Understanding Synthesis in Chemistry: Key Concepts and Applications
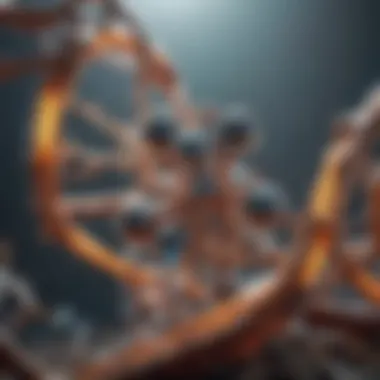
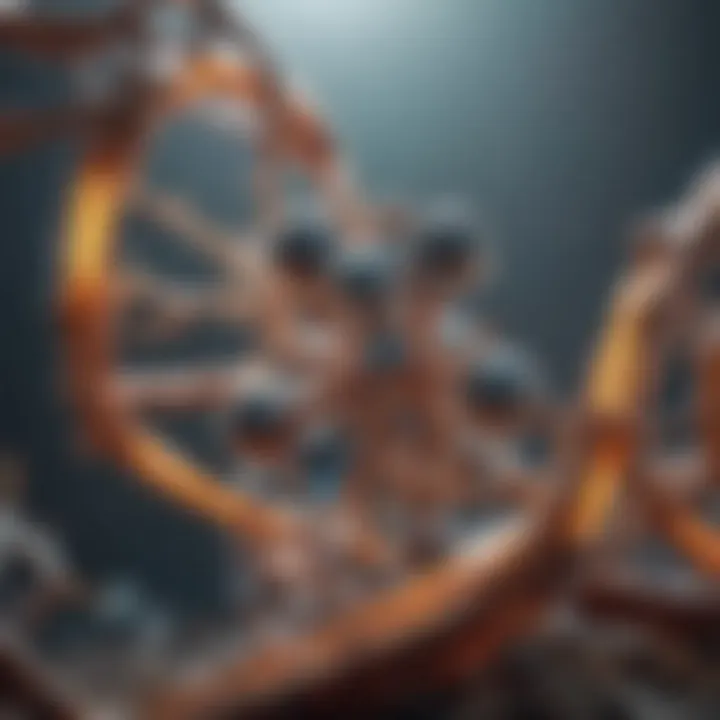
Intro
Synthesis is a fundamental process in chemistry, key to understanding how different substances can combine to form new compounds. It serves several purposes, from creating novel materials to developing effective pharmaceuticals. Chemical synthesis can be broadly categorized into two types: organic synthesis, which focuses on carbon-based compounds, and inorganic synthesis, dealing with a broader range of materials. Both are essential across various industries, each with unique methodologies that push the boundaries of scientific innovation.
The significance of synthesis extends beyond mere academic interest; it influences real-world applications and research. The ability to synthesize compounds efficiently can lead to breakthroughs, particularly in fields such as drug development and nanomaterials. This article will delve into the methodologies employed in both organic and inorganic synthesis, while exploring the tools and technologies that aid these processes.
Understanding synthesis also involves examining the theoretical frameworks that underpin these techniques. This knowledge is essential for anyone involved in research or industry, ensuring they are well-equipped to tackle the challenges and opportunities that arise in the field of chemistry.
Methodologies
Delving into the methodologies of chemical synthesis reveals a rich tapestry of techniques that researchers employ to create new substances. The choice of method often depends on the specific characteristics of the compounds in question and the desired outcomes.
Description of Research Techniques
In organic synthesis, several research techniques are prominent:
- Retrosynthetic analysis: This approach involves deconstructing complex molecules into simpler precursors. By understanding the pathways that lead to these molecules, chemists can plan efficient synthesis routes.
- Functional group transformations: These reactions involve converting one functional group into another, allowing for the construction of complex molecules from simpler ones.
- Total synthesis: This is the complete laboratory synthesis of complex organic molecules from simpler organic compounds. It often requires innovative strategies to overcome challenges.
In inorganic synthesis, techniques such as sol-gel processes, ion exchange, and solid-state synthesis are common. These methods facilitate the manipulation of inorganic compounds, which can have a wide range of applications from catalysis to materials science.
