Exploring the Mechanisms of Muscle Contraction
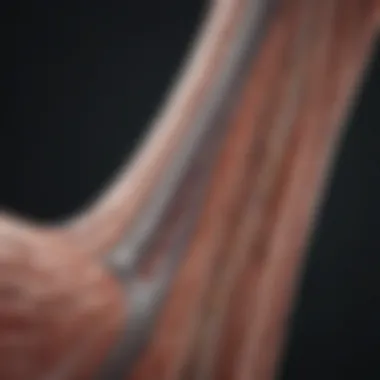
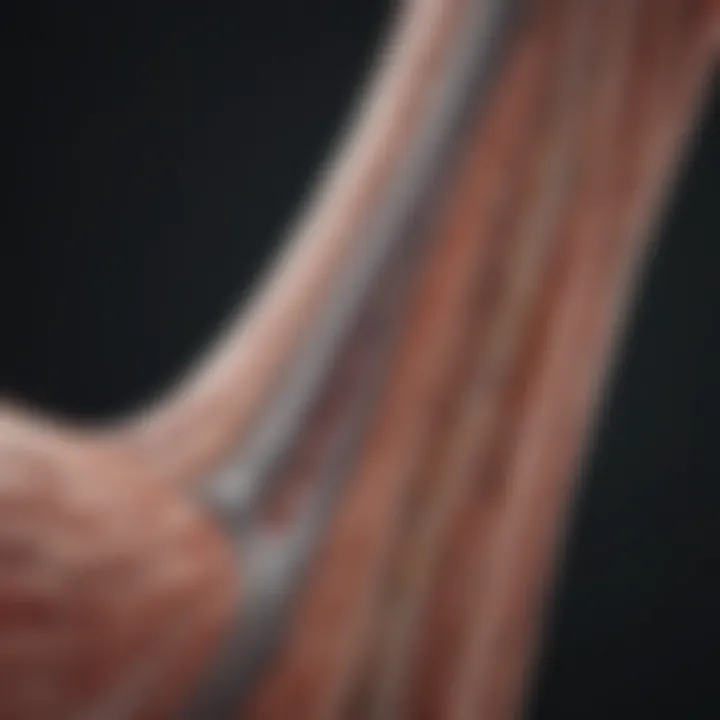
Intro
Muscle contraction plays a pivotal role in our daily lives, influencing everything from the simplest movements like picking up a pen to intricate actions such as a dancer's leap. Understanding the causes behind this essential physiological process can shed light not just on how our bodies function, but also on potential health implications.
Muscles can be broadly categorized into three types: skeletal, smooth, and cardiac. Each type exhibits unique characteristics and functions, yet they all rely on similar underlying mechanisms of contraction. In this article, we will dissect these mechanisms and consider the anatomical, biochemical, and neurological factors at play.
Additionally, we’ll discuss how research in this field continues to evolve, thus enhancing our comprehension of muscular functions, as well as related health conditions. Let’s embark on this journey to explore the intricate dance of muscle contractions, gaining insights that could be beneficial for students, researchers, educators, and professionals alike.
Methodologies
To fully grasp the complexities of muscle contraction, it is essential to delve into the methodologies of research that have shaped our current understanding. The approaches used in this field blend various disciplines, each contributing insights that help paint a complete picture.
Description of Research Techniques
Muscle contraction research often employs a mix of laboratory testing, observational studies, and advanced simulation techniques. For instance:
- Electromyography (EMG): Used to measure muscle electrical activity,
- Force Measurement: Employing various testing apparatus to gauge the force produced by muscle tissues during contractions,
- Fibers Histology: Examining muscle fiber types under a microscope to understand their roles in different contraction types.
These techniques allow researchers to piece together the physiological puzzle, revealing how different muscle types respond under various conditions.
Tools and Technologies Used
The technology employed in muscle contraction research has significantly advanced over the years. Notable tools include:
- High-Speed Cameras: Capturing rapid movements for further analysis
- Computational Models: Simulating muscle behavior under different scenarios
- Functional MRI (fMRI): Monitoring blood flow and activity in muscles during contraction
As methods evolve, so does our understanding of muscle contraction dynamics, paving the way for new therapeutic strategies and interventions.
