tools and Technologies Used
The advancements in microscopy have been accompanied by the development of sophisticated tools and technologies. Some key instruments include:
- Light Microscopes: They use lenses to focus light and magnify specimens, suitable for living cells and detailed morphology.
- Electron Microscopes: Such as TEM and SEM, they utilize beams of electrons, enabling high-resolution imaging of surfaces and internal structures.
- Fluorescence Microscopes: These employ specific wavelengths to excite fluorescently labeled samples, exposing intricate biological interactions.
The integration of computing technology has greatly enhanced image processing, analysis, and storage. This allows researchers easier access to vast data sets and fosters collaboration over long distances.
"The microscope is not merely a tool but a gateway to discovery, opening the door to worlds previously invisible to humanity."
Discussion
Comparison with Previous Research
Reflecting on the trajectory of microscopy reveals a marked improvement in both capability and accessibility. Early microscopes lacked the refinement and resolution required for modern scientific inquiries. Historical figures like Antonie van Leeuwenhoek laid the groundwork, but contemporary instruments allow far more detailed exploration. Today’s research can build on findings from the past with greater precision and breadth, facilitating interdisciplinary approaches and innovations.
Theoretical Implications
The impact of microscopy extends beyond mere observation. The theoretical implications inform multiple layers of scientific inquiry. While researchers may seek to understand cellular mechanics, they simultaneously contribute insights that can affect broader frameworks, such as evolutionary biology or materials science. The continuous evolution of microscopy promises to deepen these theoretical explorations, potentially leading to new paradigms within scientific thought.
Intro to Microscopy
Microscopy is an integral area of investigation that has considerably influenced scientific understanding across multiple disciplines. This introduction serves to highlight the cornerstone concepts of microscopy, fostering an appreciation for its evolution and significance in modern research.
Definition and Purpose
Microscopy refers to the technique of using instruments called microscopes to observe small objects or specimens that cannot be viewed with the naked eye. The primary purpose of microscopy is to magnify and resolve these minute details, allowing for a deeper understanding of structures, functions, and interactions at a microscopic level. This capability is vital in fields such as biology, where cell structures can be analyzed, and in materials science, where the properties of materials can be examined in detail.
"Microscopy has transformed our approach to understanding the biological and material worlds, illuminating phenomena invisible to the naked eye."
The importance of microscopy cannot be overstated. It is not just a tool but a gateway to insights into the fundamental aspects of life and matter. Through it, scientists have uncovered the intricacies of cell biology, validated hypotheses in chemistry, and fostered advancements in technological innovations such as nanotechnology.
Historical Context
The history of microscopy traces back to the late 16th century with the invention of the compound microscope. This invention was pivotal, marking the intersection of science and technology. Early contributors such as Hans and Zacharias Janssen, who developed simple lenses, laid the groundwork for future advancements. Galileo Galilei also played a significant role with his modifications to earlier designs, enhancing magnification capabilities.
In the 17th century, Antonie van Leeuwenhoek famously utilized simple microscopes to observe bacteria and protists. His observations opened new horizons for microbiology, thereby shifting the scientific paradigm. Over the years, microscopy went through various developments, including the refinement of optical systems and the eventual advent of electron microscopy in the 20th century, which further deepened our understanding of materials at an atomic scale.
Today, the rich historical context of microscopy not only highlights its growth but also emphasizes the continuous need for innovation in microscopy technologies. As research advances, so too does the necessity to explore and utilize different types of microscopy, each bringing unique capabilities and insights to the forefront of scientific discovery.
Types of Microscopes
Understanding the various types of microscopes is crucial in the study of microscopic analysis. Each type serves a unique purpose and has distinct benefits and limitations. By exploring these classifications, researchers can select the appropriate microscope for their specific needs. In this section, we will explore several categories, including optical microscopes, electron microscopes, scanning probe microscopes, and specialized microscopes.
Optical Microscopes
Optical microscopes are among the most widely used instruments in microscopy. They rely on visible light to illuminate samples and produce images. There are two main types within this category: compound microscopes and stereo microscopes.
