Whole Genome Sequencing: An In-Depth Overview
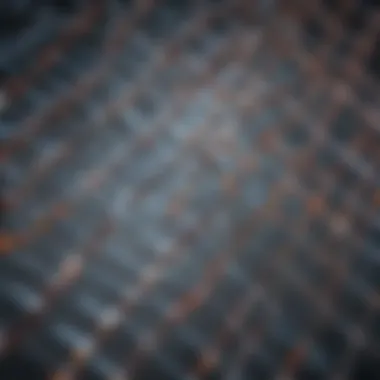
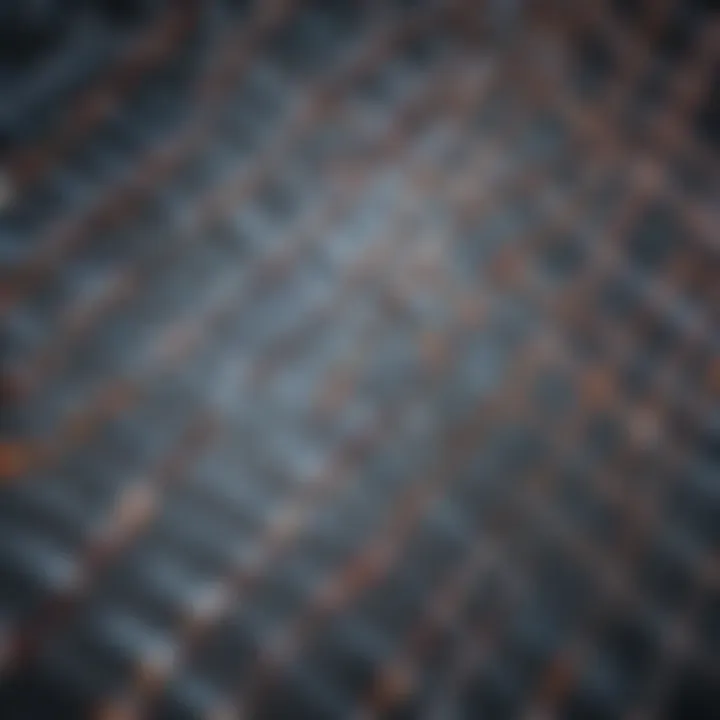
Intro
Whole genome sequencing (WGS) represents a significant leap in understanding the genetic architecture of organisms. This process allows researchers to decode the entire DNA sequence of an organism, providing critical insights that span various fields including medicine, evolutionary biology, and genetics. The evolution of genomic technologies has placed WGS at the forefront of research, enabling advancements in personalized medicine, disease prevention, and the comprehension of hereditary conditions.
This article examines the WGS process in detail, emphasizing specific methodologies used, technological advancements, and broader implications. By navigating through this comprehensive overview, readers can appreciate the intricacies involved and the transformative potential of genomic research.
Methodologies
Description of Research Techniques
The methodologies behind whole genome sequencing encompass several steps that are essential for accurate and reliable results. Initially, sample collection and preparation form the foundation of WGS. Samples can come from blood, saliva, or even tissue biopsies. Once collected, they undergo extraction and purification of the DNA. This step is crucial because the quality of the DNA obtained can directly affect the sequencing outcome.
Following extraction, the DNA is fragmented into smaller pieces. This fragmentation can be achieved through mechanical means or chemical processes. The resulting fragments are then subjected to library preparation. Library preparation involves adding specific adapters to the DNA fragments, which play a vital role in the sequencing process. These adapters allow the fragments to bind to the sequencing platform, ensuring that they are correctly identified and sequenced.
Tools and Technologies Used
A variety of sequencing technologies have emerged over the years, each with unique qualities. Some of the most commonly used methods include:
- Sanger Sequencing: This technique is often considered the gold standard for DNA sequencing. It is highly accurate but also quite labor-intensive and time-consuming.
- Next-Generation Sequencing (NGS): NGS has revolutionized WGS by allowing massive parallel sequencing of DNA fragments. This method significantly reduces the time and cost associated with genomic sequencing.