Discussion
Comparison with Previous Research
Historically, muscle contraction was understood primarily through legacy theories like the sliding filament theory. Groundbreaking studies back then laid foundational knowledge, painting muscles as systems of filaments that slide past one another. Yet, as research methodologies advanced, the narrative has grown more complex. Recent insights challenge old beliefs, emphasizing the role of phosphorylation, and calcium dynamics in muscle contractions, showcasing a more nuanced interaction among various cellular components than previously recognized.
Theoretical Implications
The implications of these findings extend far beyond academic interest. A deeper understanding of muscle contraction can inform therapeutic techniques for muscle-related diseases, sports medicine, and rehabilitation strategies. Insights garnered can foster better preventative measures for muscle deterioration with age, and guide research on conditions like muscular dystrophy.
By harmonizing anatomical understanding with biochemical processes and neural controls, we approach a more holistic perspective on muscle contraction that could have vast clinical implications.
Prelude to Muscle Contraction
Muscle contraction is at the heart of many physiological processes that allow for movement, stability, and coordination in the human body. Understanding how muscles contract is not just of academic interest; it underpins everything from athletic performance to the management of various muscle disorders. When we talk about muscle contraction, we aren’t merely touching upon the physical act of flexing a bicep or taking a step. It's a multifaceted process that integrates anatomical, biochemical, and neurological components.
In the following sections, we will dissect the definition of muscle contraction, delve into the types of muscle tissues involved, and examine the intricate biochemical and neurological pathways that make this phenomenon possible. A comprehensive grasp of muscle contraction can inform best practices in physical therapy, sports science, and general health management. Moreover, it can aid in developing effective rehabilitation strategies for individuals recovering from muscle-related injuries or diseases.
**"The understanding of muscle contraction can open doors to innovations in treatments and therapies that enhance physical health."
This journey into muscle contraction aims not only to educate but also to inspire curiosity about the biological marvels that function in our everyday motions. It lays the groundwork for further exploration into how various factors may affect these processes, be they physical attributes or chemical imbalances. With this foundational context, we can better appreciate the importance of muscle contraction in both health and disease contexts.
Types of Muscle Tissue
Understanding the various types of muscle tissue is crucial for grasping how our bodies perform movements and maintain overall function. Each type of muscle contributes differently to our physiology, and knowing their unique characteristics and roles can provide insights into how muscle contraction occurs and how it can be affected by various factors. In this section, we will explore the three primary types of muscle tissue: skeletal muscle, cardiac muscle, and smooth muscle. This exploration will reveal their structural differences, functional roles, and implications in health and disease.
Skeletal Muscle
Skeletal muscle is probably the most recognized type of muscle, mainly because of its direct connection to voluntary movements. Found attached to bones via tendons, these muscles are responsible for orchestrating movements that enable walking, lifting, and other physical activities.
Key characteristics of skeletal muscle include:
- Striated appearance: Under the microscope, skeletal muscle displays a striped pattern. This striation reflects its organized structure, which is critical for its function.
- Multi-nucleated cells: Skeletal muscles are unique in that each fiber contains multiple nuclei. This characteristic allows for efficient coordination and repair of the muscle tissues.
- Voluntary control: Unlike cardiac and smooth muscle, skeletal muscles are under conscious control. This means that the brain can directly influence when these muscles contract.
It's interesting to note that skeletal muscles are highly adaptable. They can grow stronger and larger with exercise, thanks to a process called hypertrophy. Conversely, if not used, they can shrink in size—this phenomenon is known as atrophy.
