Understanding RNA Sequencing: A Comprehensive Exploration
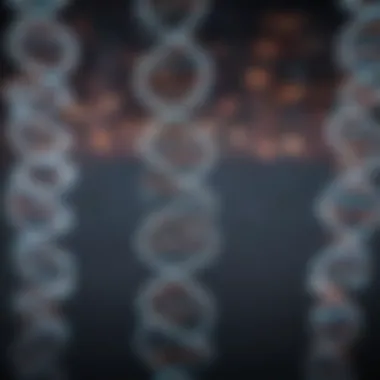
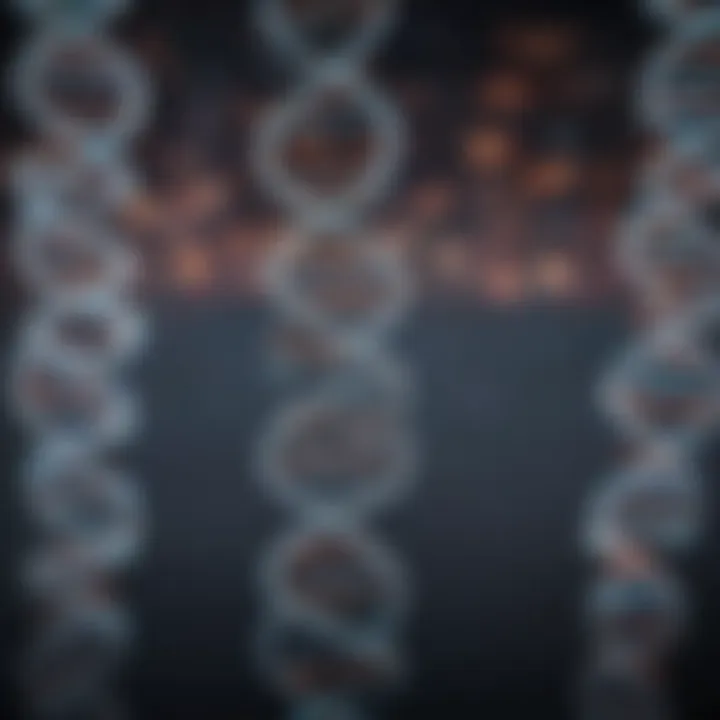
Intro
RNA sequencing, or RNA-seq, is revolutionizing the field of genomics and molecular biology. This technique enables researchers to gain insights into the entire transcriptome of an organism, allowing them to explore gene expression levels, identify various RNA transcripts, and even discover new RNA molecules. Therefore, understanding the methodologies and applications of RNA-seq is crucial for students, researchers, educators, and professionals in the life sciences.
This article aims to comprehensively explore RNA sequencing, discussing its principles and methodologies while illuminating its applications in various biological contexts. Furthermore, we will consider the strengths and limitations of RNA-seq, and address emerging trends that impact its future in scientific research.
In the following sections, we will delve into the methodologies employed in RNA sequencing, examining the tools and technologies that underpin this powerful technique.
Prolusion to RNA Sequencing
RNA sequencing, often referred to as RNA-seq, has revolutionized the way researchers analyze genetic material. This technique provides a comprehensive view of the transcriptome, which encompasses all RNA molecules in a cell. Understanding RNA sequencing is crucial for various reasons.
Firstly, it enables the identification of different RNA species and their expression levels. By quantifying gene expression, scientists can gain insights into how genes regulate biological processes. This capability is essential not just in basic research, but also in clinical settings where identifying gene behavior can lead to better diagnosis and treatment of diseases.
Furthermore, RNA-seq allows for the detection of alternative splicing events, which contribute to protein diversity. This is particularly important in understanding complex diseases like cancer, where altered splicing can play a critical role.
Moreover, with the increase in availability and affordability of sequencing technologies, RNA-seq is becoming a standard approach in genomics. It holds great potential for personalized medicine, where treatments can be tailored based on individual genetic profiles.
As RNA sequencing continues to evolve, it will undoubtedly shape our understanding of genetics, biology, and medicine. The following sections will delve deeper into its definition, purpose, and historical significance.