Compound Microscopes
Compound microscopes are typically characterized by multiple lenses that provide high levels of magnification. This type is essential in fields such as biology and medicine, where detailed examination of cells and tissues is required. The key characteristic of compound microscopes is their ability to magnify samples up to 1000x or more, which makes them particularly effective for observing fine details.
A unique feature of compound microscopes is the use of oil immersion lenses, which enhance resolution by allowing light to enter at a greater angle. One major advantage is their capability to visualize stained specimens, which helps in enhancing contrast. However, these microscopes have some limitations; they require thin samples, which can be a hindrance when dealing with thicker specimens, reducing overall versatility.
Stereo Microscopes
Stereo microscopes provide a three-dimensional view of the sample, making them invaluable for applications where depth perception is vital. They are used extensively in fields like botany and electronics, where the surface structure of objects is analyzed. The key characteristic of stereo microscopes is their low magnification range, usually between 10x to 100x, coupled with a wide field of view.
The unique feature of stereo microscopes is that they use two eyepieces, allowing for binocular viewing. This aids in assessing depth and surface details, which is not possible with compound microscopes. One advantage is the ability to work with larger, thicker samples without precise slicing. Disadvantageously, they provide less detailed images compared to compound types, limiting their use for cellular structure studies.
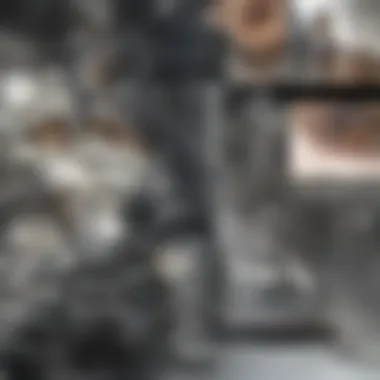
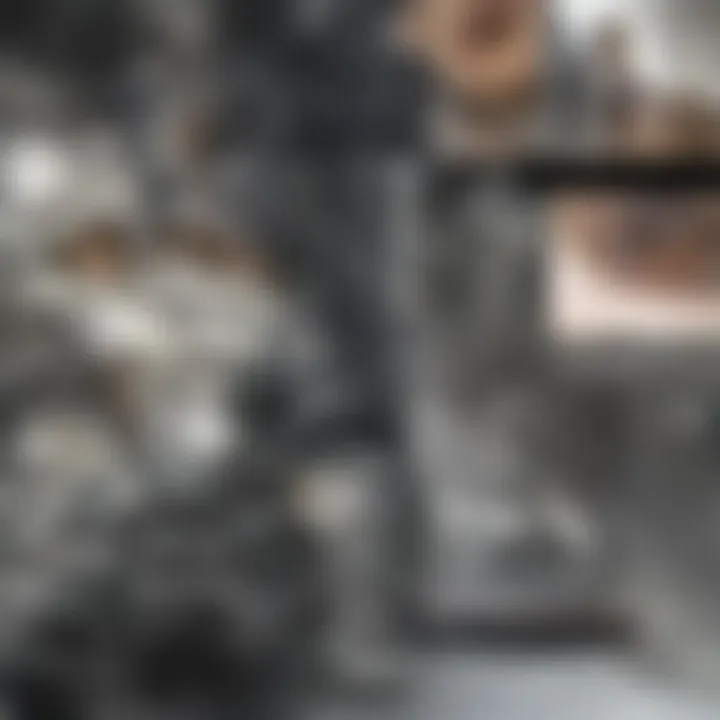
Electron Microscopes
Electron microscopes are critical in materials science, biology, and nanotechnology due to their high resolution and magnification capacities. They surpass optical microscopes by utilizing electrons instead of light to achieve much higher magnifications. Subcategories include transmission electron microscopes and scanning electron microscopes.
Transmission Electron Microscopes
Transmission electron microscopes (TEM) allow scientists to view internal structures within samples. A key characteristic of TEM is its ability to magnify objects beyond 1 million times. TEM is particularly beneficial for examining thin sections of biological samples and materials science applications.