Cardiac Muscle
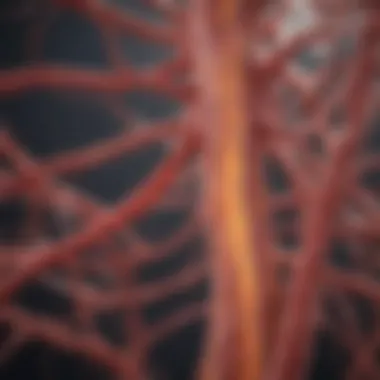
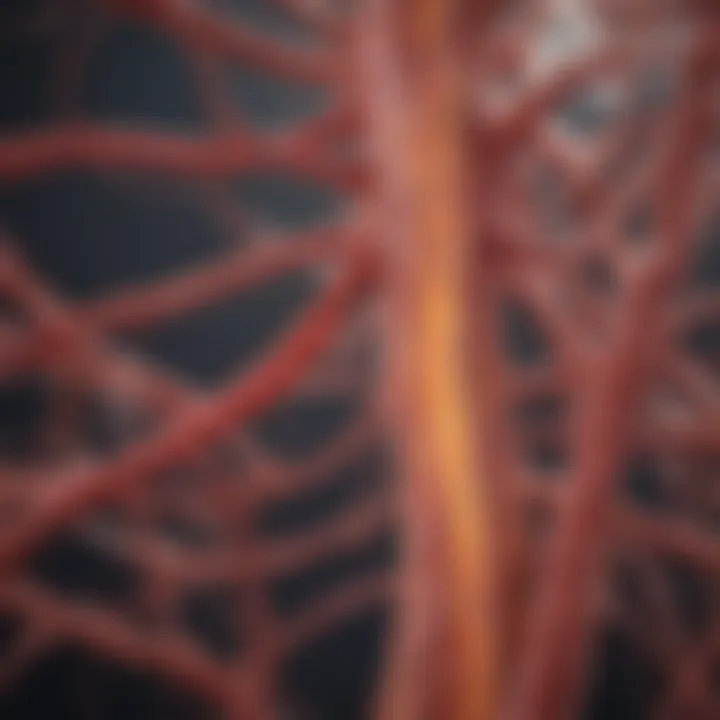
Cardiac muscle is another vital type, nestled solely in the heart. Its primary role is to pump blood throughout the body, supplying oxygen and essential nutrients to tissues. This muscle feels like a blend of skeletal and smooth muscle.
Distinct features of cardiac muscle include:
- Striated structure: Like skeletal muscles, cardiac muscles have a striated look but are branched, giving them a distinct shape.
- Single nucleus per cell: While skeletal muscles have multiple nuclei, cardiac muscle cells usually have only one. This can lead to faster transmission of impulses across the heart.
- Involuntary control: Cardiac muscles function automatically, meaning they contract without conscious effort. This autonomic property is essential for maintaining a constant heart rate and rhythm.
Cardiac muscle cells are connected by intercalated discs, which support rapid communication between cells. This is why the heart can continue to beat rhythmically even when isolated from the rest of the body.
Smooth Muscle
Lastly, we have smooth muscle, which plays a pivotal role in involuntary movements throughout the body. Found in places such as the walls of blood vessels, the digestive tract, and other internal organs, this type of muscle helps control functions like digestion and blood flow.
Important aspects of smooth muscle include:
- Non-striated appearance: Unlike skeletal and cardiac muscle, smooth muscle doesn't have a striped appearance. This smooth texture aids it in contracting in a precise and controlled manner.
- Spindle-shaped cells: The cells of smooth muscle are elongated and taper off at both ends, which contributes to their ability to contract efficiently.
- Invoulntary control: Smooth muscle works outside of our conscious awareness. For example, you cannot will your blood vessels to constrict or your stomach to churn, yet these processes happen constantly.
"Smooth muscles are like the unsung heroes of the body, quietly working to maintain vital processes without fanfare."
The function of smooth muscles can be influenced by various factors including hormones and local chemical signals, allowing the body to adapt to changing conditions.
Through understanding these three types of muscle tissue, we lay the groundwork to comprehend how muscle contractions occur and impact our health. Each type of muscle has specific roles that contribute to the overall functionality of the body, especially in the context of movement and stability. By recognizing the differences and similarities among skeletal, cardiac, and smooth muscle, we appreciate the intricate dance of biology that supports our every action.
Anatomical Structure of Muscles
The anatomical structure of muscles is crucial to understanding how they function during contraction. It delves into the intricate makeup of muscle fibers and the way they are organized. This knowledge is not just for the academic exercise; it lends insight into many practical applications, including physical therapy, sports science, and the treatment of muscle-related disorders.
Muscle Fibers and Myofibrils
Muscle fibers are the building blocks of all muscle types, fundamentally responsible for contraction. Each fiber is a long, cylindrical cell, often multinucleated, which distinguishes it from other cell types. Inside these fibers, we find myofibrils, which are long, thread-like structures packed with the protein filaments actin and myosin. These proteins are essential for the contraction process.
- Actin is a thin filament that forms the backbone of muscle contraction.
- Myosin is a thicker filament that interacts with actin, causing contraction.
