Understanding Protein: Structure, Function, and Impact
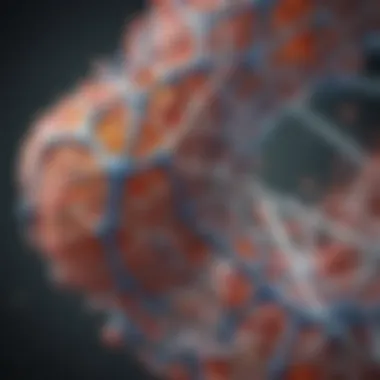
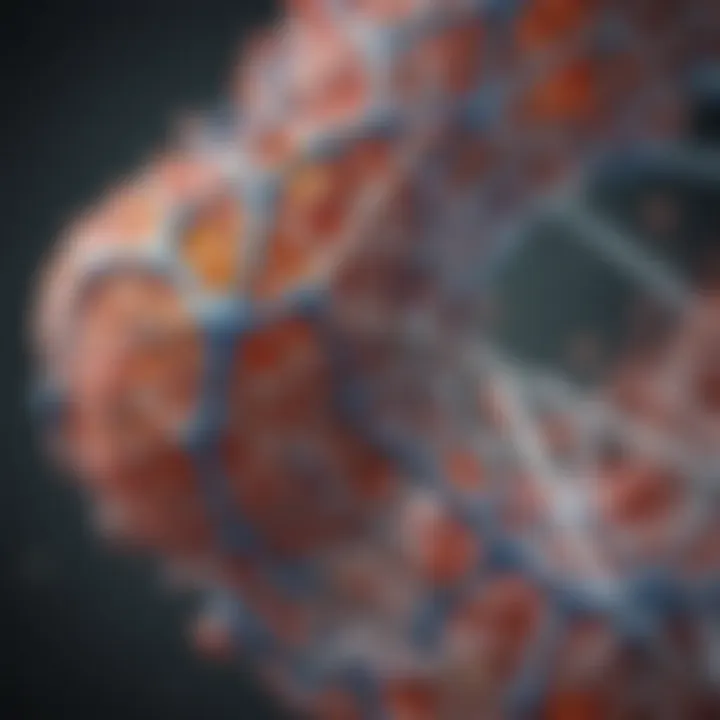
Intro
Proteins, often hailed as the workhorses of the cell, play a crucial role in countless biological processes. Their intricate structure and diverse functions underscore their centrality within any living organism. As we embark on this journey through the multifaceted world of proteins, we will unpack their significance from cellular mechanisms to broader implications in health and nutrition.
This article sets to illuminate the varied roles of proteins, emphasizing how their structure is directly linked to their function. From enzymes speeding up chemical reactions to antibodies defending the body against pathogens, proteins are at the heart of life itself. Moreover, understanding the impact of proteins on metabolic pathways and their role in diseases can unveil pathways for therapeutic interventions.
In varying degrees, the study of proteins extends beyond theoretical biology, influencing areas like nutrition, medicine, and biochemistry. By synthesizing insights from research, we can appreciate the importance of proteins in not only sustaining life but also in shaping our well-being. Let’s dive deeper into methodologies used in protein research, examining the techniques and technologies that help scientists untangle this complex sphere.
Understanding Proteins: A Foundation
Proteins are the workhorses of the cellular world, underpinning countless biochemical processes that dictate life itself. In discussing the importance of understanding proteins, it is crucial to appreciate not just their structure but also their myriad functions within distinct biological contexts. The foundation of protein science provides insights into how living organisms maintain homeostasis, respond to environmental cues, and engage in intricate signaling pathways. Navigating this complex web reveals how proteins are indispensable in health, development, and disease.
The Nature of Proteins
At their core, proteins are large, complex molecules formed by long chains of amino acids. Amino acids, often deemed the building blocks of life, link together through peptide bonds. Each protein's unique sequence of amino acids determines its structure and function—a point that cannot be overstated. For example, consider collagen, primarily composed of amino acids like glycine and proline. This protein imparts strength and structural integrity to tissues like skin and bones.
Proteins also undergo intricate folding processes that are influenced by environmental factors like pH and temperature. Their shapes—be it fibrous, globular, or membrane-bound—directly correlate with their roles within biological systems. For instance, the characteristic helical structure of alpha-keratin enables its use in hair and nails, reflecting a fascinating interplay between structure and function.
Significance in Biological Systems
The significance of proteins in biological systems is truly profound. They are involved in virtually every cellular process, from catalyzing biochemical reactions to orchestrating cellular movement and maintaining cellular structure. The diversity of proteins manifests in various forms:
- Enzymes, which accelerate metabolic reactions, are essential for processes like digestion and DNA replication.