- Third-Generation Sequencing: This includes technologies like PacBio and Oxford Nanopore, which enable longer reads and can provide insights into structural variations within genomes.
These tools enhance the quality and speed of data collection in genomic studies. Each method has its own strengths, which researchers select according to the specific requirements of their studies.
Discussion
Comparison with Previous Research
Whole genome sequencing represents a significant advancement compared to previous methods of genetics research, such as single-gene sequencing. While earlier techniques provided insights into specific genes or regions of the genome, WGS enables a holistic view of the entire genetic makeup. This comprehensive understanding allows for a more nuanced analysis of complex traits, guiding research beyond mere associations and into causative relationships.
Theoretical Implications
As WGS continues to evolve, it brings forward critical theoretical implications. The data generated through WGS has the potential to challenge existing paradigms in genetics. For instance, understanding the complete genetic canvas of an organism can shed light on polygenic traits, elucidating how multiple genes contribute to a particular phenotype or disease. This deeper understanding can influence future research directions, pushing boundaries of what is known about gene interaction and expression.
"Whole Genome Sequencing is not only a tool for medical advancements but also a key to unlocking the mysteries of evolution and genetics."
By exploring the methodologies and implications of whole genome sequencing, this article educates readers about its vital role in transforming research and applying findings in real-world scenarios. As ethical considerations loom large, it is pivotal to navigate these developments with caution, ensuring equitable access and responsible use of genomic information.
Preamble to Whole Genome Sequencing
Whole genome sequencing (WGS) represents a cornerstone in genomics, allowing scientists and medical professionals to explore the complete DNA sequence of an organism. This comprehensive analysis serves as a powerful tool in various fields, such as medicine, evolutionary biology, and environmental science. WGS enables the identification of genetic variations that can influence traits and susceptibility to diseases.
The significance of WGS is underscored by its capacity to advance personalized medicine, which tailors medical treatment to the individual's genetic makeup. Additionally, understanding the entire genome provides insights into evolutionary processes and relationships among species. Given the vast potential applications, recognizing the framework of whole genome sequencing is paramount for students, researchers, educators, and professionals alike.
Definition of Whole Genome Sequencing
Whole genome sequencing is the process of determining the complete DNA sequence of an organism’s genome at a single time. This includes not only the coding regions of genes but also the non-coding regions, which play important regulatory roles. WGS provides a comprehensive map of the genome, presenting an exhaustive catalog of genetic variants, from single nucleotide polymorphisms to larger structural changes. By acquiring such detailed genetic information, researchers can enhance their understanding of biological functions and disease mechanisms.
Historical Context of Genomic Studies
The journey of genomic studies began with the discovery of DNA structure by James Watson and Francis Crick in 1953. This pivotal moment set the stage for future genomic research. In the following decades, scientists made advancements in sequencing techniques, notably the development of Sanger sequencing in the late 1970s, which allowed for the sequencing of relatively small lengths of DNA.
The completion of the Human Genome Project in 2003 marked a significant milestone, providing the first complete human genome sequence. This enormous collaborative effort facilitated the mapping of genetic information, sparking interest in larger-scale genome projects across diverse organisms. Essentially, the historical backdrop enriches our understanding of how WGS has evolved from theoretical models to actionable insights within various scientific disciplines.
Technical Foundations
The technical foundations of whole genome sequencing (WGS) are crucial in understanding how this process works. These basics help frame the overarching methodologies and technologies used to analyze DNA. Knowing these principles is essential for anyone involved in the fields of genomics, bioinformatics, or related research. Here, we will break down the basic principles of DNA sequencing and discuss key technologies fundamental to WGS.
Basic Principles of DNA Sequencing
DNA sequencing refers to determining the precise order of nucleotides within a DNA molecule. Each sequence consists of four types of nucleotide bases: adenine (A), thymine (T), cytosine (C), and guanine (G). The sequence in which these bases are arranged encodes genetic information.
Understanding the basic principles of sequencing is important for comprehending how changes in the sequence can impact biological functions.