Tools and Technologies Used
The role of technology in synthesis cannot be overstated. Advanced instrumentation is critical for successful synthesis. Key tools include:
- Nuclear Magnetic Resonance (NMR): This technique provides detailed information about the structure of molecules, aiding in confirming the results of a synthesis.
- Mass Spectrometry (MS): This tool helps determine the mass-to-charge ratio of ions, offering insights into molecular weights and structures.
- High-Performance Liquid Chromatography (HPLC): HPLC separates, identifies, and quantifies components in a mixture, ensuring purity in synthesized compounds.
Innovations in automation and artificial intelligence are also changing the landscape of synthesis, enabling faster and more accurate reactions.
Discussion
Comparison with Previous Research
Recent advancements in synthesis illustrate a significant evolution from traditional methodologies.Historically, synthesis relied heavily on manual techniques and extensive trial and error. Today, automation and streamlined processes play a vital role in increasing efficiency and reproducibility. For instance, the advent of combinatorial chemistry has allowed researchers to rapidly create and screen large libraries of compounds, significantly speeding up the discovery phase in drug development.
Theoretical Implications
Theoretical frameworks in synthesis provide insights into reaction mechanisms and kinetics. Understanding these principles allows chemists to predict outcomes and optimize conditions for desired products. Advances in computational chemistry also enhance this understanding, allowing for simulations that inform laboratory practices and reduce resource consumption.
Synthesis is not just a method but an art that bridges scientific theory and practical application, fostering innovation across various sectors.
Preface to Synthesis in Chemistry
Synthesis in chemistry is a fundamental process that underpins much of the scientific exploration and innovation in this field. It plays a crucial role in not only understanding chemical compounds but also in creating new materials and pharmaceuticals. The significance of synthesis lies in its ability to transform simple substances into complex entities, a skill that has implications across various industries and research domains. As we explore this topic in detail, it becomes apparent that synthesis is not merely a technical exercise; it represents the very essence of creative problem solving in chemistry.
Definition of Synthesis
Synthesis, in the context of chemistry, refers to the process through which chemical compounds are formed. This can occur through various methods, including combining simpler reactants to produce more complex products. The terms "chemical synthesis" encapsulate both the methods used and the underlying chemical reactions involved. It is essential to recognize that synthesis can be broadly classified into organic and inorganic synthesis, each with its own unique set of methodologies and applications.
In organic chemistry, synthesis often involves the construction of carbon-based compounds, while inorganic synthesis covers the production of compounds that do not primarily involve carbon. Understanding the definition of synthesis is the first step toward appreciating its vast applications and significance in the advancement of modern chemistry.
Historical Context
The history of chemical synthesis is rich and marked by numerous pivotal moments that have shaped our current understanding. Early contributions date back to ancient alchemists, who, though lacking the scientific framework we have today, laid the groundwork for modern chemistry. They attempted the transformation of base metals into gold, unknowingly exploring concepts that would later be defined within the realm of chemical synthesis.
The advent of the 19th century marked a significant turning point. Chemists like Friedrich Wöhler demonstrated that organic compounds could be synthesized from inorganic substances, challenging the belief in the "vital force" theory, which posited that organic compounds could only be produced by living systems. This was a monumental moment in understanding synthesis as it opened new pathways for research and innovation.
In the 20th century, the pace of development accelerated with the invention of new synthetic techniques and better understanding of reaction mechanisms. Researchers began to explore not just how to synthesize compounds, but also why certain reactions occurred, leading to the birth of modern synthetic organic chemistry. Today, advancements such as automated synthesis and green chemistry are redefining the boundaries of what is possible in the field. Through this historical lens, one can appreciate how synthesis has evolved and continues to be a linchpin in various chemical investigations.
In summary, synthesis in chemistry transcends the mere combination of substances. It is a creative and intellectual pursuit that has driven significant advancements in science and technology.
Types of Chemical Synthesis
The topic of different types of chemical synthesis is crucial to understanding the broader field of synthesis in chemistry. Each type offers unique methodologies, applications, and challenges that define their significance in research and industrial settings. Organic synthesis and inorganic synthesis, the two main categories, showcase the diversity in techniques used to create desired compounds. Recognizing the distinctions between them is essential, as it informs researchers and practitioners on best practices, efficiency, and innovation.