A unique feature of transmission electron microscopes is the need to prepare ultra-thin samples. This can be an advantage as it allows for detailed internal examination. However, preparing these samples can be tricky and time-consuming, presenting a disadvantage in terms of workflow efficiency.
Scanning Electron Microscopes
Scanning electron microscopes (SEM) focus on surface characteristics, providing detailed three-dimensional images. The key characteristic of SEM is its versatility and ability to analyze bulk materials, coatings, and biological samples. SEM typically results in magnifications of up to 500,000x.
A unique feature of SEM is its capability to render high surface detail without requiring extensive sample preparation. It is also advantageous in producing images rapidly for larger samples than TEM can accommodate. However, SEM primarily provides surface information and lacks the ability to visualize internal structures effectively.
Scanning Probe Microscopes
Scanning probe microscopes operate at the nanoscale and are indispensable in nanotechnology. Key types include atomic force microscopes and scanning tunneling microscopes.
Atomic Force Microscopes
Atomic force microscopes (AFM) are utilized for topographical mapping at the atomic level. A key characteristic of AFM is its capability to measure forces between the probe and the sample, enabling precise analysis of surface structures. This makes AFM an essential tool in materials science, biology, and nanotechnology.
The unique feature of atomic force microscopes is their ability to operate in various environments, including air and liquid. This versatility is advantageous for observing live biological samples. One downside is that AFM does not provide detailed imaging at lower magnifications, limiting its use for larger samples.
Scanning Tunneling Microscopes
Scanning tunneling microscopes (STM) are groundbreaking as they manipulate individual atoms on surfaces. The key characteristic of STM is its extremely high resolution, providing atomic-level imaging of conductive surfaces. This feature is transformational for research in surface science and material studies.
Like AFM, the unique feature of scanning tunneling microscopes is their ability to visualize samples at atomic resolutions. Its precision is advantageous for studying surface properties. However, STM requires conductive materials and does not work effectively with non-conductive samples, which can restrict its applicability.
Specialized Microscopes
Specialized microscopes are designed for specific applications, enhancing imaging techniques. This category includes fluorescence microscopes and confocal microscopes.
Fluorescence Microscopes
Fluorescence microscopes employ fluorescence to illuminate samples stained with specific dyes, allowing for the visualization of proteins and other cellular components. The key characteristic is the ability to achieve high contrast images of specific target molecules. This is impactful in cellular biology and medical diagnostics.
A unique feature of fluorescence microscopes is their capability to perform live-cell imaging. This feature is advantageous for studying dynamic cellular processes in real-time. On the downside, the requirement for fluorescent stains can alter the sample and may not be suitable for all types of studies.
Confocal Microscopes
Confocal microscopes improve image resolution and contrast by using lasers to scan samples, obtaining optical sections at varied depths. The key characteristic of confocal microscopes is their ability to discern pinpoint details while reducing background noise. This capability is beneficial for thick specimens requiring precise analysis.
A unique feature of confocal microscopes is their ability to produce 3D images from multiple optical sections. The advantage lies in obtaining clearer images of complex structures. However, the complexity and cost of confocal microscopes can be a barrier for some labs.
Overall, understanding the types and functionalities of microscopes provides clarity in selecting the right instrument for specific scientific inquiries. Each category has its distinct strengths and weaknesses, and comprehending these can enhance research outcomes.
The Mechanics of Microscopy
Understanding the mechanics of microscopy is fundamental to grasping how this powerful tool functions. The interplay between optics, light, and sample preparation forms the backbone of any microscopy effort. In this section, we will explore three pivotal components: lenses and light, magnification and resolution, and sample preparation techniques. Together, these elements illuminate how microscopes produce the detailed images that have shaped scientific discovery in numerous fields.
Lenses and Light
At the heart of microscopy are the lenses, which focus light onto the samples being observed. Light microscopes generally use glass lenses that bend light to magnify images. The quality of these lenses is paramount. With high-quality optics, users can achieve clear and crisp images. However, optical microscopes are limited by the wavelength of visible light, which restricts the close examination of materials at the nanoscale.