These myofibrils are organized into functional units called sarcomeres. Each sarcomere is bounded by Z-discs and is the basic contractile unit of muscle cells. They work in unison, shortening simultaneously to create movement and, therefore, the essential contraction of muscles. Understanding the relationship between muscle fibers and myofibrils offers key insights into how muscle contraction occurs at the most fundamental level.
"The contraction of a muscle is initiated by an electrical impulse that triggers the release of calcium ions, fundamentally involving the interaction of myosin and actin."
In more familiar terms, think of muscle fibers as individual strings on a guitar. Each string works on its own but comes together with the others to create a harmonious sound. This harmony contributes to the overall functionality of muscles in the human body, whether it’s lifting weights or simply maintaining posture.
Arrangement of Muscle Fibers
The arrangement of muscle fibers can greatly influence their function. Muscles can have different architectures, each ideal for specific actions. There are two primary arrangements: parallel and pennate.
- Parallel muscles have fibers aligned parallel to the line of action. This structure allows for greater range of motion but sacrifices force production.
- Pennate muscles, which resemble feathers, can be further divided into unipennate, bipennate, and multipennate. They offer a higher force output due to the increased number of fibers packed into the same space.
Muscle architecture not only dictates the potential for force production but also reflects how effectively a muscle can contract. In simple terms, a pennate arrangement might be akin to a compact car; it's smaller, denser, and can accelerate quickly. Meanwhile, a parallel arrangement might represent a long-hauler vehicle, capable of covering distances but less potent in terms of raw power.
In summary, the anatomical structure of muscles, including both the muscle fibers and their arrangement, plays a pivotal role in the overall capacity for contraction. Understanding these structures helps bridge the anatomical and functional aspects of muscle, leading to better insights into health, movement, and potential therapeutic strategies.
Biochemical Pathways in Muscle Contraction
The biochemical pathways involved in muscle contraction are fundamental for understanding how muscles operate at a molecular level. These pathways illustrate the intricate interactions that facilitate movement and highlight the cooperation between various cellular components. By delving into these biochemical processes, we uncover the essential roles of energy substrates, signaling molecules, and enzymatic reactions involved in contraction. This knowledge is not only crucial for academics and healthcare professionals but also has implications for athletic performance and rehabilitation.
Role of ATP in Muscle Contraction
Adenosine triphosphate, commonly referred to as ATP, serves as the primary energy currency within muscle cells. ATP is indispensable in muscle contraction, fueling the actions necessary for movement. Each time a muscle fiber contracts, ATP is hydrolyzed, releasing energy that powers the myosin heads during the cross-bridge cycle. Without adequate ATP supply, muscle contraction is hindered significantly.
To emphasize its importance, it can be summarized as follows:
- Energy Supply: ATP is broken down into adenosine diphosphate (ADP) and inorganic phosphate, leading to the release of energy.
- Reactivation of Myosin Heads: The energy released is critical for resetting the myosin heads so they can attach to actin and continue the contraction process.
- Calcium Pump Functionality: ATP is also essential for pumping calcium ions back into the sarcoplasmic reticulum, which helps muscle fibers relax after contraction.
In short, ATP is the lifeblood of muscle activity – without it, muscle fibers would be unable to contract efficiently, if at all.