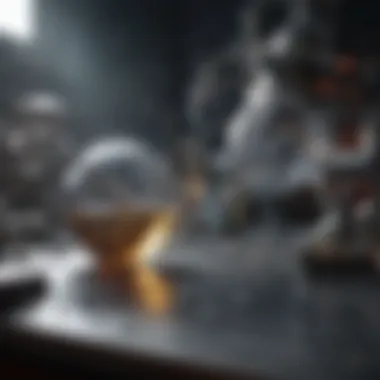
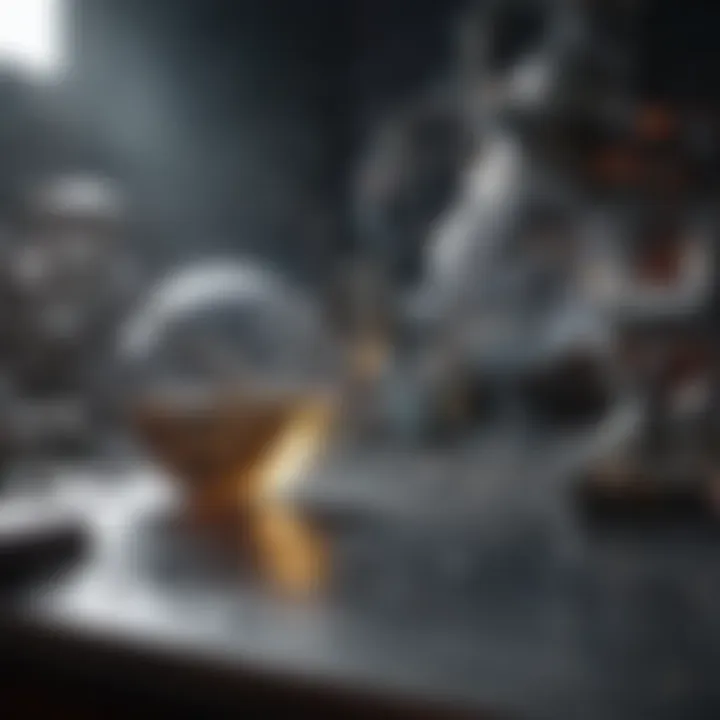
Organic Synthesis
Definition and Importance
Organic synthesis refers to the process of creating organic compounds through chemical reactions. These compounds often contain carbon and are foundational to pharmaceuticals, agrochemicals, and materials science. The importance of organic synthesis lies in its ability to produce complex molecules that serve various applications, including drug development. It is a key pathway to discovering new medications, enabling the design of molecules tailored to target specific biological mechanisms, thus improving therapeutic efficacy.
One key characteristic of organic synthesis is its versatility. It accommodates a broad array of methods, allowing chemists to construct complex structures. However, it often requires substantial time and resources, which can be a disadvantage when rapid production is necessary. Despite this, the benefits of organic synthesis in creating life-saving drugs cannot be overstated.
Common Methods
Organic synthesis encompasses several common methods, including reactions such as substitution, addition, and elimination. Each method provides distinct avenues for building compounds. The popularity of methods like the Grignard reaction and various coupling reactions stems from their efficiency in forming new carbon-carbon bonds.
A unique feature of these methods is their adaptability; they can be modified to accommodate different starting materials and desired products. However, this flexibility comes with challenges, especially related to reaction conditions and yields. Achieving optimal conditions can be tedious and requires significant expertise.
Synthetic Pathways
Synthetic pathways in organic synthesis map out the steps taken to create target compounds, often involving multiple reactions. Understanding these pathways is essential for chemists, as they help predict outcomes and optimize processes. The importance of synthetic pathways lies in their systematic breakdown of molecular construction, guiding chemists through intricate transformations.
A key characteristic of synthetic pathways is their strategic development. Chemists select pathways based on reaction conditions, expected yields, and available reagents. This foresight enhances efficiency and reduces waste, aligning with modern commitments to sustainable practices. However, the complexity of pathways may lead to unforeseen difficulties, often necessitating adjustments as the synthesis progresses.