On the other hand, electron microscopes use electron beams instead of light. Electrons have much shorter wavelengths, making it possible to achieve much higher resolutions. This allows scientists to observe structures at atomic scales. Importantly, the choice between light and electron beams depends on the specifics of the research aims.
Magnification and Resolution
Magnification is the apparent increase in size of a specimen when viewed through a microscope. However, it is essential not to confuse magnification with resolution. Magnification allows for the enlargement of images, while resolution defines the clarity and detail within those images. It is possible to have high magnification paired with low resolution, resulting in unclear images.
Achieving optimal resolution requires precise alignment of lenses, stabilization of light sources, and careful selection of viewing methods. Factors like numerical aperture (NA) and condenser placement play crucial roles in this. Furthermore, some modern microscopes come equipped with digital imaging technology, which enhances both magnification and resolution.
Sample Preparation Techniques
Sample preparation is a critical part of microscopy that directly affects the quality of the observed images. Improperly prepared samples can lead to artefacts that obscure true observations. Typical preparation methods vary by microscopy type. For optical microscopy, samples often need to be thinly sliced, stained, or mounted on slides for effective viewing. Staining can highlight specific parts within cells or tissues, revealing more details about their structure and function.
In contrast, electron microscopy requires even more stringent preparation. Samples must often be conductive, as non-conductive materials can produce charging effects. To make them suitable for electron imaging, they might undergo dehydration or be coated with a thin layer of metal. Proper preparation techniques not only improve image quality but also reliability in the results obtained through microscopy.
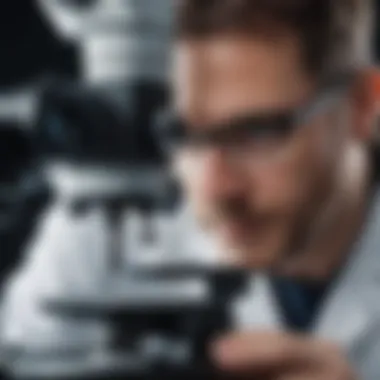
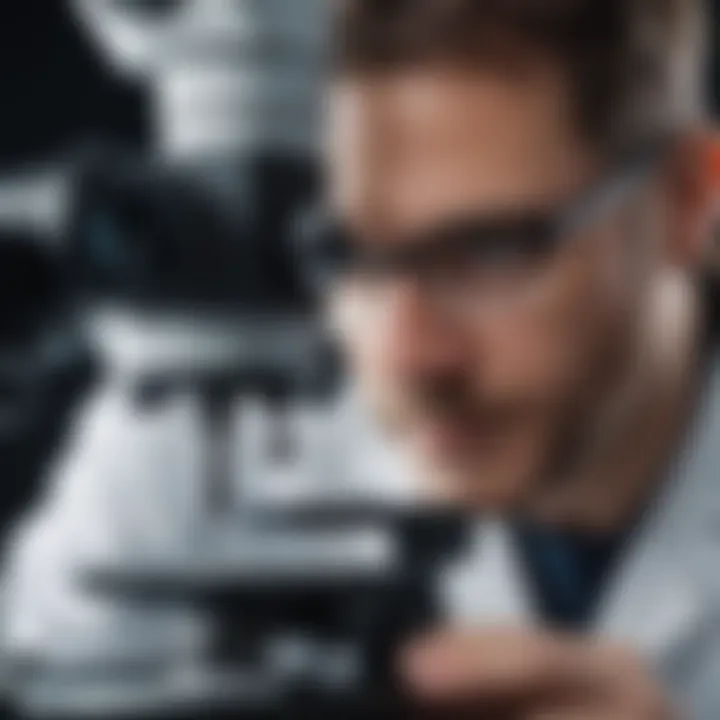
"Proper sample preparation can enhance resolution and reduce artefacts in microscopy, ensuring more reliable findings."