The process begins with the amplification of the DNA, ensuring enough material is available for analysis. This is often done through polymerase chain reaction (PCR) techniques. Once amplified, the DNA is either fragmented or prepared in a manner suitable for sequencing, ensuring the bases can be read accurately. After preparation, the actual sequencing phases commence, where the chemistry and technology involved come into play.
Key Technologies in Whole Genome Sequencing
Several key technologies stand out in the process of WGS. Each one plays a significant role in the sequencing workflow, having unique features and contributions to the field. Let's explore three predominant technologies.
Sanger Sequencing
Sanger sequencing is one of the earliest methods developed for sequencing DNA. It uses a technique called chain termination where specific modified nucleotides prevent further elongation of the DNA strand. This results in fragments of varying lengths, which can then be separated by size using gel electrophoresis.
A key characteristic of Sanger sequencing is its high accuracy, making it a reliable choice for smaller-scale sequencing projects. However, it is slower and more expensive compared to newer technologies.
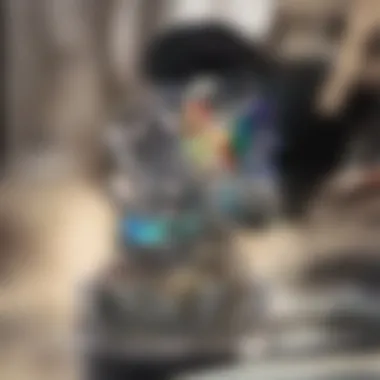
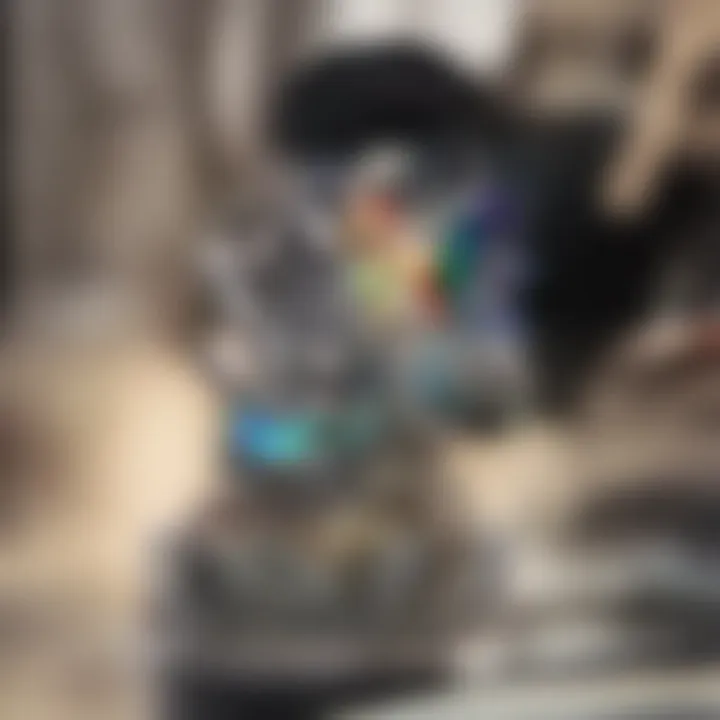
Advantages of Sanger sequencing include:
- High accuracy and precision for small sequences.
- Effective for targeted sequencing efforts.
Disadvantages include:
- Limited throughput for larger genomes and longer processing time relative to modern methods.
Next-Generation Sequencing (NGS)
Next-Generation Sequencing represents a revolution in sequencing technology, allowing for massive parallel processing of DNA. This method enables the rapid sequencing of entire genomes at a fraction of the cost and time of Sanger sequencing. NGS methods produce millions of short reads, which can be assembled through computational approaches to reconstruct the entire genome.
The key characteristic of NGS is its high throughput, which is particularly beneficial for large-scale studies such as population genomics.
Advantages of NGS include:
- Capability to process vast amounts of data quickly.
- Versatility in application from small targeted panels to whole genomes.