Inorganic Synthesis
Overview and Techniques
Inorganic synthesis focuses on the creation of inorganic compounds, which include metals, minerals, and coordination complexes. Techniques used in inorganic synthesis vary widely, from solid-state reactions to hydrothermal synthesis. This diversity of approaches allows for the production of materials with tailored properties, essential for catalysis, electronics, and other advanced applications.
A key characteristic of inorganic synthesis is precision in controlling reaction conditions. This precision is vital to achieving the specific properties desired in the final product. While it can provide high-purity compounds, the intricate nature of some inorganic reactions may demand specialized equipment and environments, which could complicate scalability.
Applications of Inorganic Synthesis
The applications of inorganic synthesis are vast, spanning fields such as catalysis, materials science, and nanotechnology. For instance, metal oxides produced through inorganic synthesis have unique electronic properties that make them valuable in semiconductor technology. In addition, many inorganic compounds serve as catalysts in industrial processes, showcasing their practical importance.
One standout characteristic of inorganic synthesis is its impact on technology. The ability to design compounds at the molecular level creates opportunities for innovation. However, scaling these processes for industrial use often presents challenges. The costs associated with reagent purity and process optimization can be significant, underscoring the need for ongoing research to improve efficiency and sustainability in inorganic synthesis.
The Role of Synthesis in Chemical Research
Synthesis plays a crucial role in chemical research, acting as the backbone for developing new compounds and materials. Understanding the intricate processes that underlie the synthesis enables scientists to innovate, solve complex problems, and drive advancements in various fields. The significance of synthesis extends beyond mere laboratory practice; it is integral to the progression of chemical sciences, pharmaceuticals, and materials development.
One of the main benefits of synthesis in chemical research is the ability to create compounds that do not exist naturally. This capability allows for the exploration of new properties and functionalities, expanding the potential applications in real-world scenarios. As researchers refine synthetic methods, they improve efficiency and reduce waste, ultimately leading to more sustainable practices.
Additionally, the role of synthesis is evident in its collaborative nature, bringing together elements of different scientific disciplines. The increasing complexity of modern research requires an interdisciplinary approach, where synthesis connects fields such as biology and materials science. This interface fosters innovative applications and enhances scientific understanding.
Advancements in Synthetic Methods
Green Chemistry Approaches
Green chemistry approaches focus on designing chemical processes that minimize environmental impact. These methods are significant for addressing global concerns such as pollution and resource depletion. The hallmark of green chemistry is its commitment to sustainability, promoting the use of safer chemicals and reducing the generation of hazardous substances.
A key character of green chemistry is its emphasis on energy efficiency. By utilizing renewable resources, these approaches lower the consumption of energy during synthesis. This not only decreases operational costs but also contributes to environmental responsibility.
While green chemistry has many advantages, it can also present challenges. The selection of appropriate alternative solvents or reagents may require extensive research and development. Substituting well-established methods for greener options might initially lead to increased costs and uncertainties in scalability. However, the long-term benefits often outweigh these disadvantages, making it a valuable focus within chemical research.
Automated Synthesis Techniques
Automated synthesis techniques represent a significant breakthrough in chemical research. These methodologies leverage technology to streamline the synthesis process, allowing for higher throughput and reproducibility. Key characteristics include precision and the ability to manage complex procedures with minimal human intervention.
With automation, researchers can conduct multiple reactions simultaneously. This results in a faster development cycle for new compounds and materials. Additionally, automation enhances the accuracy of experiments, which is vital for generating reliable data.
However, automated techniques can come with a downside. The initial setup costs may be prohibitive for some laboratories, especially smaller ones. There is also a learning curve involved with integrating new technologies into established workflows. Despite these challenges, the advantages offered by automated synthesis techniques often justify the investment, making them a significant aspect of modern synthesis research.
Cross-Disciplinary Applications
Relationship with Biology
The relationship between synthesis and biology is increasingly recognized as a powerful synergy in chemical research. This connection enables the development of pharmaceuticals and biomaterials that directly impact health care and medical treatments.