Calcium Ions and Muscle Function
Calcium ions play a pivotal role in muscle function, acting as a key signaling molecule that triggers the contraction process. During muscle activation, when a motor neuron is stimulated, an influx of calcium ions occurs through voltage-gated channels. This rise in calcium concentration initiates a cascade of events:
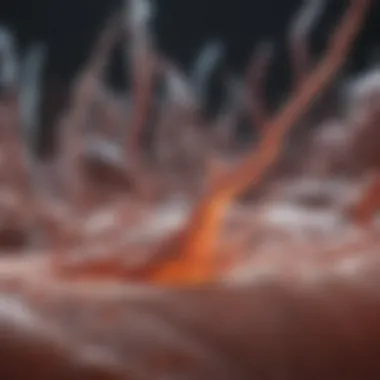
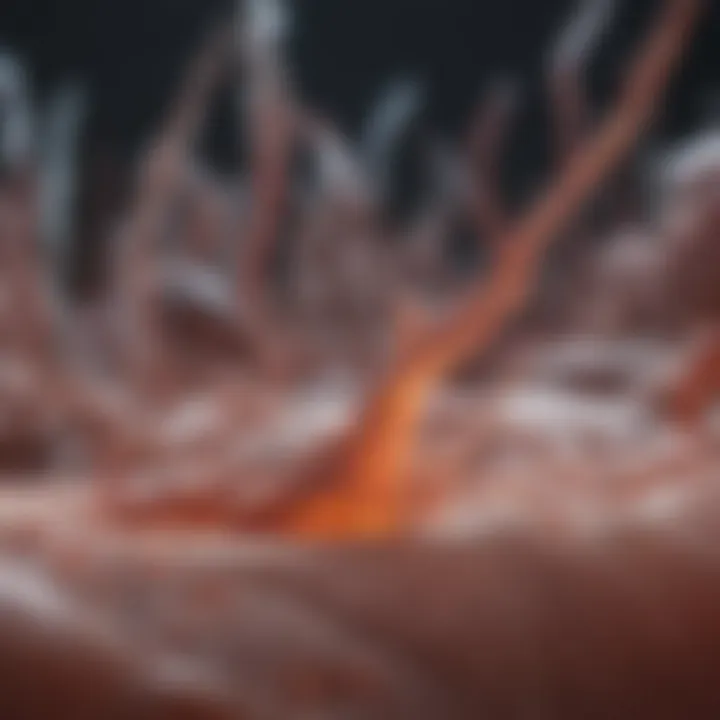
- Binding to Troponin: Calcium ions bind to the troponin complex on the actin filament, causing a shape change.
- Exposure of Binding Sites: This conformational change shifts tropomyosin and exposes the binding sites on actin, permitting myosin heads to attach.
- Contraction Mechanism: The binding of myosin to actin leads to the power stroke of myosin, resulting in muscle shortening and contraction.
Moreover, when the contraction signal ceases, calcium ions must be rapidly pumped out of the cytoplasm to restore muscle relaxation. Hence, the proper regulation of calcium ions is crucial for efficient muscle contraction and overall muscle health.
"Calcium is not just a mineral; it’s the maestro conducting the symphony of muscle contraction."
Cross-Bridge Cycle
The cross-bridge cycle is the fundamental mechanism through which muscle contraction occurs. This cycle illustrates the interactions between the myosin heads and actin filaments that dictate when and how muscles contract. It can be broken down into several distinct phases:
- Attachment: Myosin heads, in a high-energy state, bind to actin, forming a cross-bridge.
- Power Stroke: The release of ADP and inorganic phosphate causes the myosin head to pivot, pulling the actin filament toward the center of the sarcomere – a process referred to as the power stroke.
- Detachment: New ATP molecules bind to myosin, causing myosin to release from the actin filament, thus detaching the cross-bridge.
- Hydrolysis and Re-cocking: The split of ATP into ADP and phosphate reactivates the myosin head, placing it back in a high-energy position, ready to bind to actin again.
This cycle continues as long as ATP and calcium ions are present. The ability of the muscle to sustain contraction is largely determined by the efficiency and speed of the cross-bridge cycling, which varies between different muscle types.
In summary, the biochemical pathways in muscle contraction highlight a finely tuned interplay between various molecules like ATP and calcium ions. Each component is vital for ensuring muscles function smoothly, underlining the complexity and elegance of muscle physiology.
Neurological Control of Muscle Contraction
Understanding the neurological control of muscle contraction is not just a matter of academic interest. This process is fundamental to how our bodies operate daily, right from the moment we get out of bed until we settle down for the night. The nerves are like the electrical wires of our body, sending signals essential for movement. Without these signals, our voluntary and involuntary movements would come to a standstill. Understanding this relationship can enhance our knowledge not only of muscle contraction but also of overall health and wellness.
Motor Neurons and Their Role
Motor neurons are the key players in the dance of muscle contraction. These specialized nerve cells convey signals from the central nervous system to muscles, allowing for precise movements. To paint a clearer picture, when you decide to raise your arm, your brain sends an electrical impulse down through the spinal cord to the motor neurons, which then relay the message to the muscles in your arm. The span of influence from one motor neuron can be quite significant, as each neuron can control multiple muscle fibers simultaneously, thus orchestrating complex motions.
Effective communication between the brain and muscles is vital for seamless movement.
When motor neurons malfunction due to injury or disease, the consequences can be severe—think of conditions like amyotrophic lateral sclerosis (ALS) where voluntary muscle control is compromised. Understanding motor neurons and their functioning can enable researchers and clinicians to devise better therapeutic strategies and rehabilitation methods for affected individuals.