In summary, the mechanics of microscopy—encompassing lenses and light, magnification and resolution, and sample preparation techniques—are essential components that determine the efficacy and accuracy of the observations made. Mastery of these mechanics enhances the entire microscopy experience, revealing insights across the scientific spectrum.
Applications of Microscopy
Applications of microscopy span across various disciplines, making it a fundamental tool in scientific research and analysis. The ability to magnify and visualize objects at a microscopic level uncovers details that are crucial for numerous fields, including biology, materials science, chemistry, and environmental science. Understanding these applications is important as it highlights how microscopy facilitates advancements in knowledge and practices.
Biology and Medicine
Cell Structure Analysis
Cell structure analysis is invaluable for understanding the complexities of biological organisms. It allows researchers to observe cellular components such as membranes, organelles, and even molecular structures within cells. This form of microscopy plays a crucial role in cell biology, aiding in the identification of cellular functions and interactions.
A key characteristic of cell structure analysis is its utilization of techniques like optical and electron microscopy, which provide high-resolution images of cells. This makes it a popular choice for microscopic studies as it reveals intricate details essential for advanced research. The unique feature of cell structure analysis lies in its capability to visualize live cells in some methods, offering insights into biological processes in real time.
The primary advantages of this approach include better understanding of diseases and cellular behaviors. However, a disadvantage is the complexity involved in sample preparation, which can sometimes lead to artifacts that misrepresent the true state of cells.
Disease Diagnosis
In the realm of medicine, microscopy is central to disease diagnosis. It aids in the identification of pathogens, cancer cells, and various abnormalities within tissues. The use of microscopy in diagnostics has revolutionized patient care, allowing for precise identifications that guide effective treatments.
A hallmark of disease diagnosis through microscopy is its ability to differentiate between healthy and diseased tissues, often using staining techniques to enhance visibility. This is a beneficial method as it reduces reliance on invasive tests when possible. Furthermore, unique to disease diagnosis is the growing integration of molecular techniques with microscopy, enhancing its utility in identifying disease at a genetic level.
The advantages of employing microscopy in diagnostics are clear: faster, more accurate results can significantly improve treatment timelines. However, it requires highly skilled operatives and may involve expensive equipment, which can be considered a limitation.
Materials Science
Microstructural Analysis
Microstructural analysis involves studying the internal microstructures of materials, such as metals or polymers. This application has profound implications in ensuring the integrity and performance of materials used in construction and manufacturing.
A defining characteristic of microstructural analysis is its ability to reveal the arrangement of grains and phases within materials. This is crucial for understanding properties like strength, ductility, and fatigue resistance. Such insights make it a favorable approach in materials science, as they directly influence the selection of materials for specific applications.
The unique aspect of this analysis is often its combination with techniques like electron microscopy, providing a detailed view of atomic structure. The primary advantage is the ability to optimize materials for desired properties. However, it can be time-consuming and requires meticulous sample preparation.
Nanotechnology Applications
Nanotechnology applications leverage the principles of microscopy to manipulate materials at the molecular level. This emerging field holds potential for breakthroughs in various sectors, including healthcare, electronics, and environmental science.
A key characteristic of nanotechnology applications is the ability to observe and manipulate structures at the nanoscale, making it a cutting-edge choice for researchers. The unique feature of microscopy in this context allows scientists to visualize the behavior of nanomaterials under different conditions, such as temperature and pressure.
Advantages include the exploration of innovative materials with enhanced properties, but challenges persist in terms of scalability and reproducibility, creating a careful balance in nanotechnology ventures.
Chemistry
Chemical Reactions Observation
In chemistry, observing chemical reactions through microscopy provides essential insights into reaction mechanisms and kinetics. It allows chemists to visualize changes in substance at the molecular level, paving the way for better understanding and optimization of reactions.
A key characteristic of this application is the direct observation of reactions, which can be achieved through advanced imaging techniques like fluorescence microscopy. This is beneficial for chemists as it aids in real-time analysis and leads to well-informed conclusions about reaction pathways.