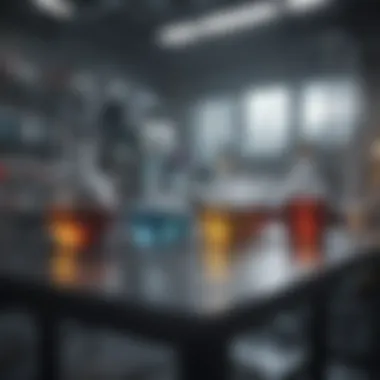
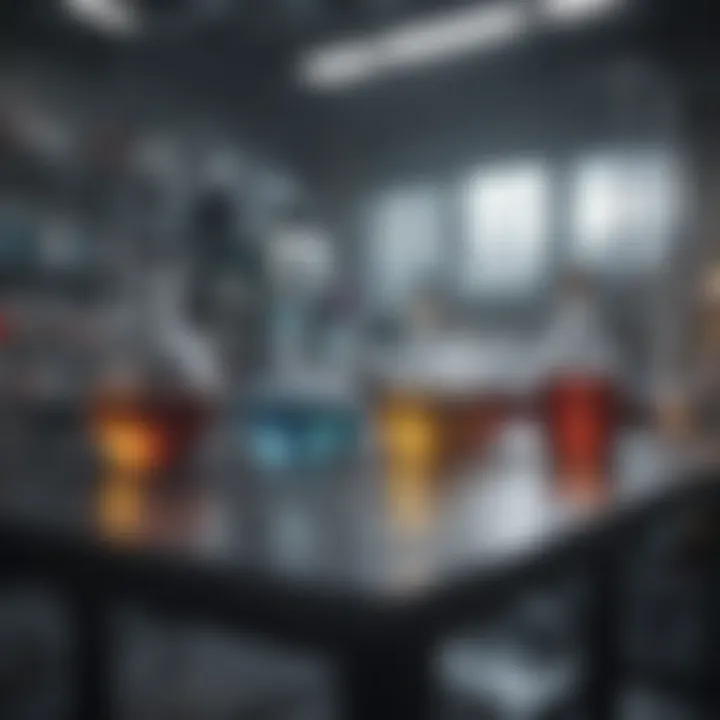
A key characteristic of this relationship is the use of synthetic pathways to create biologically active compounds. These can range from simple drug molecules to complex biomolecules for research purposes. This adaptability makes synthesis an essential tool in drug discovery and development, enhancing its relevance in this article.
Although the collaboration between synthesis and biology offers many benefits, such as more targeted therapies, it also presents certain hurdles. The natural complexity of biological systems can complicate the translation of synthetic compounds into effective treatments. Rigorously testing these compounds is vital to ensure both efficacy and safety before clinical applications.
Interactions with Materials Science
Interactions between synthesis and materials science create exciting opportunities for innovation. Advances in synthetic methods lead to the design of new materials with tailored properties, essential for various applications including electronics, energy storage, and nanotechnology.
The key characteristic of these interactions lies in the ability to manipulate materials at the molecular level. This opens pathways for creating advanced materials that can meet specific demands in technology and industry. Such versatility has solidified synthesis's role as a pivotal factor in materials science developments.
Nonetheless, the challenge remains in the scalability of such materials. Transitioning from laboratory-scale synthesis to larger production systems can pose difficulties. This often results in increased costs and potential compromises in material properties. Despite these issues, the ongoing exploration of these interactions highlights the promise of synthesis in shaping the future of materials science.
Key Techniques in Synthesis
Key techniques in synthesis are foundational to the practice of chemistry. Understanding these methods enables chemists to develop new compounds efficiently. Techniques assist in creating various products that serve diverse functions across industries. Familiarity with these techniques impacts research and development significantly. The focus on reagents, catalysts, and reaction mechanisms highlights their roles in optimization of synthetic strategies.
Reagents and Catalysts
The Role of Catalysts
Catalysts play a crucial role in enhancing the efficiency of chemical reactions. They accelerate reactions without undergoing permanent change themselves. This characteristic makes them essential in multiple types of synthesis. Catalysts lower the activation energy required, thus improving reaction rates substantially. In the context of this article, their importance cannot be overstated.
A unique feature of catalysts is their ability to facilitate reactions under milder conditions. This lowers the risk of side reactions and helps produce higher yields. Moreover, the use of catalysts can lead to significant economic advantages by reducing resource consumption. However, catalysts also require careful selection as factors such as selectivity and availability must be considered.