Neuromuscular Junction
At the intersection of nerve and muscle lies the neuromuscular junction, a fascinating yet intricate structure. This specialized synapse plays a vital role in translating neuronal excitement into muscle action. When a motor neuron reaches the end of its axon, it releases a neurotransmitter called acetylcholine. This chemical messengers binds to receptors on the muscle membrane, triggering a cascade of events leading to muscle contraction.
The neuromuscular junction can be thought of as the interface between intention and movement. If you imagine a relay race, the neuromuscular junction is the baton hand-off that translates the runner’s intention into action. Any disruption in this process can lead to dysfunction, as seen in diseases like myasthenia gravis, where the body’s immune system attacks the receptors and leads to muscle weakness.
Central and Peripheral Nervous System Interactions
The central and peripheral nervous systems work hand in glove when it comes to muscle contraction. The central nervous system, comprising the brain and spinal cord, serves as the command center, while the peripheral nervous system extends the reach of that command to the muscles. This elegant interdependence allows us to react swiftly to our environment.
- Central Nervous System: Integrates sensory information and sends motor commands.
- Peripheral Nervous System: Couples the commands to the muscles, enabling responses ranging from delicate finger movements to powerful leg kicks.
Interactions between these systems can be complex. For instance, when the brain processes a stimulus like a hot surface, it sends immediate signals to withdraw your hand before you even realize the pain. This reflexive action is a testament to the rapid communication channel established between the central and peripheral nervous systems.
As we delve deeper into this topic, we uncover a world where every muscle twitch is the result of an incredible ballet of signals orchestrated by the nervous system.
Factors Influencing Muscle Contraction
Understanding the factors that influence muscle contraction is akin to grasping the intricacies of a finely tuned machine. Muscle contraction is not just a matter of signals being sent from the brain; it involves a complex interplay of various elements. Recognizing how these factors interact helps in diagnosing conditions related to muscle function and contributes significantly to fields like sports medicine and rehabilitation.
Physical Factors
Physical factors that affect muscle contraction encompass a range of mechanical and environmental elements. The condition of the muscle—such as its length-tension relationship—is pivotal. When a muscle is at an optimal length, it can generate maximal force. If the muscle is either too stretched or too short, the force output diminishes. Other physical factors include:
- Temperature: Muscles tend to contract more efficiently at warmer temperatures, which is why warming up before exercise is crucial.
- Fatigue: Prolonged or intense activity strains the muscle fibers, reducing their ability to contract effectively due to the depletion of energy reserves.
- Hydration: Sufficient hydration regulates electrolyte balance, essential for muscle function. Dehydration can lead to cramping and decrease overall performance.
Maintaining a keen awareness of these physical factors not just helps athletes, but also informs clinicians working on muscle rehabilitation.
Chemical Factors
The cocktail of chemicals that involves muscle contraction cannot be overlooked. The primary player is the presence of ions, notably calcium and sodium. When it comes to muscle contraction:
- Calcium Ions: Calcium ions are crucial to the contraction process. They bind to specific proteins (troponin and tropomyosin) and enable the interaction between actin and myosin, the two proteins that facilitate muscle contraction.
- ATP (Adenosine Triphosphate): Often referred to as the energy currency of the cell, ATP is needed for muscle contraction and relaxation. Without sufficient ATP, muscles cannot properly contract or recover.
Chemical imbalances, such as those seen in metabolic disorders, can dramatically alter muscle function and performance, highlighting the need for balance in chemical levels.