The Basics of RNA Biology
Understanding the fundamentals of RNA biology is critical when exploring RNA sequencing. This section elucidates the various types of RNA and their respective roles in biological processes. Each type serves distinct functions that contribute to cellular mechanisms and gene expression, all vital when interpreting data from RNA sequencing. Recognizing these elements enables researchers to make sense of sequencing results and their implications.
Types of RNA
RNA is not a uniform molecule; it exists in several forms, each fulfilling unique roles in the cell. Here, we will delve into the four main types: messenger RNA (mRNA), transfer RNA (tRNA), ribosomal RNA (rRNA), and non-coding RNA (ncRNA).
Messenger RNA (mRNA)
Messenger RNA is crucial as it serves as the template for protein synthesis. It facilitates the conversion of genetic information from DNA into proteins, which are essential for various cellular functions. The key characteristic of mRNA is its ability to carry instructions from the nucleus to ribosomes in the cytoplasm.
This characteristic makes mRNA a beneficial focus for RNA sequencing studies. It represents the genes that are actively expressed in a cell at a given time, providing insights into cellular activity. However, mRNA has a relatively short lifespan and can be prone to degradation, which can influence the reliability of quantification in sequencing results.
Transfer RNA (tRNA)
Transfer RNA interprets the mRNA sequence and brings the corresponding amino acids to the ribosome during protein synthesis. Each tRNA molecule is specific to an amino acid and has a unique anticodon that pairs with the codon on the mRNA. This precise interaction is vital for accurate translation of genetic information into proteins.
The key feature of tRNA is its role in matching amino acids with codons, which is critical for forming polypeptide chains. While tRNA may not be the primary focus of RNA sequencing, its understanding is essential for comprehending the translation process and the overall protein synthesis landscape.
One limitation is that tRNA molecules are less abundant than mRNA, making their detection more challenging in RNA sequencing.
Ribosomal RNA (rRNA)
Ribosomal RNA is a pivotal component of ribosomes, where proteins are synthesized. It ensures the proper alignment of mRNA and tRNA during translation. The most significant aspect of rRNA is its structural and functional role in the ribosomal machinery, which is essential for translating the genetic code.
Ribosomal RNA is advantageous for RNA sequencing because it is abundant in cells, providing a steady reference for normalization in quantitative studies. However, rRNA sequences are relatively conserved, which may limit the discovery of novel RNA species in sequencing data.
Non-coding RNA (ncRNA)
Non-coding RNA encompasses various types of RNA that do not code for proteins. These include microRNAs (miRNAs), long non-coding RNAs (lncRNAs), and small interfering RNAs (siRNAs). Non-coding RNAs play critical regulatory roles in gene expression, influencing various processes, such as development and response to environmental stimuli.
The key role of non-coding RNA in regulating gene expression makes it a powerful component in RNA sequencing analysis. They serve as important markers for biological processes and disease states. The challenge lies in their complexity and variability; their diverse functions may complicate interpretation and analysis, but uncovering these functions can lead to greater insights in RNA research.
Role of RNA in Gene Expression
RNA plays a central role in gene expression, effectively linking genetic information stored in DNA to protein synthesis. This process involves transcription, where RNA polymerase synthesizes RNA from a DNA template, and translation, where ribosomes interpret mRNA to produce proteins. Each type of RNA contributes to this complex network, highlighting the importance of understanding RNA biology when investigating gene expression through RNA sequencing.
"The vast array of RNA types and their specific roles underscore the complexity and dynamism of gene expression."