Disadvantages involve:
- Lower accuracy in individual read quality compared to Sanger sequencing.
- Computational complexity for data analysis and interpretation.
Third-Generation Sequencing (TGS)
Third-Generation Sequencing marks another leap forward, focusing on sequencing long stretches of DNA in real-time. Unlike NGS, TGS technologies can read longer fragments, which helps produce a more comprehensive view of genomic regions that may be challenging to assemble accurately. This is essential for resolving complex genomic structures and repetitive regions.
A notable characteristic of TGS is its ability to generate very long reads, which are valuable for understanding structural variants within genomes.
Advantages of TGS include:
- Long read lengths allow for better assembly and complex variation detection.
- Real-time sequencing capabilities facilitate rapid results.
Disadvantages include:
- Higher costs and still emerging technology with some limitations in throughput compared to NGS.
Understanding these technical foundations and technologies provides a solid base for comprehending the broader implications and applications of whole genome sequencing. Each method contributes uniquely to the evolving landscape of genomic research.
The Whole Genome Sequencing Process
Whole genome sequencing (WGS) is at the forefront of genomic research, providing a complete view of the genetic blueprint of an organism. Understanding this process is crucial as it informs various applications in medicine, research, and agriculture. The importance of WGS lies in its ability to offer detailed insights, guiding decisions that can lead to advancements in personalized medicine and disease prevention.
The process of WGS involves several key steps, including sample collection, DNA extraction, sequencing, and data analysis. Each step contributes significantly to the overall process and success of genome sequencing.
Sample Collection and Preparation
Types of Biological Samples
Biological samples are the foundation of whole genome sequencing. They can include blood, saliva, tissues, and even environmental samples. Blood samples are often preferred due to their abundance of genomic material. Saliva is a less invasive option, easily collected but may yield lower DNA quality. The choice of sample affects the quality of the genomic data.
When it comes to tissue samples, the specificity and integrity are crucial. Freshly frozen tissues usually provide high-quality DNA, but they require meticulous handling to avoid degradation. Each type of sample has its unique features leading to different advantages and disadvantages in the WGS process.
Extraction of DNA
Extraction of DNA from biological samples is a vital step in WGS. Various methods exist, like silica-based, phenol-chloroform extraction, or magnetic bead methods. The goal is to obtain a pure and intact DNA sample.
Silica-based methods are popular for their rapidity and efficiency. Magnetic bead methods are beneficial because they minimize contamination. However, some methods may yield lower quantities of DNA, impacting downstream applications.
Quality Control Measures
Quality control measures are essential to ensure that the DNA extracted is suitable for sequencing. Quality assessment techniques, such as spectrophotometry and gel electrophoresis, help verify DNA integrity and purity.
High-quality DNA is critical for accurate sequencing results. Poor quality DNA can lead to misinterpretation of data, impacting research outcomes and clinical applications. Implementing robust quality checks at this stage contributes to the reliability of whole genome sequencing.
Sequencing Methods and Techniques
Library Preparation
Library preparation is necessary before sequencing can occur. This involves fragmenting DNA and attaching specific adapters that allow sequencing machines to recognize and process the DNA.
The method of library preparation can influence both the quality and quantity of the data obtained. Specific techniques, such as PCR amplification or ligation, may be employed depending on the desired outcomes. Each technique comes with its own set of advantages and challenges, impacting sequencing results.