Hormonal Influences
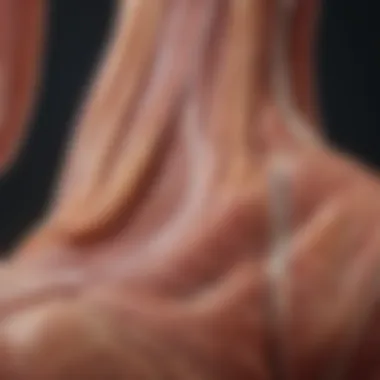
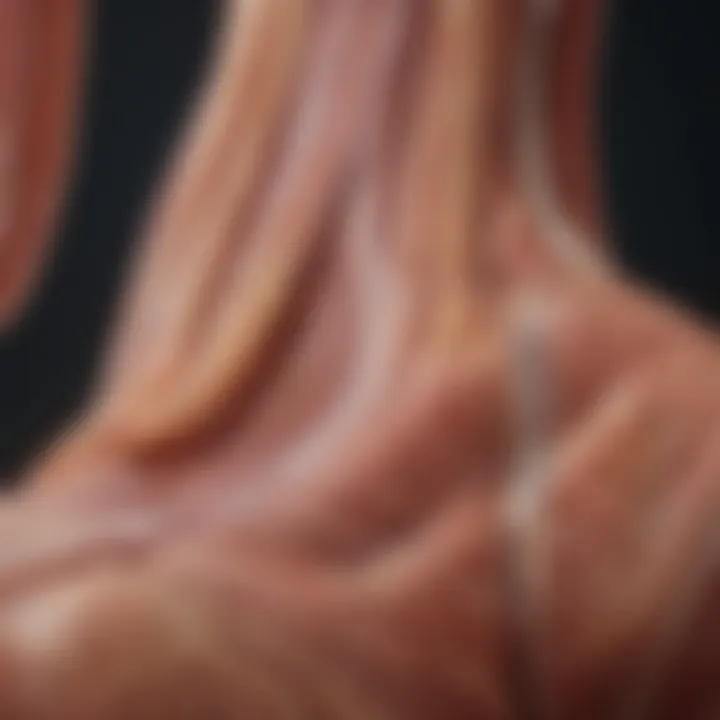
Hormones play essential roles in regulating muscle contraction. Different hormones influence not just growth and metabolism but also the effectiveness of muscle contractions:
- Testosterone: This hormone is vital for muscle growth and development. It stimulates protein synthesis, which leads to muscle strengthening.
- Cortisol: While it is necessary for various body functions, excessive cortisol can hinder muscle growth and recovery, especially in high-stress conditions.
- Insulin: Often underrated, insulin is crucial for muscle contraction. It facilitates glucose uptake in muscle cells, which is critical during periods of exertion.
The flood of hormones impacts muscle performance in multifaceted ways; understanding these interactions is vital for those in health and fitness sectors attempting to optimize muscle function.
"The ability of muscles to contract is not solely dependent on the nerve supply but on a multitude of influencing factors that range from chemical solutions to physical conditions."
Through examining physical, chemical, and hormonal influences, we not only enhance our understanding of muscle physiology but also empower individuals in athletic training, rehabilitation, and beyond. This holistic approach to muscle contraction underscores its dynamic nature, encouraging ongoing research and professional development in the field.
Pathophysiology of Muscle Contraction
Understanding the pathophysiology of muscle contraction is pivotal in comprehending how various disorders can introduce dysfunction in the muscular system. Muscle contraction does not merely involve the mechanical aspect of fibers moving but also integrates biochemical, neurological, and physical elements that are susceptible to a range of pathological conditions. Recognizing these intricacies enables deeper insights into how muscle disorders manifest and affect overall health, guiding research and potential treatments.
Muscle Disorders and Their Impact
Muscle disorders come in many shapes and sizes, affecting people differently depending on their unique physiological makeup. Conditions such as muscular dystrophy, myasthenia gravis, and rhabdomyolysis illustrate the diversity of muscle-related diseases. These disorders can impede normal muscle contraction—leading to muscle weakness, fatigue, or even complete loss of muscle function. For instance:
- Muscular Dystrophy: This genetic condition gradually weakens muscles over time; it disrupts the production of proteins needed for healthy muscle life.
- Myasthenia Gravis: An autoimmune disorder where antibodies interfere with neuromuscular transmission, causing extreme fatigability.
- Rhabdomyolysis: This severe condition occurs when muscle fibers break down at an alarming rate, often due to physical trauma or stress.
The repercussions of such disorders are profound, impacting not just physical capabilities, but also psychological well-being. Individuals may encounter difficulties in daily activities, leading to frustrations and a diminished quality of life.
"Every movement we make is a testament to the intricate design of our musculature; disruption can lead to significant life challenges."
Nerve Damage and Muscle Contraction
The interplay of nerves and muscles is crucial. Any damage to the nervous system can remarkably affect muscle contraction, and conditions such as peripheral neuropathy or spinal cord injuries exemplify this interaction. For example, when nerves that control muscle movements are injured, signals may fail to reach the muscle fibers, thus hampering contraction. This can lead to:
- Muscle Atrophy: Failure of the muscle to receive proper signals results in wasted muscle mass over time.