A unique aspect of this method is its capability to track dynamic processes, such as the formation of products from reactants. While the advantages include increased knowledge of complex reactions, limitations can arise due to potential interference from the observation techniques used.
Catalyst Studies
Catalyst studies rely on microscopy to explore the surface properties and active sites of catalysts, which are vital for numerous chemical reactions. This application significantly advances the understanding of how catalysts facilitate reactions, crucial in fields such as petrochemical production and green chemistry.
A notable characteristic is that these studies reveal the microscopic arrangements that affect catalytic efficiency. This is particularly beneficial as understanding these properties can lead to the development of better catalysts.
The unique feature of catalyst studies is the ability to analyze the catalysts in situ, meaning under working conditions, which provides practical insights. However, challenges can arise with experimental controls and variability in results based on the microscopic techniques applied.
Environmental Science
Pollution Analysis
In environmental science, pollution analysis helps in assessing the impact of pollutants at microscopic levels. This includes identifying contaminants in air, water, and soil. The significance of this analysis extends beyond mere detection; it supports policy-making and remediation efforts.
A critical aspect of pollution analysis is its role in understanding the specific particles that contribute to environmental degradation. This makes it a beneficial choice as it can guide targeted actions towards mitigation. The unique capability of microscopy allows for the identification of pollutants even at very low concentrations, enhancing its effectiveness.
However, while this method is powerful, it can be costly, and involves extensive training to ensure proper analysis.
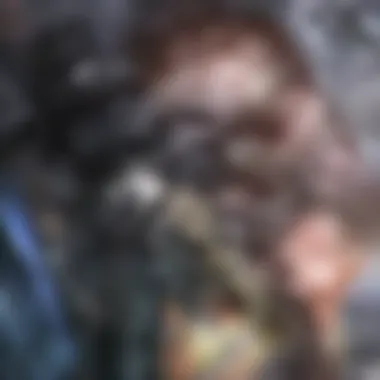
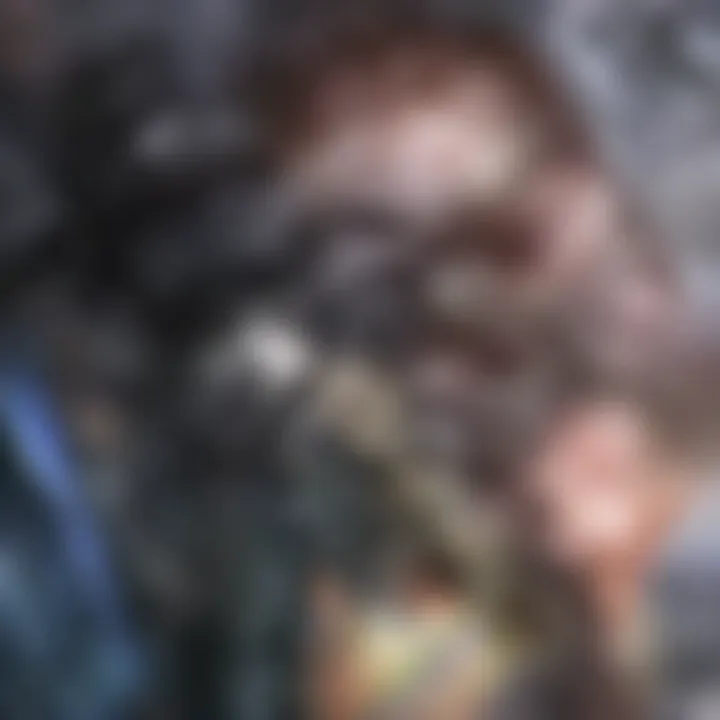
Microbial Studies
Microbial studies use microscopy to explore various microorganisms, particularly in aspects like ecology, health, and biotechnology. This application provides essential data about microbial diversity, behavior, and the role of microbes in various environments.
A key characteristic of microbial studies is their capacity to observe microorganisms in their natural habitats, which reveals interactions and community structures. This makes it a popular choice for researchers studying ecosystems and health.