- Transport proteins, such as hemoglobin, carry oxygen in the bloodstream, vital for cellular respiration and energy production.
- Signal proteins, like insulin, regulate processes across various systems, influencing activities such as glucose metabolism.
Understanding these protein functions lays the groundwork for investigating their roles in health and disease. Misfolding or deficiency of specific proteins can trigger a cascade of unfortunate outcomes, as seen in disorders like cystic fibrosis or sickle cell anemia. Thus, gaining a foundational knowledge of proteins is not merely an academic exercise; it is key to unlocking solutions for many biomedical challenges.
"Proteins are not just macromolecules; they are the mediators of life’s complexities."
In essence, embarking on a journey into the world of proteins sheds light on the intricate balancing act that sustains life. A solid foundation in the understanding of proteins is essential for students, researchers, educators, and professionals alike, providing the critical framework necessary to explore the frontiers of biological sciences.
Chemical Composition of Proteins
In the world of molecular biology, understanding the chemical composition of proteins is paramount. It lays the foundation for knowing how life functions on a cellular level. Proteins play versatile roles in the body, acting as enzymes, structural components, and signaling molecules, influencing almost every biochemical process. Their composition directly correlates to their function, making it a topic that requires close attention.
The primary players in this composition are amino acids and peptide bonds. Each amino acid contributes unique properties to the protein, whether it allows flexibility, strength, or specific attachment properties. The variety in amino acid composition is what allows proteins to exhibit such a vast array of functions, from muscle contraction to immune response. One can't overlook how that intricate dance of chemistry translates into physical phenomenon at the larger scale.
Amino Acids: The Building Blocks
Amino acids are often dubbed the "building blocks" of proteins, and for a good reason. There are 20 standard amino acids that combine in various sequences to form different proteins. Each amino acid consists of a basic structure with an amino group, a carboxyl group, and a side chain (or R-group) that varies among amino acids. The identity of this R-group is what distinguishes one amino acid from another, imparting distinct characteristics.
When discussing amino acids, two main categories come to light: essential and non-essential.
- Essential amino acids must be obtained through diet, as the body cannot synthesize them. Examples include leucine and valine, critical for muscle repair and growth.
- Non-essential amino acids can be synthesized by the body, such as alanine and asparagine, yet they still play crucial roles in metabolic pathways.
In a nutshell, the specific arrangement of these amino acids creates a unique protein structure, and any alteration can affect its function significantly. Think of it like a chef's special ingredient mix; alter just one and the flavor could change drastically.
Peptide Bonds and Their Formation
When amino acids come together to form proteins, they don’t just line up next to each other. Instead, they form a covalent bond known as a peptide bond. This bond is formed through a dehydration synthesis reaction, where a molecule of water is removed, allowing the amino group of one amino acid to bind with the carboxyl group of another.
This process can be explained through the following simple steps:
- Two amino acids approach each other. The amino group (–NH₂) of one bonds with the carboxyl group (–COOH) of the other.
- Water is released. As the bond forms, one hydrogen from the amino group and a hydroxyl from the carboxyl group are lost, yielding a water molecule.
- Formation of a peptide bond. The resultant bond is now termed a peptide bond, linking these amino acids into a chain.
"The sequence of amino acids in a protein determines its shape, and consequently its function."
Understanding how peptide bonds are formed is crucial because the stability and flexibility of a protein can entirely hinge on these connections. If you take the time to appreciate this seemingly simple bond, you begin to realize that it underpins the complex tapestry of protein function within living organisms.
Overall, the chemical composition of proteins not only informs their structural characteristics but also their roles within biological systems. Understanding this undercurrent offers insights into health, nutrition, and the very essence of life itself. Fascinating, isn’t it?
For a deeper dive into proteins, you can explore more on platforms like Wikipedia, Britannica.
Protein Structure: An In-Depth Analysis
Understanding the structure of proteins is paramount for grasping their myriad functions and how they impact biological systems. At a basic level, the structure of a protein dictates its shape, which subsequently influences its behavior and role within the body. A nuanced comprehension of protein structures fosters insights not only into their biochemical properties but also into potential therapeutic applications. Such understanding offers a molecular blueprint for biologists, researchers, and medical professionals, guiding innovations in treatments for diseases associated with protein malfunctions.