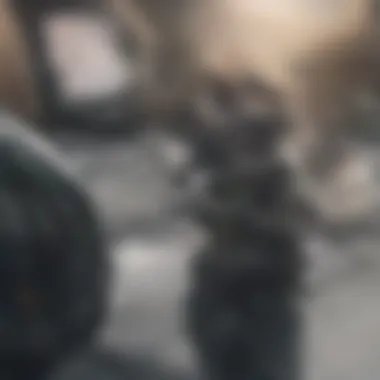
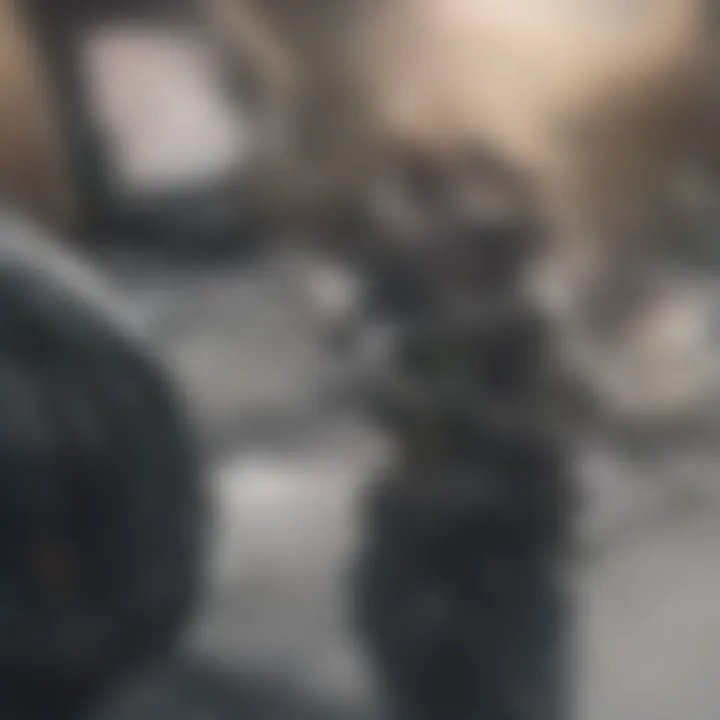
In summary, grasping the basics of RNA biology is essential to accurately interpret and analyze RNA sequencing results. The distinct features and functions of various RNA types not only inform research strategies but also enhance our understanding of the intricate biology underlying transcriptomes.
RNA Sequencing Techniques
RNA sequencing is a critical aspect of transcriptomic studies. It encompasses various methodologies that allow for a comprehensive analysis of RNA within a given sample. Each technique brings unique advantages and challenges, impacting data quality, resolution, and the scope of information gathered. The importance of RNA sequencing techniques lies in their ability to provide insights into gene expression, transcript variability, and the overall complexity of cellular mechanisms. Understanding these techniques is essential for researchers aiming to integrate RNA sequencing into their work.
Library Preparation
RNA Extraction and Purification
RNA extraction and purification is the first crucial step in library preparation. This process directly affects the integrity and quality of RNA, which are vital for downstream applications in RNA sequencing. The key characteristic of this step is its ability to isolate high-quality RNA while removing contaminants such as proteins and genomic DNA. As a result, it becomes a beneficial choice for generating reliable sequencing data. The most common methods include silica-based columns and phenol-chloroform extraction, each with its unique features. Silica columns offer simplicity and rapid processing, whereas phenol-chloroform can yield higher purity but may be more complex. The disadvantages can involve RNA degradation if not handled properly during extraction.
cDNA Synthesis
cDNA synthesis is a pivotal step in converting RNA into complementary DNA, a more stable form suitable for amplification and sequencing. The primary advantage of this step is that it allows researchers to work with a more stable molecule that can be efficiently amplified. The most recognized methodology involves the use of reverse transcriptase, which synthesizes cDNA from RNA templates. A unique feature of this process is its ability to select for mature mRNA by targeting poly-A tails, ensuring that irrelevant non-coding RNA is minimized. However, a disadvantage could be the introduction of biases during amplification, which may affect the quantification of gene expression levels.
Fragmentation and Adapter Ligation
Fragmentation and adapter ligation are crucial for preparing the cDNA libraries for sequencing. Fragmentation breaks the cDNA into smaller pieces, allowing for more effective sequencing. The characteristic of this step is the introduction of specific adapter sequences that facilitate subsequent amplification and sequencing processes. Adapter ligation enhances the ability to attach primers necessary for sequencing, making it a beneficial step in producing a translatable library. However, an over-fragmentation can result in loss of useful sequences, and improper adapter ligation can affect library complexity.