The unique feature of this method is the feasibility of using techniques like fluorescence in situ hybridization to target specific microbial populations. The advantages of such studies include profound insights into microbial roles in ecosystems. However, a disadvantage is the complexity of analyzing and interpreting high-throughput data, often generated through modern techniques.
Recent Innovations in Microscopy
In recent years, microscopy has experienced significant advancements. These innovations improve the capabilities of traditional microscopy and introduce new techniques that enhance our understanding of complex biological and material structures. The integration of digital solutions, three-dimensional imaging, and artificial intelligence has opened new frontiers. This section examines these recent developments and their implications for scientific research.
Digital Microscopy
Digital microscopy represents a substantial shift from traditional optical techniques. It utilizes digital cameras to capture images directly from the microscope. One main advantage is the ability to view and manipulate images in real-time. Researchers can enhance contrast, adjust brightness, and analyze samples without needing physical alterations. This method not only streamlines workflows but also allows for improved documentation and sharing of findings.
Users can easily record and store images, facilitating data management. Many digital systems also support software for image analysis, thus providing tools for advanced metrics like quantitative size measurements and automated counting of particles. This approach enhances productivity in laboratories and educational settings.
3D Imaging Techniques
Three-dimensional imaging techniques have vastly improved our ability to visualize samples in a spatial context. Traditional microscopy typically offers only a two-dimensional view. However, methods like confocal microscopy and two-photon microscopy allow for the imaging of thick biological specimens. This capability is crucial for studying structures such as tissues or cellular interactions, providing insights that would otherwise be obscured in flat images.
3D imaging enables scientists to reconstruct sample dimensions accurately. Moreover, it helps visualize processes within live cells over time, offering a dynamic perspective on biological activities. This dimensional depth helps in understanding complex interactions and structures in ways that 2D images cannot convey effectively.
AI and Machine Learning Integration
The integration of artificial intelligence and machine learning into microscopy marks a transformative era for this field. These technologies can analyze vast amounts of data rapidly, identifying patterns that might elude time-strapped researchers. For example, AI algorithms can classify cell types within images based on subtle morphological differences, considerably speeding up the analyzing process.
Moreover, machine learning models can improve image quality using denoising techniques, enhancing clarity in captured data. Some tools can even predict sample outcomes based on previous data, aiding in experimental design and hypothesis generation. This evolution empowers researchers to focus more on interpretation and less on data handling, thus amplifying scientific discovery.
"In microscopy, innovations in technology enhance not just visualization, but also our understanding of the microscopic world."
As microscopy continues to evolve, it promises to reveal more about the intricate details of life and materials. By adopting these innovations, scientists will further integrate interdisciplinary approaches, potentially unlocking new discoveries across various fields like biology, chemistry, and environmental science.
Challenges in Microscopy
The field of microscopy is significant for many scientific disciplines, but it comes with its own set of challenges. Understanding these challenges is vital for researchers who aim to push the boundaries of what is possible in microscopy. This section explores the limitations of current techniques and outlines potential future research directions that could address these challenges.
Limitations of Current Techniques
Current microscopy techniques face several limitations that can hinder research progress. Some of the noteworthy limitations include:
- Resolution Constraints: Despite advances in technology, many optical microscopes are limited by the diffraction limit, which restricts the ability to resolve finer details. This affects studies on cellular structures, where high resolution is necessary to observe subtle differences in morphology.
- Sample Preparation: Sample preparation can be invasive. Many techniques require that specimens be fixed or stained, which can alter their natural state or introduce artifacts. This is particularly concerning in biological studies, where the organism's behavior and structure may change during preparation.
- Imaging Depth: In biological tissues, the imaging depth can be shallow. For example, fluorescence microscopy is often limited to a few micrometers below the surface, making it hard to study larger biological specimens.
- Cost of Equipment: Advanced microscopy techniques, such as scanning electron microscopy and super-resolution microscopy, can be prohibitively expensive. This creates barriers for small labs and institutions with limited budgets, constraining the reach of high-quality research.