Primary Structure: Sequence Matters
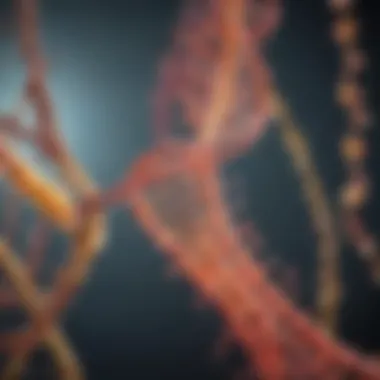
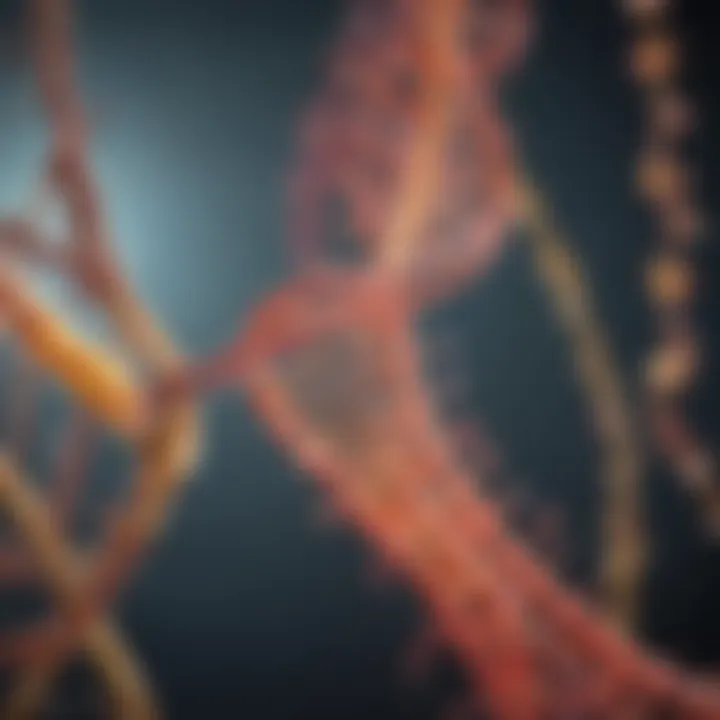
The primary structure refers to the unique sequence of amino acids that compose a protein. This sequence resembles a string of beads, where each bead is an amino acid linked together by peptide bonds. Notably, the specific arrangement of amino acids is critical, as even a single change can lead to drastic variations in the protein's function. For instance, sickle cell anemia is caused by a single mutation in the hemoglobin gene which alters the sequence of 146 amino acids, resulting in a malformed protein that can’t effectively transport oxygen.
- Key Points about Primary Structure:
- It determines the protein's identity.
- Plays a crucial role in the final shape of the protein.
- Dictates the functional attributes of the protein.
Secondary Structure: Form and Function
The secondary structure refers to localized folding patterns within the protein, primarily formed by hydrogen bonds. The two most common forms of secondary structure are alpha helices and beta sheets. These formations occur as the peptide chain bends and folds back on itself, which enhances the overall stability of the protein.
This structural level has significant implications. For example, keratin, found in nails and hair, exemplifies a large amount of alpha helices, lending strength and resilience. Conversely, silk fibroin consists largely of beta sheets, contributing to its softness and flexibility.
- Types of Secondary Structures:
- Alpha Helices: Coiled structures that provide elasticity.
- Beta Sheets: Pleated structures that offer strength.
Tertiary Structure: The Complex Fold
Tertiary structure represents the comprehensive 3D shape of a protein formed by the bending and folding of the secondary structure. This intricate conformation arises from various interactions, including hydrophobic interactions, ionic bonds, and disulfide bridges between distant amino acids in the sequence.
The role of tertiary structure is critical in determining the protein’s function. Enzymes, for instance, have active sites formed by tertiary folding that allow them to interact with substrates. When a protein misfolds at this stage, it can lead to loss of function or, in some cases, diseases like Alzheimer's.
- Key Aspects of Tertiary Structure:
- Critical for functionality.
- Could be altered by environmental factors (like pH and temperature).
Quaternary Structure: Interaction of Multiple Chains
Some proteins are composed of multiple polypeptide chains that come together to form a functional unit. This assembly is referred to as quaternary structure. The interactions between these chains can enhance stability and expand functionality. Hemoglobin is a prime example, made up of four globin chains working together to bind oxygen efficiently.
Understanding quaternary structure is essential for grasping how proteins communicate with one another and operate within cellular contexts. Changes in this structure can profoundly affect protein efficacy and have repercussions on physiological processes.
- Examples of Quaternary Structures:
- Hemoglobin: Facilitates oxygen transport.
- Antibodies: Engaged in immune response.
In summary, the structures of proteins—their primary, secondary, tertiary, and quaternary forms—are not only fundamental to understanding their biological functions but are also crucial for exploring new pathways in research and treatments in diseases associated with protein malfunctions.