Sequencing Platforms

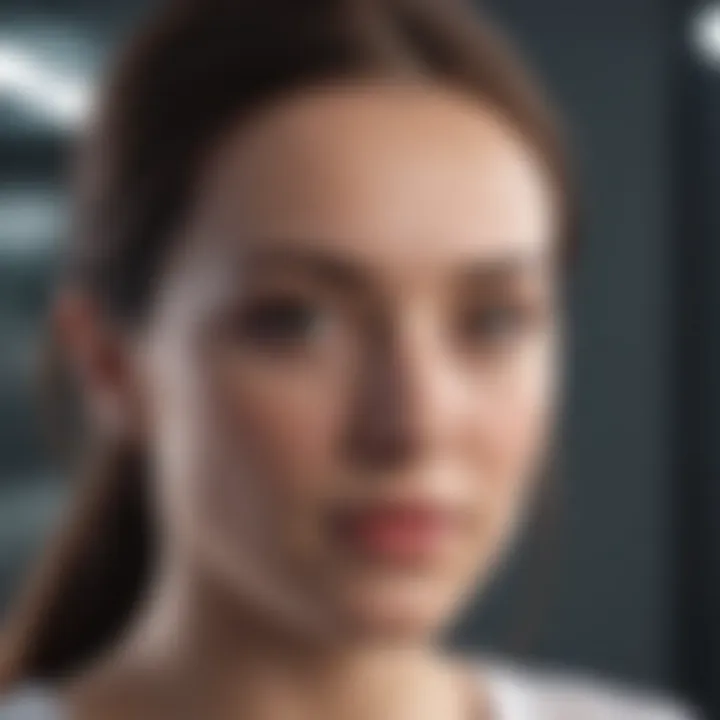
The choice of sequencing platform is crucial in defining the nature of the data produced. Illumina, PacBio, and Oxford Nanopore Technologies are popular platforms, each offering unique advantages.
Illumina's sequencing technology is known for high throughput and accuracy. PacBio provides longer read lengths, suitable for complex genomic areas. Oxford Nanopore allows for real-time sequencing, giving researchers flexibility in data acquisition. The choice of platform can dictate the speed, cost, and depth of sequencing, ultimately influencing the outcomes of genomic projects.
Read Length and Coverage Depth
Read length and coverage depth are critical metrics in whole genome sequencing. Read length refers to the size of the DNA fragments sequenced, while coverage depth indicates how many times a given region is sequenced.
Longer reads can resolve complex repetitive regions, while deeper coverage can enhance confidence in variant calling. Balancing read length and coverage depth is essential to ensure accurate and complete genome assembly. The decision on these parameters can greatly affect the quality and usefulness of the sequencing results.
Data Analysis and Interpretation
Bioinformatics Tools
Bioinformatics tools analyze and interpret the raw sequence data generated from WGS. These tools help in aligning sequences to reference genomes, identifying variations, and visualizing data.
Popular bioinformatics tools like GATK or SAMtools play a pivotal role in streamlining the analysis process. The selection of appropriate tools can significantly impact the quality of results yielded from data analysis, as different tools are optimized for various types of analysis.
Variant Calling
Variant calling is a fundamental aspect of data analysis. It involves identifying genetic variations, such as single nucleotide polymorphisms (SNPs) and structural variants. Accurate variant calling is essential for understanding genetic diseases and for applications like personalized medicine.
The methods used in variant calling affect the sensitivity and specificity of the results. Tools such as FreeBayes or Sentieon are widely used in the field. The choice of tools is driven by the specific requirements of the research being conducted.
Annotation and Functional Analysis
Once variants are called, annotation is performed to interpret their biological significance. Functional analysis determines the impact of variants on genes and potentially on phenotype. This step is vital for translating genomic data into meaningful insights that can influence clinical decisions.
A variety of databases and tools, like snpEff or ANNOVAR, are used for annotation. Choosing the right tools for annotation can enhance the understanding of genetic variants. However, challenges in interpreting variants with unknown significance persist, highlighting the complexity of genomic data analysis.
Understanding the whole genome sequencing process provides insights that drive advancements in various domains of biology and medicine. The implications of this process are vast, influencing healthcare, research, and ethical discussions surrounding genetic data.
Applications of Whole Genome Sequencing
Whole Genome Sequencing (WGS) is not just a potent scientific tool; its applications span across numerous fields, significantly shaping modern biology and medicine. Understanding the applications of this technology sheds light on its relevance and impact. From clinical settings to research contexts, the uses of WGS demonstrate its importance in resolving intricate biological questions and advancing health care.