Sequencing Platforms
Sanger Sequencing
Sanger sequencing, one of the first sequencing techniques developed, remains relevant today for its accuracy and reliability. It involves chain termination methods to sequence individual fragments of DNA. This method allows precise analysis of targeted regions of interest, which is valuable for confirming variations identified by other techniques. A unique feature of Sanger sequencing is its high fidelity, making it suitable for validating results from high-throughput sequencing technologies. However, the primary drawback is its limited throughput and higher cost relative to more modern methods.
Next-Generation Sequencing (NGS)
Next-generation sequencing has fundamentally changed genomic research by allowing massively parallel sequencing of millions of fragments at once. This platform provides a comprehensive overview of transcriptomic landscapes, making it an essential tool for researchers. A key characteristic of NGS is its ability to produce large volumes of data in a relatively short time. The unique feature here is the advancement of multiplexing, which efficiently captures data from multiple samples. Nonetheless, NGS can be expensive and requires complex data analysis pipelines, which can be a barrier for some laboratories.
Long-Read Sequencing
Long-read sequencing technologies have emerged to overcome limitations posed by short-read approaches. They can accurately sequence longer strands of DNA or RNA, providing more extensive information about complex genes and genomic rearrangements. The important aspect of long-read sequencing is its ability to resolve structural variants and give a clearer picture of transcript isoforms. A unique feature is the capacity to span repetitive regions and handle full-length transcript analysis. However, the primary challenge includes higher error rates relative to short-read technologies, which necessitates careful validation of results.
Data Analysis and Interpretation
Quality Control
Quality control is vital to ensure the reliability of RNA sequencing data. This process involves evaluating raw sequencing reads to identify and filter out low-quality sequences that can distort analysis outcomes. A key characteristic of quality control is the use of various metrics to assess sequencing quality, such as read length and base quality scores. It is essential for maintaining the integrity of data. The unique feature is the implementation of software tools that automate quality checks, allowing for efficient data processing. However, it can be time-consuming and may require significant computational resources.
Alignment and Quantification
Alignment and quantification are crucial for interpreting RNA sequencing data. This involves aligning sequenced data to a reference genome to identify where transcripts originate. A key characteristic is the use of sophisticated algorithms that improve accuracy in matching reads to the reference. This becomes essential for quantifying gene expression levels across samples. The unique feature of modern alignment software is its ability to handle splicing events and multimapping reads. Disadvantages may include computational complexity and that misalignment may lead to errors in quantification.
Statistical Methods
Statistical methods in RNA sequencing data analysis are imperative for identifying significant patterns and differences between samples. Techniques such as differential expression analysis allow researchers to determine which genes are upregulated or downregulated under various conditions. A key characteristic of these methods is their ability to control for multiple testing errors. They often employ sophisticated statistical techniques to ensure robustness. A unique feature is the integration of Bayesian approaches, which can improve the interpretability of results. However, statistical approaches can be complex and require a sound understanding of statistical principles.
Applications of RNA Sequencing
RNA sequencing is essential in modern biological research. The versatility of RNA-seq allows scientists to explore various aspects of gene expression and transcriptomic diversity. Understanding these applications gives insights into RNA sequencing's significant role in advancing our comprehension of biology and disease.
Gene Expression Profiling
Gene expression profiling using RNA sequencing provides a comprehensive view of which genes are actively expressed in a cell or tissue at a given time. This technique reveals not only the presence of messenger RNA but also the level of expression. It provides a quantitative measurement of RNA levels, which can be essential for understanding cellular responses to external stimuli or developmental changes.
Key benefits of gene expression profiling include:
- Identification of Biomarkers: RNA-seq helps in discovering biomarkers for diseases, allowing for early detection and better prognosis.
- Comparative Analysis: Researchers can compare gene expression profiles under different conditions, aiding in drug response studies and understanding pathways that lead to diseases.
- Comprehensive Data: Unlike other methods like microarrays, RNA-seq can capture both known and novel transcripts, allowing for a broader analysis.