Selection of Reagents
The selection of reagents is equally critical in the synthesis process. Reactants determine the pathways and the efficiency of chemical syntheses. Proper selection leads to higher yields and purer products, which is pivotal in chemistry. In this article, we emphasize the importance of choosing reagents wisely.
A key characteristic of reagents is their reactivity and stability. The unique feature of some reagents is their ability to engage in multiple reaction types. This versatility can be beneficial for chemists looking to streamline synthetic procedures. However, the selection of inappropriate reagents may lead to unwanted by-products or reaction failures, impacting overall success.
Reaction Mechanisms
Key Mechanisms in Organic Chemistry
Understanding key mechanisms in organic chemistry is vital for chemists. These mechanisms define how reactions proceed at the molecular level. They provide insight into the relationship between structure and reactivity. In this article, we explore mechanisms that contribute significantly to synthetic pathways.
The importance of these mechanisms lies in their ability to predict reaction outcomes. By understanding how different reagents interact, chemists can devise more efficient synthetic strategies. A notable advantage of mastering these mechanisms is facilitating rational design of new compounds. However, complexities in certain reaction pathways may pose challenges in predicting outcomes accurately.
Mechanisms in Inorganic Reactions
Inorganic reactions also possess distinct mechanisms that guide synthesis. These mechanisms focus on transitions between different oxidation states or coordination environments. The exploration of these mechanisms enriches our overarching understanding of chemical synthesis. They contribute to diverse applications, especially in materials science.
One key characteristic of these mechanisms is their diverse nature. They can involve phenomena such as ligand exchange and electron transfer. Their unique interaction patterns can assist in designing novel inorganic materials. However, the complexity of inorganic mechanisms can sometimes make generalization difficult, which may hinder advancements in specific areas.
Applications of Synthesis
Synthesis plays a pivotal role in many branches of chemistry. It allows chemists to create a wide variety of compounds that have significant applications in various fields. The process of synthesis not only contributes to academic knowledge but also enhances practical solutions to real-world problems. In this section, the focus is on two crucial applications: pharmaceutical synthesis and materials synthesis.
Pharmaceutical Synthesis
Development of New Drugs
Pharmaceutical synthesis is fundamental for the development of new drugs. This area focuses on the creation of novel chemical entities that can function effectively as medicinal treatments. The key characteristic of drug development lies in the ability to modify chemical structures to enhance efficacy or reduce side effects. It is a central theme in this article because it illustrates the direct impact of synthetic chemistry on health and well-being.
The unique feature of drug development is its iterative nature. Chemists often synthesize multiple variants of a drug to optimize its properties. The advantages include the potential for discovering new treatments for various diseases. However, challenges include lengthy development times and high costs associated with thorough testing and regulatory approval.
Role in Drug Discovery
The role of synthesis in drug discovery cannot be overstated. Synthesis provides the necessary compounds that researchers test for biological activity. This direct contribution aids in identifying candidates that can become effective therapeutic agents. A noteworthy aspect of this role is the utilization of structure-activity relationship studies to guide the design of new compounds.
One key characteristic is the collaboration between chemists and biologists. This teamwork enhances the efficacy of the discovery process. The advantage is a more targeted approach to identifying potential drugs. However, there are risks, including the possibility of compounds failing during clinical trials, which can lead to wasted resources.