Protein Synthesis: The Central Dogma
The process of protein synthesis is at the heart of molecular biology, often referred to as the central dogma of biology. It delineates how genetic information encoded within DNA ultimately results in the production of proteins, which are key players in virtually every biological function. Understanding this process is crucial for students, researchers, and professionals alike, as it not only illuminates the pathway from gene to protein but also underscores the intricacies involved in regulating cellular functions. The significance of protein synthesis extends beyond mere biological curiosity; it has profound implications in fields such as genetics, medicine, and biotechnology.
Transcription Process
Transcription marks the initial step in the pathway from DNA to protein. In simple terms, it can be described as the conversion of a specific segment of DNA into messenger RNA (mRNA). Here’s a clearer breakdown of how this works:
- Initiation: The transcription process begins when the enzyme RNA polymerase attaches to a specific sequence on the DNA known as the promoter region. This region acts as a signal for the RNA polymerase to start synthesizing mRNA. Moreover, transcription factors may assist in this binding process to ensure accuracy.
- Elongation: Once attached, RNA polymerase unwinds the DNA helix and begins adding RNA nucleotides in a sequence complementary to the DNA strand. A crucial point is that the new mRNA strand grows in the 5’ to 3’ direction, fundamentally different from the way the DNA template is read.
- Termination: This phase occurs when RNA polymerase encounters a termination sequence on the DNA. Upon reaching this sequence, RNA polymerase detaches, and the newly synthesized mRNA strand is released. Notably, this primary mRNA transcript is often modified in eukaryotic cells – it undergoes processes such as splicing, capping, and polyadenylation, preparing it for export from the nucleus.
The significance of transcription cannot be overstressed. It serves as a regulatory checkpoint, giving cells the ability to control which proteins are synthesized based on their immediate needs and environmental cues.
"Transcription can be seen as the first step in orchestrating the symphony of life, where each gene's expression is crucial for maintaining harmony in cellular functions."
Translation Mechanism
Once the mRNA has been processed and transported to the ribosome, the next step is translation, which is inherently the synthesis of proteins based on the encoded information within the mRNA. Here's how the translation mechanism unfolds:
- Initiation: During this stage, the small ribosomal subunit binds to the 5' end of the mRNA strand. A special initiator tRNA carries the amino acid methionine and pairs with the start codon on the mRNA. Subsequently, the large ribosomal subunit joins to form a complete ribosome, allowing the translation process to commence.
- Elongation: In this phase, tRNA molecules transport specific amino acids to the ribosome corresponding to the codons on the mRNA. The ribosome catalyzes the formation of peptide bonds between the amino acids, creating a growing polypeptide chain. Each cycle of elongation adds a new amino acid to the chain; this continues until a stop codon is reached.
- Termination: This final step occurs when a stop codon on the mRNA joins with a protein called release factor. The release factor triggers the dissociation of the ribosomal subunits, releasing the complete polypeptide chain. Once synthesized, the protein may undergo various post-translational modifications before it can perform its specific functions within the cell.
In summary, the translation process is vital as it translates the genetic code into functional proteins, playing a crucial role in cellular function, signaling, and response. Understanding both the transcription and translation processes is pivotal for grasping how life operates at the molecular level.
Functional Diversity of Proteins
The functional diversity of proteins is at the heart of biological systems, acting as the dynamic components that enable life. Proteins perform a myriad of tasks, shaping the very fabric of cellular and physiological processes. Understanding this diversity is paramount in appreciating how proteins contribute not just to individual health, but to the intricate web of interactions that sustain all forms of life. From facilitating chemical reactions to providing structural support, proteins embody a remarkable complexity that is both fascinating and crucial.
Enzymatic Proteins: Catalysts in Biochemical Reactions
Enzymatic proteins, also known simply as enzymes, serve as biological catalysts that accelerate biochemical reactions. They are essential for processes such as digestion, metabolism, and DNA replication. These proteins lower the activation energy required for reactions, thus making them proceed at rates suitable for sustaining life.