Detection of Alternative Splicing
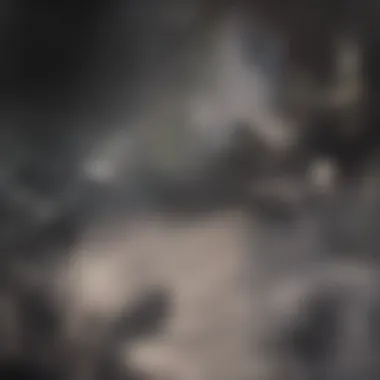
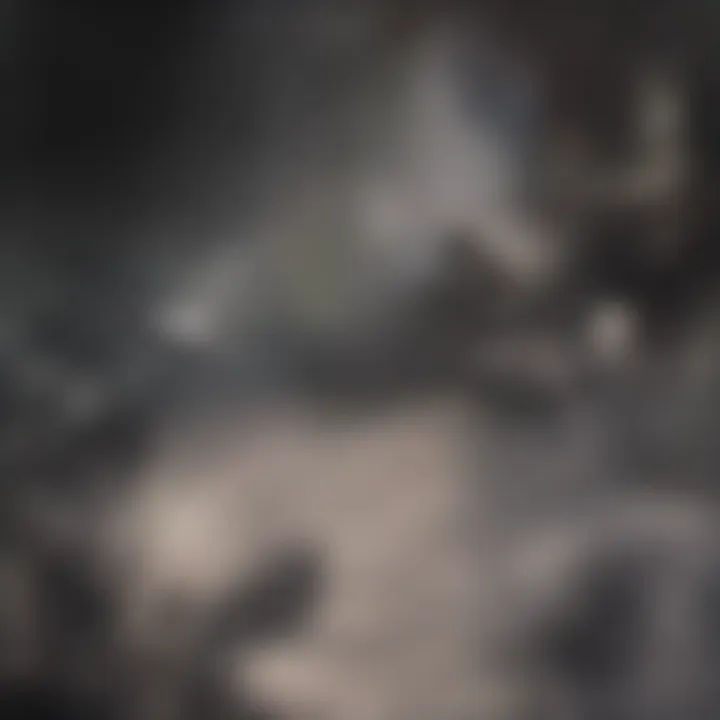
Alternative splicing is a process that generates multiple protein isoforms from a single gene. RNA sequencing plays a critical role in detecting these splicing variants. By analyzing the sequencing data, researchers can identify which isoforms are expressed in different conditions.
The significance of detecting alternative splicing includes:
- Understanding Complexity: It provides insights into the complexity of gene regulation and the functional diversity of proteins.
- Disease Associations: Many diseases are linked to aberrant splicing events. Identifying these changes can lead to better insights into mechanisms of diseases like cancer.
- Therapeutic Targets: Understanding splicing variants may reveal novel targets for therapeutic intervention, especially in targeted therapies.
Identification of Novel Transcripts
RNA sequencing allows for the discovery of previously uncharacterized transcripts. This includes novel lncRNAs and other non-coding RNAs that were not detected in earlier studies. The possibility of identifying new transcripts adds depth to our understanding of functional genomics.
Key points regarding novel transcript identification include:
- Enhanced Annotation: Expanding the gene annotation database helps in improving the existing genomic maps.
- Functional Studies: New transcripts may play important roles in cellular processes. Thus, they provide new avenues for research into gene function.
- Evolutionary Insights: Studying novel transcripts across different species can offer evolutionary insights into genome evolution and adaptation.
Single-Cell RNA Sequencing
Single-cell RNA sequencing (scRNA-seq) takes RNA sequencing a step further by allowing analysis at the single-cell level. This method uncovers cellular heterogeneity within a tissue, essential for understanding complex biological systems.
The implications of scRNA-seq include:
- Understanding Cellular Diversity: scRNA-seq can identify distinct cell populations, giving insights into how different cells within a tissue contribute to overall function.
- Developmental Biology: This approach is crucial in studying developmental processes, as it allows researchers to track cellular changes over time.
- Tumor Microenvironment: In cancer research, scRNA-seq aids in analyzing the tumor microenvironment, helping understand tumor progression and response to therapies.
RNA sequencing applications redefine our understanding of gene expression, alternative splicing, novel transcript identification, and cellular heterogeneity. These tools are key to unraveling the complexities of biological systems.