Materials Synthesis
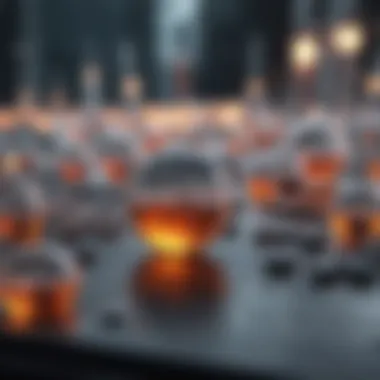
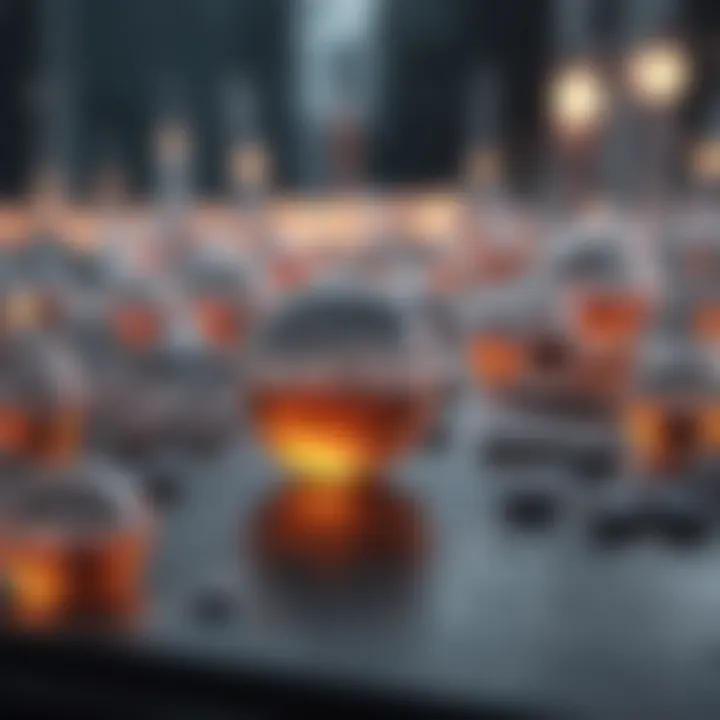
Nanomaterials
Nanomaterials have gained significant attention due to their unique properties and potential applications. The synthesis of nanomaterials allows for the creation of materials at a molecular scale, resulting in substances that can exhibit enhanced strength, electrical conductivity, or chemical reactivity. This area is particularly beneficial because it drives innovation in fields like electronics and energy storage.
A noteworthy characteristic of nanomaterials is their large surface area relative to volume, which can lead to improved performance in various applications. The advantages include advancements in technology and a reduction in material usage. However, some challenges involve the complexities of synthesis and potential environmental impacts.
Polymers and Their Applications
The synthesis of polymers involves creating long chains of molecules that can have diverse applications. From plastics to biocompatible materials, polymers are everywhere in modern life. Their significance in this article is underscored by their versatility and the wide range of functional properties they can possess.
A key characteristic of polymers is their ability to be tailored for specific properties through synthesis techniques. This customization allows for new applications in technology, healthcare, and more. The advantage of focusing on polymers is their ubiquitous nature and relevance in everyday products. However, the challenges often include issues related to biodegradability and environmental impact.
Challenges in Chemical Synthesis
Chemical synthesis plays a critical role in the advancement of science. However, numerous challenges arise during the synthesis process. Addressing these challenges is essential for enhancing the efficiency and effectiveness of chemical reactions. Difficulties such as scalability, cost, safety, and environmental impact must be considered. Understanding these challenges fosters innovation and leads to better methodologies. This section examines key challenges in chemical synthesis, including scalability issues and safety concerns.
Scalability Issues
From Laboratory to Industry
One major challenge in chemical synthesis is the transition from laboratory-scale experiments to industrial-scale production. This process, often termed scale-up, involves numerous factors. A critical aspect is maintaining efficiency and yield when increasing the volume of reagent. The unique characteristic of scaling from laboratory to industry is often non-linear. Small-scale reactions may not easily translate to larger volumes. Poor scalability can lead to decreased yields and increased production costs. Therefore, understanding these differences is vital to ensuring successful synthesis in an industrial setting.
Cost Efficiency
Cost efficiency is another significant aspect of chemical synthesis. In a competitive market, reducing costs without compromising quality is crucial. Cost efficiency involves selecting appropriate reagents, optimizing reaction conditions, and minimizing waste. A benefit of high cost efficiency is the potential for increased profitability. However, achieving cost efficiency can sometimes lead to trade-offs. For instance, using less expensive reagents may not always deliver the desired reaction yields. Thus, balancing robustness, scalability, and cost remains a constant challenge for chemists.