Key Features of Enzymatic Proteins:
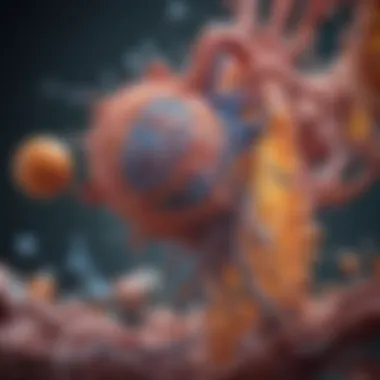
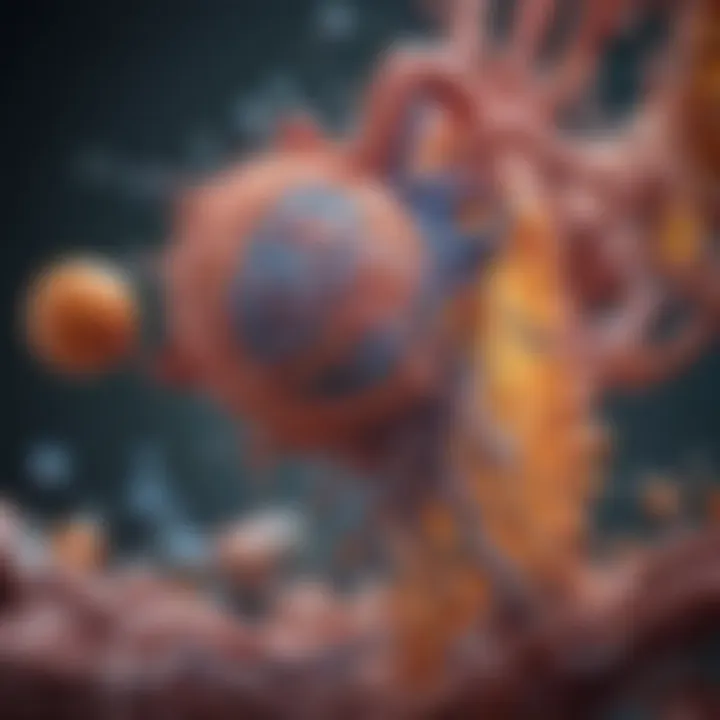
- Specificity: Enzymes are highly specific, meaning each one catalyzes a particular reaction or type of reaction.
- Regulation: Enzyme activity can be regulated through various mechanisms, including allosteric regulation, where the binding of a molecule at one site affects the activity at another.
- Cofactors and Coenzymes: Many enzymes require additional non-protein molecules, such as metal ions or organic molecules, to function effectively. For instance, iron is crucial for hemoglobin’s function, while vitamins often serve as coenzymes.
Enzymatic proteins are vital components of metabolic pathways, and their dysfunction can lead to a myriad of diseases. For instance, the deficiency of certain enzymes can cause metabolic disorders, revealing how intricately linked enzyme function is to health.
Structural Proteins: Framework of Life
Structural proteins are the scaffolding of cells and tissues. They provide support, shape and strength, playing a pivotal role in determining the physical characteristics of organisms. Collagen, keratin, and fibroin are prime examples that show how these proteins contribute to structural integrity.
Characteristics of Structural Proteins:
- Durability: These proteins are incredibly strong and resilient. For example, collagen fibers offer tensile strength in connective tissues.
- Variety of Forms: Structural proteins are found in muscles, skin, tendons, and many other tissues, demonstrating their versatility.
- Role in Cell Structure: They help form the cytoskeleton, an essential framework that gives cells their shape and organization.
Being the framework upon which biological systems are built, structural proteins have critical implications in health. Conditions like osteogenesis imperfecta arise from defects in collagen production, showcasing their importance in maintaining bodily integrity.
Transport Proteins: Facilitators of Movement
Transport proteins are responsible for carrying substances across cell membranes and throughout the body. They play a significant role in maintaining homeostasis by regulating the movement of ions, nutrients, and waste. Hemoglobin, the protein that carries oxygen in red blood cells, exemplifies this function.
Functions of Transport Proteins:
- Selective Permeability: Transport proteins selectively allow certain molecules to pass while blocking others, thereby maintaining the cell's internal environment.
- Energy Utilization: Many transport activities require energy; for instance, the sodium-potassium pump moves ions against their concentration gradients, vital for electrical activity in neurons.
- Role in Disease: Malfunctioning transport proteins can lead to various health issues. Conditions such as cystic fibrosis are associated with defective transport proteins, highlighting their crucial role in health.
Hormonal Proteins: Regulators of Biological Processes
Hormonal proteins act as signaling molecules that regulate physiological processes across various systems in the body. They travel through the bloodstream to target organs, where they initiate responses to maintain homeostasis. Insulin and glucagon are two well-studied examples of this class of proteins.
Functions of Hormonal Proteins:
- Regulation of Metabolism: Hormones control metabolic processes, influencing how the body utilizes energy. For instance, insulin lowers blood sugar levels by facilitating the uptake of glucose by cells.
- Growth and Development: Hormonal proteins are crucial during growth phases, regulating processes from childhood to adulthood. Growth hormone is a key player in this context.
- Response to Stress: Hormonal signals such as adrenaline prepare the body to respond to stressors, demonstrating their role in the fight-or-flight response.
Each category of proteins brings a distinct functional ability, contributing considerably to the biological panorama. Their functional diversity highlights the adaptability and complexity of life, framing an essential theme not just in this article but in the very essence of biology itself.