Importance of RNA Sequencing in Research
RNA sequencing plays a pivotal role in modern biological research. It allows for the examination of the entire transcriptome, which includes the RNA molecules expressed in a particular cell or tissue type at any given time. This capability elucidates how genes are expressed and how their expression differs among various conditions, such as during disease states or developmental stages. The insights gained from RNA sequencing not only advance our understanding of biological processes but also provide critical information that can lead to new diagnostic and therapeutic strategies.
Contributions to Understanding Diseases
Cancer Research
Cancer research significantly benefits from RNA sequencing. This technique enables researchers to identify differentially expressed genes in tumors compared to normal tissues. By analyzing these gene expression patterns, scientists can uncover molecular mechanisms responsible for cancer progression. A key characteristic of cancer research is its focus on tumor heterogeneity, which is often masked in bulk tissue analyses. RNA sequencing highlights the presence of subpopulations of cells that may have distinct gene expression signatures, providing insights into cancer evolution and treatment resistance. The unique feature of cancer research using RNA sequencing is its ability to discover potential biomarkers for targeted therapies, which could enhance the specificity of treatments offered to patients.
Neurological Disorders
RNA sequencing is also instrumental in the study of neurological disorders. These conditions, such as Alzheimer's and Parkinson's diseases, have complex molecular pathology that is not fully understood. Through RNA sequencing, researchers can profile gene expression changes in brain tissues affected by these disorders. A notable feature of this area is the potential for identifying novel therapeutic targets. This approach allows scientists to investigate how specific RNA molecules are involved in disease mechanisms. However, one should note that interpreting the data from neural tissues can be challenging due to the intricate network of gene interactions in the brain.
Genetic Diseases
Genetic diseases, caused by mutations in specific genes, represent another crucial area where RNA sequencing provides insights. By assessing gene expression in patients with genetic conditions, researchers can determine how these mutations impact transcript levels, leading to a better understanding of the disease pathology. The beneficial aspect of studying genetic diseases through RNA-seq is its potential for discovering new gene functions. This could lead to novel treatment options or gene therapies. However, the limitations include the complexity of analyzing large volumes of data, which can sometimes lead to ambiguous conclusions about the relationship between gene expression and disease phenotypes.
Role in Drug Development
RNA sequencing is increasingly utilized in drug development processes. It helps researchers understand how drugs affect the transcriptome of target cells. By observing changes in gene expression caused by therapeutic agents, scientists can identify pathways that are modulated by these treatments. This information is essential for fine-tuning drug design and optimizing therapeutic efficacy. Furthermore, RNA sequencing can support the identification of biomarkers that predict drug response, thus enabling personalized medicine approaches. The integration of RNA sequencing in drug development reflects its profound impact on enhancing the precision and effectiveness of therapeutic strategies.
Challenges and Limitations of RNA Sequencing
RNA sequencing, while an invaluable tool in modern biology, is not without its hardships. An awareness of these limitations enhances interpretation and application of RNA-seq results. Understanding these challenges is important to maximize the utility of the technology in research.
Technical Limitations
Bias in Library Preparation
Bias during library preparation can significantly influence the final data generated from RNA sequencing. This refers to the skewed representation of RNA molecules, often favoring more abundant transcripts over rare ones. Such bias occurs from several stages, including selection of RNA, reverse transcription to cDNA, and the amplification of the library. This characteristic makes bias a crucial point of discussion in RNA sequencing.
Key Characteristics: The main feature of bias is the difficulty of achieving uniform representation across diverse RNA populations. Many conventional protocols may amplify particular transcripts disproportionately.
Advantages and Disadvantages: The bias does provide insight into highly expressed genes, which can be beneficial in specific contexts. However, it limits the detection of low-expressed genes, which might be critical in certain biological instances. This poses a challenge in deriving accurate biological conclusions that rely on a complete picture of the transcriptome.