Safety and Environmental Concerns
The safety and environmental issues associated with chemical synthesis cannot be overlooked. Synthesizing chemicals often involves hazardous materials and reagents. Proper safety protocols must be established to protect researchers and the environment. Furthermore, the environmental impact of synthetic processes should be evaluated. Innovative approaches are being developed to mitigate waste and reduce the carbon footprint of chemical synthesis. The integration of green chemistry principles is becoming fundamental. This involves developing processes that are both safe and sustainable, ensuring that advancements in synthesis also consider their broader impacts.
Future Directions in Synthesis
The future of synthesis in chemistry holds significant promise in addressing modern challenges and advancing scientific fields. As industries and researchers seek more efficient and environmentally friendly methodologies, the focus is increasingly on sustainable practices and innovative technologies. Emphasizing future directions not only highlights the potential progress in synthesis but also aligns with current global trends that prioritize green chemistry and automation.
Emerging Trends
Sustainable Synthesis
Sustainable synthesis represents a critical shift in chemical processes. This approach aims to minimize environmental impact while maximizing resource efficiency. Key characteristics of sustainable synthesis include the use of renewable feedstocks, energy-efficient reactions, and waste reduction. This method is beneficial as it reduces ecological footprints in chemical production.
One unique feature of sustainable synthesis is the incorporation of alternative solvents, such as water or biodegradable solvents. These choices often lead to safer and more environmentally friendly processes. However, challenges such as the scalability of these methods can exist.
Integration with Artificial Intelligence
The integration of artificial intelligence (AI) into synthesis marks a revolutionary advance. AI technologies assist in predicting reaction outcomes and optimizing conditions. The key characteristic of AI integration in synthesis is its capacity for predictive analytics, allowing researchers to simulate reactions before physically conducting them. This aspect makes it a popular choice in contemporary research.
One unique feature of AI integration is the development of machine learning algorithms that continuously improve through data analysis. Its advantages include accelerated research timelines and enhanced safety through risk reduction. But, the reliance on data quality and availability is a significant challenge.
Innovative Collaborative Efforts
Looking ahead, innovative collaborations between academia and industry play a pivotal role in sustaining growth in synthesis. By combining resources, knowledge, and technology, these partnerships can pioneer new methodologies and unlock potential applications. Emphasizing teamwork between diverse fields such as biology, materials science, and engineering will enhance the possibilities within synthesis. These efforts align with global trends and showcase how collaboration can propel scientific advancement forward.
End
The conclusion serves as a critical component of the discussion on synthesis in chemistry. It transcends merely summarizing the sections to encapsulate the essence of the entire exploration. At its heart, the conclusion reinforces the significance of synthesis, highlighting its pervasive impact across various domains of science and industry.
Synthesis in chemistry isn't only a foundational concept; it represents the bridge between raw materials and functional products. Whether exploring medicinal applications or innovative materials, the ability to design and execute synthetic pathways dictates the advancement of technology and science. Understanding this connectivity allows for better exploration of how different segments of science interact and influence one another.
Summary of Key Points
In this article, we've dissected synthesis in chemistry through several lenses. The main points include:
- Definition of Synthesis: Chemical synthesis is the process through which complex compounds are formed from simpler substances, underscoring its fundamental role in both organic and inorganic chemistry.
- Types of Chemical Synthesis: We explored organic synthesis, which is vital in pharmaceuticals, and inorganic synthesis, with applications in various technologies and materials.
- Techniques and Challenges: The techniques discussed include the use of reagents and catalysts, reaction mechanisms, and the challenges of scalability and safety in chemical synthesis.
- Future Directions: Emerging trends, such as sustainable synthesis and the integration of artificial intelligence, were highlighted as pivotal to the evolution of the field.
Implications for Future Research
The implications for future research in synthesis are profound. As the demand for innovative solutions grows, synthesis will likely foster advancements in various sectors, including healthcare, clean energy, and materials science.
Investigating more sustainable synthesis methods could greatly enhance environmental preservation while maintaining efficiency. Furthermore, the incorporation of artificial intelligence into synthetic methodologies presents a transformative approach. This integration can facilitate predictive modeling, enabling chemists to foresee the outcomes of chemical reactions with greater accuracy.
In summary, synthesis in chemistry is more than a topic of study; it is a catalyst for innovation and advancement across multiple scientific fields. The possibilities for future research are not only exciting but essential for meeting the challenges of tomorrow.