- Data Management: High-resolution images produce vast amounts of data, necessitating substantial storage and sophisticated data analysis methods. Many researchers struggle to manage and analyze this data effectively.
"Understanding these limitations is crucial for developing next-generation tools in microscopy."
Future Research Directions
Looking into the future, several areas in microscopy warrant further investigation to address the existing challenges:
- Development of Novel Optical Techniques: Research is underway to create new optical microscopes that can overcome the diffraction limits. Techniques such as super-resolution and four-dimensional microscopy are promising avenues worth exploring in detail.
- Improved Sample Preparation Methods: Innovations in non-invasive imaging techniques can help maintain the integrity of specimens. The exploration of new staining methods or technologies that allow for live imaging without alterations will be essential.
- Multi-Modal Imaging: Integrating multiple imaging modalities can provide a richer dataset, allowing for more comprehensive studies of specimens. Combining fluorescence with electron microscopy, for example, could help in correlated studies where both surface and internal structures need simultaneous examination.
- Machine Learning for Image Analysis: As microscopy produces more data, machine learning can facilitate more efficient data processing and interpretation. Continued development in algorithms can enhance image recognition, automate analysis, and derive insights that may be challenging for human analysis alone.
- Accessibility and Cost Reduction: Driven by advancements in technology, future efforts should focus on reducing the costs associated with high-end microscopy tools. Making advanced microscopy techniques more accessible can democratize research opportunities and inspire new scientific inquiries across various fields.
In summary, the challenges in microscopy reflect its complexity and the necessity for continuous improvement in techniques and protocols. Addressing these challenges through focused research can unlock new frontiers in scientific discovery.
The Future of Microscopy
The future of microscopy holds immense potential and significance. As technology continues to advance, the methodologies and capabilities of microscopy evolve. This section will explore emerging technologies and the potential scientific breakthroughs that may arise from developments in this essential field. Understanding the future of microscopy is crucial for various reasons.
Firstly, it highlights the role of innovation in scientific research. By keeping an eye on upcoming technologies, researchers can adapt to changes and improve their methodologies. Secondly, it serves to inform educational institutions about the evolving landscape of microscopy, ensuring that students and professionals are equipped with the necessary skills. Finally, recognizing trends in microscopy aids in investment and resource allocation, both in academic and commercial sectors.
Emerging Technologies
Emerging technologies are reshaping the way microscopy is utilized across disciplines. One such development is super-resolution microscopy. This technique transcends the diffraction limit of light, enabling scientists to observe structures at nanometer scales. Traditional optical methods face constraints, but super-resolution methods like STORM and PALM break these barriers, facilitating unprecedented exploration in cellular biology and materials science.
Another advancement is the integration of photonic crystals. These materials allow for enhanced imaging capabilities and better light manipulation, which can lead to sharper images.
- Multiphoton microscopy offers the ability to penetrate deeper into samples without causing significant damage. It is particularly useful in studying live tissues, making it invaluable in medical research.
- Machine learning and AI applications are increasingly being implemented in microscopy for image analysis. These algorithms can process vast amounts of data quickly, identifying patterns that the human eye might miss.
Potential Scientific Breakthroughs
The advancements made in microscopy herald the potential for significant scientific breakthroughs. In the realm of biomedicine, improved microscopy may enable early detection of diseases at the cellular level, allowing for timely intervention. Innovations like high-content screening can transform drug discovery, giving researchers insights into the effects of compounds on live cells at an unprecedented scale.
In materials science, advanced imaging techniques can provide insights into nano-scale structures and properties, leading to the development of stronger, lighter, and more efficient materials. This could have implications in fields ranging from defense to electronics.
"The advancements in microscopy are not just about seeing things better; they are fundamentally about understanding the universe at a much deeper level."
Furthermore, as environmental concerns continue to rise, microscopy will play a crucial role in monitoring and understanding pollutants at microscopic levels. This may lead to breakthroughs in environmental science, aiding in the development of cleanup methods and sustainable practices.