Protein Misfolding and Disease
Understanding the relationship between protein misfolding and disease is crucial for comprehending a multitude of health issues that arise due to errors in protein structure. Misfolded proteins can lead to a range of pathologies, where the delicate balance of protein functionality is disrupted. As proteins are essential for numerous cellular processes, their incorrect folding underscores a significant aspect of biomedical research and potential therapeutic interventions.
This section will spotlight the mechanisms through which proteins misfold, introducing the nuances that lead to abnormalities and their corresponding diseases. Focusing on the biological implications of misfolding helps to illuminate its relevance not only to basic science but also to clinical applications.
"In the grand scheme of life, the proper folding of proteins is not just a minutiae; it's the linchpin of cellular survival."
Mechanisms of Misfolding
Protein misfolding can arise from a variety of factors, and it often involves a cascade of molecular missteps. Firstly, mutations in the amino acid sequences are a prime culprit. Even a single change in the sequence can lead to misfolding, as the three-dimensional structure of the protein can be particularly sensitive. When these errors occur during synthesis, the resulting protein may fail to reach its properly folded state.
Additionally, environmental conditions such as temperature, pH, and concentration of salts play a pivotal role in protein stability. For instance, elevated temperatures can induce denaturation, a state where the normal structure unwinds and loses its functional capabilities.
Other mechanisms include:
- Aggregation: Misfolded proteins can cluster together, forming insoluble aggregates that disrupt cellular function.
- Chaperone Malfunction: Chaperone proteins, which assist in the proper folding of others, may become overburdened or dysfunctional, leading to more proteins misfolding.
- Pathological Stress: Stress conditions within cells, such as oxidative stress, can also contribute to protein misfolding.
Understanding these mechanisms not only sheds light on the basic biology of proteins but also highlights potential therapeutic targets to correct or mitigate misfolding.
Associated Diseases: A Review
The consequences of protein misfolding are far-reaching and can manifest in a variety of serious diseases. Notable examples of conditions linked to this phenomenon include:
- Alzheimer's Disease: Characterized by the accumulation of amyloid plaques, which consist of misfolded amyloid-beta peptides that disrupt cell function and ultimately lead to neurodegeneration.
- Parkinson's Disease: Involves the aggregation of alpha-synuclein, leading to neuronal cell death and motor dysfunction.
- Huntington's Disease: Caused by the misfolding of huntingtin protein, resulting in cognitive decline and movement disorders.
- Cystic Fibrosis: Often stem from mutations in the CFTR protein, which affect its folding and result in severe respiratory and digestive issues.
These diseases underscore the cascade of biological dysfunction that can follow from a seemingly simple misstep in protein folding. Research continues to explore how therapeutic interventions can either correct misfolded proteins or bolster cellular mechanisms to manage these abnormalities.
By highlighting the complexity and significance of protein misfolding, we can better appreciate the broader implications it carries for human health and potential strategies for intervention. As we move forward in our understanding, the need for effective treatments becomes ever more pressing.
Nutritional Role of Proteins
Proteins play a vital role in maintaining the health and functionality of the human body. They are not just mere building blocks, as one might think; their relevance stretches far beyond that simple definition. In the context of this article, understanding the nutritional role of proteins opens a window into how they contribute to our overall health, support metabolic processes, and enhance physical performance. Engaging with this topic allows us to appreciate the nuanced ways that proteins affect our bodily functions on a daily basis.
Firstly, proteins serve as essential nutrients for growth and repair. This is especially important for active individuals, athletes, and those recovering from injury. They help in the synthesis of muscle, skin, and organs. When proteins break down, they release amino acids, which are then utilized for various bodily functions, promoting healing and maintaining tissue health. Also, as the body can’t store amino acids like it can glucose or fat, daily intake is crucial, making awareness of dietary choices fundamental.
Moreover, proteins are responsible for producing enzymes and hormones that regulate numerous physiological processes, from digestion to mood stabilization. For instance, insulin, a protein hormone, plays a key role in managing blood sugar levels. Without adequate protein intake, the body struggles to produce sufficient amounts of these important regulators, which might lead to imbalances and health complications.