Clinical Applications
Personalized Medicine
Personalized medicine, or precision medicine, tailors medical treatment to individual characteristics, needs, and preferences of patients. The advent of WGS has enhanced the capabilities of personalized medicine by allowing healthcare providers to understand the genetic underpinnings of a patient’s condition. A key characteristic of personalized medicine is its focus on genetic variation among individuals. This is beneficial for precisely targeting therapies, leading to improved outcomes.
The unique feature of personalized medicine is its emphasis on genetic information to make clinical decisions. For example, doctors can analyze specific variants that may predict an individual's response to medication, thus avoiding adverse reactions or ineffective treatments. However, it is essential to note that the complexity of genetic data can sometimes lead to challenges in interpretation.
Oncology and Cancer Genomics
Oncology has greatly benefited from the insights provided by WGS. Cancer genomics, in particular, focuses on the study of genetic mutations that lead to cancer development. A significant aspect of oncology today is understanding tumor heterogeneity – how varied cancer cells can be within the same tumor. This insight supports the identification of targeted therapies based on specific mutations found in cancer cells.
The key characteristic that makes cancer genomics popular is the potential for precision treatment. By leveraging genetic information, oncologists can choose therapies that specifically target the mutations driving a patient’s cancer. The unique aspect of this approach is the capacity to monitor tumor evolution and adjust treatment plans accordingly. Nonetheless, limitations such as cost and the need for advanced bioinformatics tools may hinder widespread utilization.
Infectious Diseases
Infectious disease research increasingly relies on WGS to track and control outbreaks. Sequencing the genomes of pathogens helps scientists understand transmission patterns and evolution. A defining characteristic of this application is its ability to generate outbreak maps, revealing how infections spread geographically.
The unique feature of this approach is the timely response it facilitates in public health. For instance, during an outbreak of a disease like COVID-19, WGS can identify mutations in the virus, aiding in vaccine development and modification. However, challenges remain in terms of data interpretation and managing privacy concerns related to genomic data.
Research Implications
Evolutionary Studies
WGS has revolutionized our approach to evolutionary biology. By comparing the genomes of different organisms, scientists can identify evolutionary relationships and trace lineage. This specific aspect enhances our understanding of natural selection and genetic drift.
The key characteristic of evolutionary studies using WGS is the detailed insight into genetic changes over time. Such information aids in reconstructing evolutionary trees that illustrate relationships between species. However, the intricate data analysis required for accurate interpretations can often be a hurdle.
Population Genomics
Population genomics examines genetic diversity within and between populations. This field is crucial for understanding how evolutionary forces shape genetic variation. The main benefit of this application lies in its role in conservation genetics, as it informs strategies to protect endangered species.
A unique feature of population genomics is its capability to analyze vast amounts of genomic data from diverse individuals. This can lead to insights into allele frequency changes due to environmental pressures. Nonetheless, complexities like sample bias and data representativeness are challenges that require careful consideration.
Functional Genomics
Functional genomics investigates the role and function of genes in biological processes. The integration of WGS into this field has enabled researchers to identify gene functions and their interactions comprehensively. A notable characteristic is the dynamic understanding of how genes influence phenotypes under different conditions.