Sequence Data Complexity
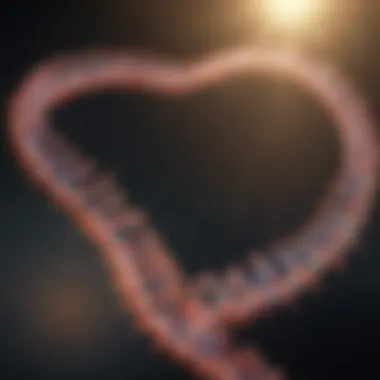
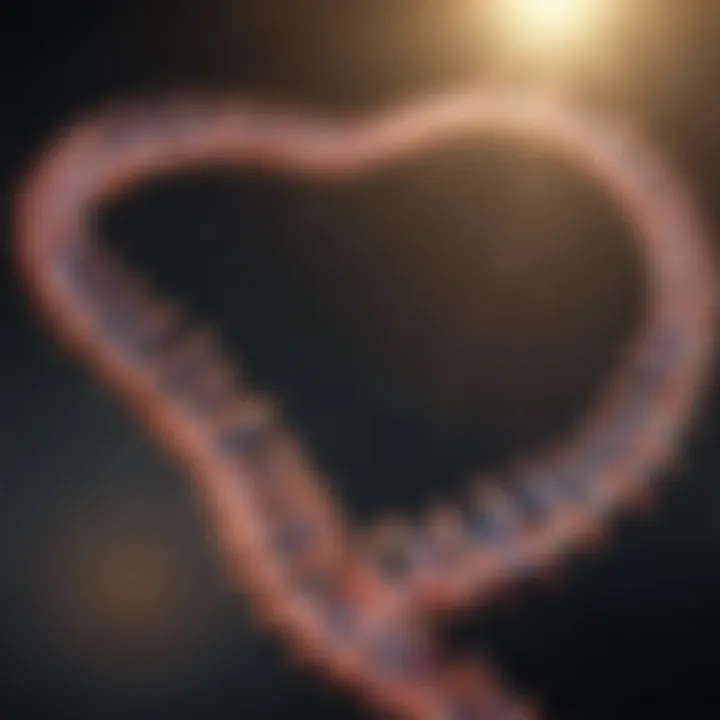
The complexity of sequence data is a another technical limitation that researchers face. RNA-seq produces large volumes of data that can include extensive variations and errors. This complexity arises from multiple sources, including sequencing errors, read-length variability, and the presence of complex genomic loci.
Key Characteristics: A characteristic of this complexity is the vast number of sequences generated, which may encompass reads from a variety of RNA molecules. This diversity increases the workload for data analysis, which must efficiently handle and interpret vast datasets.
Advantages and Disadvantages: While having a large data volume can offer more extensive insights, the challenge lies in processing and analyzing such data to extract valuable information. Accurately filtering out noise due to errors, while maintaining sensitivity to true biological signals, remains a significant hurdle in RNA-seq data analytics.
Interpretative Challenges
Data Volume Management
Managing the volume of data generated in RNA sequencing is a notable interpretative challenge. The datasets produced from RNA-seq experiments can be enormous, often ranging from gigabytes to terabytes. This data management issue is crucial for ensuring effective analysis and reproducibility of results.
Key Characteristics: The sheer size of data demands substantial storage and processing capabilities. This characteristic becomes particularly prominent in studies using high-throughput sequencing technologies.
Advantages and Disadvantages: On one hand, the ability to analyze large datasets can lead to new and unexpected biological discoveries. On the other hand, researchers must invest in significant computing resources and time for proper data management and interpretation.
Statistical Significance
The determination of statistical significance in RNA sequencing results is often challenging due to the complexity and inherent variability of RNA expression data. Proper statistical analysis is key to ensuring that observed differences are not merely due to random chance.
Key Characteristics: A unique feature of statistical significance in RNA-seq is the need for robust statistical models that can account for technical variations and biological replicates.
Advantages and Disadvantages: While rigorous statistical analysis can bolster confidence in findings, it also requires expertise and can introduce challenges if incorrectly applied. Ensuring validity and reproducibility of results hinged on statistical rigor is crucial for advancing knowledge through RNA sequencing.