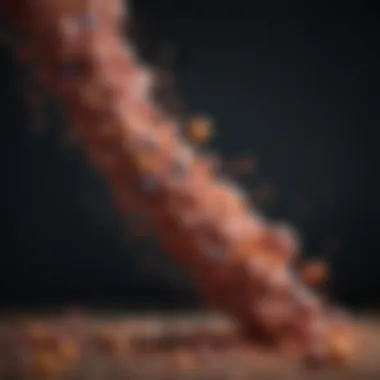
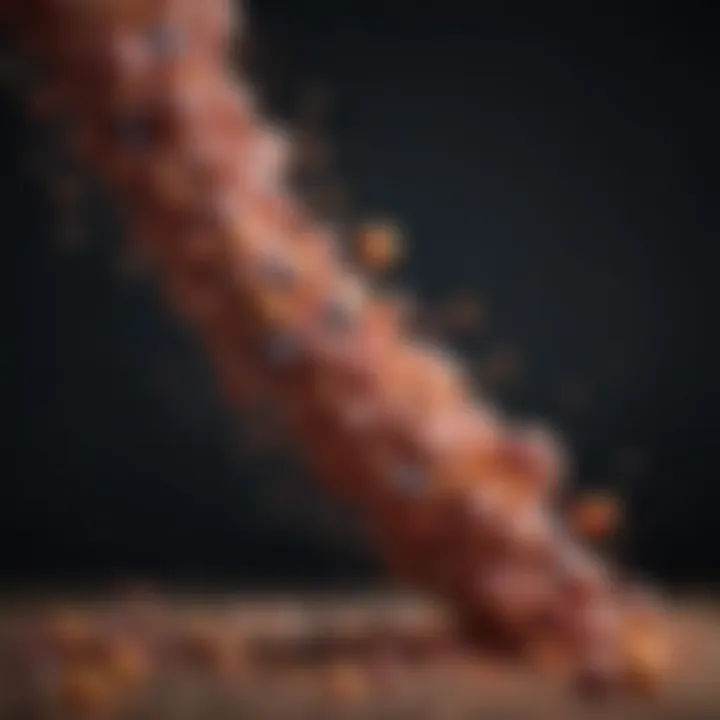
The following sub-sections will delve deeper into specific dietary sources of protein and the recommended intake to meet both health goals and lifestyle demands.
Dietary Sources of Protein
When discussing dietary sources of protein, it’s crucial to recognize the variety of both animal-based and plant-based options available. Each source provides distinct benefits, with their own amino acid profiles.
- Animal Sources: These tend to be rich in all essential amino acids. Foods such as chicken, beef, fish, eggs, and dairy products emerge as excellent choices. They are often referred to as complete proteins, crucial for supporting muscle repair and growth. On the downside, consuming excessive amounts of red and processed meats might present health risks, including heart disease and certain cancers.
- Plant Sources: For those pursuing vegetarian or vegan diets, there are ample plant-based proteins available. Options like lentils, chickpeas, quinoa, and tofu can provide a solid protein intake. Though several plant proteins are incomplete, combining them can ensure a complete amino acid profile. For example, rice and beans together offer all essential amino acids.
Incorporating a mix of both animal and plant proteins can yield the best results, catering not only to protein needs but also supplying a rich array of nutrients and fibers.
Recommendations for Protein Intake
Determining an appropriate protein intake often depends on various factors—age, activity level, and overall health. But as a general guideline:
- Recommended Daily Allowance (RDA): For the average adult, the RDA for protein is about 46 grams for women and 56 grams for men daily.
- Active Individuals: Those who engage in regular physical activity may need around 1.2 to 2.0 grams of protein per kilogram of body weight, based on exercise intensity and goals.
- Older Adults: Research suggests that older adults might benefit from higher protein intakes, approximately 1.0 to 1.2 grams per kilogram of body weight, to mitigate age-related muscle loss.
It’s important to space protein intake throughout the day, ensuring each meal contains a source of protein, which helps with satiation and promotes better muscle protein synthesis.
Ultimately, it’s advisable to consult with a healthcare professional or a registered dietitian to tailor protein recommendations based on individual needs and conditions.
"Incorporating a diverse range of protein sources not only supports health but also adds flavor and variety to one’s diet."
In summary, the nutritional role of proteins is indispensable in fostering overall well-being and optimizing bodily functions. As we embark on this journey through the topic, we lay the groundwork for further exploration into how proteins function in our diets, influencing everything from energy levels to muscle performance.
Current Research Trends in Protein Studies
The field of protein studies has continuously evolved, reflecting the complex nature of proteins and their integral role in life. Current research trends shed light on the intricacies of protein functions, structures, and their implications in health and disease. These trends highlight a fundamental understanding that is reshaping therapeutic approaches, diagnostics, and even nutritional science. Understanding these trends provides clues to enhancing human health and addressing diseases at a molecular level.
Advancements in Protein Engineering
The term protein engineering encompasses a variety of techniques aimed at designing and modifying proteins for specific purposes. The advancements in this area are nothing short of revolutionary. Scientists have developed methods to alter protein structures, which can yield enzymes that work under extreme conditions or even improve the efficiency of biomolecular processes. Techniques such as directed evolution and CRISPR-Cas9 have opened new pathways for customizing proteins.