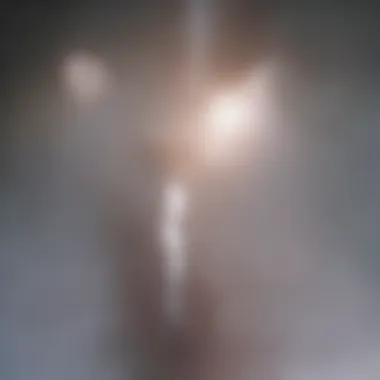
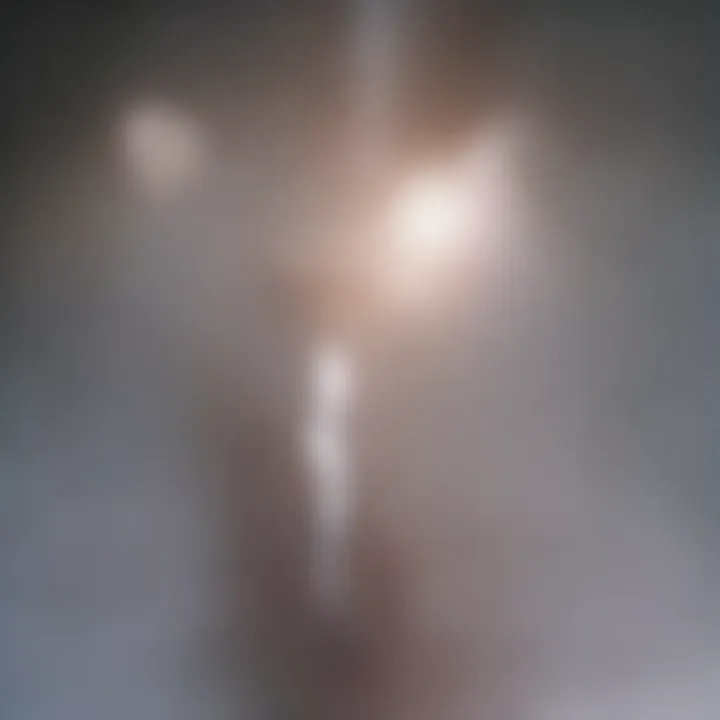
The unique aspect of functional genomics is its emphasis on gene expression analysis, helping to unravel the intricate networks that regulate biological systems. However, the integration of vast datasets and the technical expertise needed for analysis often pose problems for researchers.
In summary, the applications of whole genome sequencing extend profoundly into clinical and research settings, providing vital insights that influence medicine, public health, and biological understanding, despite the challenges posed by complexity and interpretation.
Ethical and Social Considerations
The significance of ethical and social considerations in whole genome sequencing cannot be overstated. As this technology advances and becomes more accessible, concerns arise regarding the implications for individual privacy, consent, and genetic ownership. Grounding these considerations in ethical practice is essential. Society as a whole must navigate the complex landscape that genomic data presents, balancing innovation and protection of individual rights.
Privacy and Data Security
Privacy regarding genomic data is paramount. DNA contains sensitive information about an individual’s health, ancestry, and predisposition to certain conditions. Given the potential for misuse, ensuring robust data security measures becomes crucial for institutions involved in sequencing.
Organizations must implement encryption technologies and strict access controls to protect this information. Patients should be informed about how their data will be used, stored, and shared. If not addressed, the risk of data breaches could lead to significant personal and societal consequences.
Key aspects regarding privacy and data security include:
- Informed consent: Individuals must understand what they are consenting to when providing their genomic data.
- Data anonymization: Efforts must be made to anonymize personal data to prevent identification.
- Regulations: Compliance with laws like the Health Insurance Portability and Accountability Act (HIPAA) is vital to ensure data protection.
"Genomic data must be treated with the same level of confidentiality as any other medical information."
Consent and Genetic Ownership
Consent in whole genome sequencing is another critical element. Individuals should have the right to determine how their genetic information is used. This ownership extends beyond initial consent and involves considerations about future research or commercial uses.
An effective consent process should:
- Provide clear information: Potential participants should receive detailed explanations of how their data will contribute to research, including potential risks.
- Allow withdrawal: Individuals should be able to withdraw their consent anytime, limiting the use of their data for future studies.
- Discuss benefits and risks: Understanding possible implications for personal and family health is crucial.
Genetic ownership raises further questions. Who truly owns the genetic data collected? Does the individual, the research institution, or the company developing the technology hold rights to this data? This ambiguity highlights the need for clearer guidelines and ethical standards in genomic research.
Overall, addressing these ethical and social considerations will ensure the progress of whole genome sequencing is aligned with the values of society.
Future Perspectives in Whole Genome Sequencing
The field of whole genome sequencing (WGS) is poised for significant advancements, impacting medicine, research, and our understanding of genetics. As technology evolves, so too does the potential for WGS to revolutionize various sectors. The focus on improvements in sequencing accuracy and the reduction of costs and time frames stands out. These factors are crucial for broadening access to genomic data and enhancing the capabilities in diverse applications, from healthcare to environmental studies.