Future Perspectives of RNA Sequencing
The future of RNA sequencing is both promising and complex, as advancements in technology and methodologies continue to reshape how we understand gene expression and genetic information. Embracing emerging technologies will not only enhance the efficiency and accuracy of RNA-seq but also open new avenues for research. As these advancements unfold, considerations about ethical implications and integration with other data sources become critically important.
Emerging Technologies
Improved Sequencing Methods
Improved sequencing methods are at the forefront of RNA sequencing innovation. Techniques such as single-cell RNA sequencing and 3' RNA sequencing play a significant role in refining our understanding of cellular heterogeneity and gene expression patterns at different levels. The key characteristic of these improved methods is their ability to provide higher resolution data and reduce costs compared to traditional approaches. This makes them a popular choice in contemporary research.
A unique feature of improved sequencing methods includes their higher throughput capability and reduced biases. These enhancements allow researchers to capture a more complete picture of the transcriptome. However, one must consider that while offering greater depth and precision, these methods may introduce new challenges, such as increased complexity in data analysis.
Integration with Other Omics
Integration with other omics disciplines is another pivotal aspect shaping the future of RNA sequencing. By combining RNA-seq data with genomics, proteomics, and metabolomics, researchers can develop a more holistic view of biological systems. This approach allows for a deeper understanding of the interactions and networks that govern cellular processes. The key characteristic of this integration is its ability to provide multifaceted insights, making it a beneficial choice for comprehensive biological research.
A unique feature of integrating other omics is the possibility to validate findings across various molecular layers. This strengthens results and conclusions, yet it also increases the complexity of data interpretation. Researchers need to be equipped with advanced computational tools and statistical methods to navigate these challenges effectively.
Ethical Considerations
As RNA sequencing technologies advance, ethical considerations must not be overlooked. Issues such as data privacy, consent for genetic information usage, and potential misuse of genetic data are crucial topics that researchers must address. Ensuring that ethical guidelines are in place will help safeguard not only the integrity of research but also the rights of individuals involved in scientific studies.
In summary, as RNA sequencing evolves, the intertwining of enhanced technologies with ethical frameworks will be essential. Understanding these future perspectives provides a roadmap for researchers aiming to make impactful contributions to the field.
Finale on the Role of RNA Sequencing
In this article, we have covered the intricacies and significance of RNA sequencing. This technique plays a vital role in molecular biology, acting as a bridge to understand gene expression and the complexity of transcriptomes. The importance of RNA sequencing lies not only in the clarity it brings to the study of RNA but also in its contributions to broader scientific research and applications.
Summary of Key Findings
RNA sequencing has revolutionized the field of genomics. Below are some of the key findings that emphasize its importance:
- Comprehensive Transcriptome Analysis: RNA-seq allows for a detailed portrait of the transcriptome, providing insights into gene expression levels and variations.
- Novel Transcript Discovery: This technique has exposed previously unidentified RNA species, shedding light on potential new biological functions.
- Single-Cell Resolution: Advances in single-cell RNA sequencing have enabled deeper exploration of cellular heterogeneity and differentiation, which is crucial in fields such as cancer research.
- Implications for Drug Development: Insights obtained from RNA-seq data are instrumental in tailoring therapies to specific gene expression patterns, particularly in oncology.
These findings collectively strengthen the case for adopting RNA sequencing as a standard approach in research laboratories worldwide.
Final Remarks
As we conclude, it is essential to recognize that RNA sequencing is not merely a technical tool; it is a gateway to understanding biological processes. The advancements in RNA sequencing methodologies and applications will continue to evolve, pushing the boundaries of research. The benefits it brings are significant, yet it is vital to remain cognizant of the limitations and challenges associated with data interpretation. As researchers navigate this landscape, the role of RNA sequencing in shaping our understanding of complex biological systems will remain unparalleled. The ongoing exploration of RNA will undoubtedly yield further advancements in health and disease.
"RNA sequencing acts as a lens, allowing us to view the dynamic world of gene expression with unprecedented clarity."
By integrating emerging technologies and addressing ethical considerations, future perspectives on RNA sequencing appear promising. This ensures that the knowledge generated can be translated into tangible benefits for society, emphasizing the need for continued innovation and ethical responsibility in scientific research.