This has immense implications in industries ranging from pharmaceuticals to renewable energy. For instance, modified proteins can facilitate the breakdown of waste materials, turning a potential environmental disaster into a resource. Moreover, engineered proteins have the potential to serve as more potent drugs or as targeting agents in cancer therapies. This tandem of increased efficacy and specificity can change the landscape of treatment protocols.
- Key areas of focus in protein engineering include:
- Novel Enzymes: Creating enzymes that can catalyze reactions that traditional methods can't.
- Therapeutics Development: Engineering proteins that can specifically target and disrupt the mechanism of disease-causing agents.
- Vaccine Innovations: Modifying proteins to enhance vaccine efficacy through better immune responses.
As proteins are nature's workhorses, understanding how to manipulate them proficiently asserts a huge advantage across the board in science and application.
The Role of Proteomics in Disease Understanding
Proteomics, the study of the protein content of cells, tissues, and organisms, stands at the forefront of modern biomedical research. With the ability to analyze thousands of proteins simultaneously, proteomics plays a crucial role in understanding the molecular underpinnings of diseases. By examining protein expression levels, modifications, and interactions, researchers can identify biomarkers that signify disease states.
For example, in cancer research, proteomics has enabled scientists to discover specific protein signatures unique to different cancer types, facilitating earlier detection and more precise treatments. These protein profiles can also guide therapy choices, leading to personalized medicine approaches that consider the patient's specific proteomic makeup.
"Proteomics does not only identify what proteins are present; it elucidates how they interact and contribute to processes, sometimes revealing unexpected pathways involved in disease progression."
- Impactful Contributions of Proteomics Include:
- Biomarker Discovery: Identifying proteins that signify particular diseases.
- Drug Target Identification: Pinpointing proteins involved in disease pathways for targeted treatments.
- Understanding Disease Mechanisms: Unraveling how protein interactions dictate cellular responses, which can lead to new therapeutic approaches.
In summary, both protein engineering and proteomics are paving the way for innovative solutions to past medical challenges. As these research trends continue to advance, they hold the potential to redefine the frontiers of health and disease management.
Future Perspectives in Protein Research
As we stand at the crossroads of biology and technology, the future of protein research presents a world of possibilities that hold immense potential. Understanding proteins has always been fundamental to biology, but as we explore deeper into their structures and functions, we are uncovering pathways that were once invisible to us. This section delves into the key elements surrounding emerging trends in protein research, including their therapeutic applications and the impact of advanced technologies on our understanding.
Potential Therapeutic Applications
The therapeutic landscape is rapidly evolving thanks to protein research. It’s fascinating how proteins, which are vital for many cellular functions, can be harnessed to treat various diseases. Here, we look into a few notable applications:
- Monoclonal Antibodies: These proteins are engineered to target specific antigens on pathogens or cancer cells. For instance, Herceptin is a well-known monoclonal antibody used in breast cancer treatment. The ability to create specific antibodies has revolutionized cancer therapy, leading to more personalized and effective treatment options.
- Enzyme Replacement Therapy: In cases of genetic disorders like Gaucher's disease, where enzyme deficiencies occur, scientists have developed treatments that replace missing enzymes. These therapeutic proteins can correct metabolic pathways and drastically improve quality of life for affected individuals.
- Gene Therapy: Though this is an indirect application, utilizing proteins such as CRISPR-associated protein Cas9 facilitates gene editing, allowing for corrections in genetic mutations at the DNA level. This has opened doors for tackling genetic disorders that were previously considered untreatable.
The benefits of these advancements are monumental, yet considerations regarding accessibility, cost, and ethical implications remain essential discussions in the field. Equally significant is ensuring that therapeutic proteins are effective, safe, and free from immunogenic responses.
The Impact of Technology on Protein Research
Technological advancements are reshaping protein research by providing tools that enhance our understanding and manipulation of proteins. Here are some key technologies making waves in the field:
- Next-Generation Sequencing (NGS): NGS allows for rapid sequencing of entire genomes, including those encoding for proteins. This technology has dramatically increased our capacity to identify genetic variations that contribute to protein structure and function, enhancing our capacity for discovering biomarkers for diseases.
- Cryo-Electron Microscopy: This cutting-edge imaging technique provides high-resolution structures of proteins in their native states without crystallization. It has become a game-changer for visualizing protein complexes and understanding their interactions at an unprecedented scale.
- Artificial Intelligence (AI): AI models, particularly those used in predicting protein folding, are transforming the field of protein research. These models can predict the 3D structure of proteins based on their amino acid sequences, as demonstrated by tools like AlphaFold, significantly accelerating research efforts.
Unquestionably, the impact of technology extends beyond mere data processing; it’s about generating insights that drive innovation in therapeutic developments and biological understanding.
"The next era of protein research is not just about understanding but also about manipulating and applying that knowledge for societal benefit."