Furthermore, as more data becomes available, the ethical considerations surrounding WGS will also be increasingly important. Understanding how innovations can improve healthcare delivery, societal aspects, and educational implications is vital.
Technological Innovations
Advancements in Sequencing Accuracy
One of the fundamental aspects of whole genome sequencing is advancements in sequencing accuracy. Over the past few years, improvements in technologies have led to more precise readings of genetic data. This accuracy is critical for a variety of applications, particularly in clinical settings where decisions regarding patient care depend on genetic information.
The key characteristic that makes these advancements noteworthy is the emergence of next-generation sequencing (NGS) platforms that improve both throughput and reliability. With these new capabilities, errors in SNP detection can be reduced significantly. This is a beneficial advancement for research and clinical applications alike.
A unique feature of these advancements includes the enhanced algorithms that minimize data interpretation errors. The advantages of increased accuracy translate to better diagnostics and more personalized treatments. However, there is a disadvantage when it comes to costs associated with these technologies, as cutting-edge options may still be prohibitively expensive for some institutions or laboratories.
Reduction in Costs and Timeframe
The reduction in costs and timeframe of whole genome sequencing is vital for its widespread adoption. Historically, the financial burden of sequencing the entire genome was a barrier for many researchers and institutions. Advances in technology have resulted in significant drops in costs, making sequencing more accessible.
Crucially, the key characteristic of this reduction is attributed to the development of high-throughput sequencing techniques that can process large volumes of data rapidly. This makes WGS not only more feasible for academic research but also for routine clinical diagnostics.
A unique feature in this area is the increased scalability of sequencing operations. This allows for more extensive studies, such as population genetics, to be conducted more efficiently. Advantages include making genomic technologies available to a larger audience. However, there are still challenges related to data storage and management as sequencing becomes cheaper and more data is generated.
Potential Impact on Medicine and Society
The potential impact of whole genome sequencing on medicine and society is profound. In medicine, WGS promises to simplify and enhance the implementation of personalized treatments. With precise information on individual genomes, healthcare providers can tailor therapies based on genetic profiles rather than relying solely on generalized treatments.
Moreover, the insights gained from population genomics can inform public health initiatives and assist in understanding disease patterns. On a societal level, WGS fosters genetic understanding that can lead to innovations across various sectors, including agriculture and environmental management.
"Whole genome sequencing is not just the future of medicine; it is a gateway to a deeper understanding of living systems."
The journey of whole genome sequencing is a testament to how technology can transform our capabilities and insights, echoing the importance of ongoing innovations in this fascinating field.
Epilogue
Whole genome sequencing is a transformative process in modern biological sciences, influencing several fields such as medicine, research, and ethics. This article has illustrated various aspects of whole genome sequencing, including the methodologies used, applications in clinical settings, and the ethical concerns surrounding genomic data.
Summary of Key Points
- Definition and Importance: Whole genome sequencing allows for the thorough examination of an organism's complete genetic makeup, providing insights crucial for individualized approaches in medicine.
- Technological Innovations: Advances in sequencing technology have drastically reduced costs and increased speed and accuracy, making genome sequencing accessible.
- Applications: The applications in personalized medicine, including its role in cancer treatment and infectious disease tracking, underline its clinical relevance.
- Ethical Considerations: Issues of privacy, consent, and genetic ownership are central to the discourse on genome sequencing, highlighting the need for responsible practices.
Final Thoughts on Whole Genome Sequencing
As whole genome sequencing continues to evolve, it holds the potential not only to advance medical practices but also to reshape our understanding of human biology. The implications of this technology are vast, extending beyond clinical applications to research and public health policy. Society must remain engaged in discussions surrounding data ethics and the integration of genomic information in healthcare. This engagement will ensure that the benefits of whole genome sequencing are realized while minimizing risks.
The future of healthcare may very well hinge on our ability to ethically and effectively integrate whole genome sequencing into routine practice.