- Spasticity or Flaccidity: Damage can lead to either unintended muscle tightness or a complete lack of muscle tone.
Nerve damage may stem from a variety of sources—trauma, diseases like diabetes, or even environmental factors like toxins. Such factors complicate the ability to perform basic movements and can make therapies and rehabilitation more challenging.
Overall, a comprehensive understanding of muscle contraction and its pathophysiology creates a foundation not just for academic exploration, but also for clinical intervention and the development of therapeutic strategies.
Recent Advances in Muscle Research
When it comes to understanding muscle contraction, recent advances in research are changing the landscape. These new methods and discoveries not only deepen our comprehension of basic physiological processes, but they also hold promise for addressing various muscle-related conditions. The cutting-edge techniques being developed help to visualize and manipulate muscle tissues in ways that were previously thought to be unattainable. With this increased knowledge, implications for therapies and treatments regarding muscular disorders are becoming clearer.
Innovative Techniques in Studying Muscle Contraction
Innovative tools are breaking new ground in the realm of muscle research. For instance, researchers are utilizing advanced imaging techniques, such as live-cell microscopy and super-resolution microscopy. These allow for real-time observation of muscle fibers contracting. By watching how proteins interact within the muscle cells, scientists can pinpoint specific mechanisms behind muscle contraction. This real-time analysis leads to greater insight into what happens during various muscle-related activities.
Furthermore, technologies such as optogenetics are emerging. This technique allows researchers to control muscle contractions with light. It involves genetically modifying specific muscle cells to express light-sensitive proteins, enabling manipulation of their action in a minute and controlled fashion. This precise control has opened up a forum for investigations into how muscle fibers respond to diverse stimuli, providing substantial steps towards understanding the complexities of muscular behavior.
- Key Technologies:
- Live-cell microscopy
- Super-resolution microscopy
- Optogenetics
The combination of these techniques results in a robust toolkit for scientists, making it easier to dissect the multifaceted world of muscles, and bolstering our understanding of various disorders.
Genetic Research and Muscle Function
Genetic research has also taken muscle studies by storm. It shines a light on how changes in specific genes can affect muscle function, development, and contraction. For example, scientists are exploring gene editing technology, such as CRISPR-Cas9, to investigate how to correct genetic mutations that lead to muscular dystrophies and other inherited conditions. By targeting genes responsible for muscle fiber formation and repair, researchers are can grasp vital clues on how to enhance muscle regeneration.
Additionally, studies focusing on the myokines, which are cytokines produced by muscle cells during contraction, are gaining traction. Understanding how these substances interact with the rest of the body creates another layer to our overview of muscle function. For example, they play critical roles in metabolism and inflammatory responses, showing just how interconnected muscle health is with overall bodily functions.
The End
In summation, the study of muscle contraction weaves through a tapestry of various biological processes that impact both individual health and our understanding of systemic physiology. This article highlights how intricate and multifaceted muscle contractions are, revealing not only the anatomical and biochemical pathways, but also the neurological oversight that governs them. From the moment a motor neuron fires to the point where muscle fibers shorten through the cross-bridge cycle, countless factors coalesce to produce movement.
Summary of Key Points
- Definition and Significance: Muscle contraction serves as a cornerstone for movement, underlining its relevance in everything from everyday activities to advanced athletic performance.
- Types of Muscle Tissue: Understanding skeletal, cardiac, and smooth muscle tissues broadens insights into how different muscles function and the distinct roles they play in the body.
- Biochemical Pathways: The role of ATP, calcium ions, and the cross-bridge cycle is instrumental in comprehensively understanding muscle mechanics.
- Neurological Control: Motor neurons, neuromuscular junctions, and their interactions with both the central and peripheral nervous systems underscore the importance of communication within the physiological framework.
- Pathophysiology: Recognizing how muscle disorders and nerve damage can interrupt these processes provides valuable context and emphasizes the delicacy of the muscular and nervous system.
Implications for Future Research
The exploration of muscle contraction opens numerous pathways for further investigations. As research technology advances, areas such as the genetic basis of muscle function and regenerative medicine promise to be particularly fruitful.
- Genetic Research: Identifying specific genes linked to muscle function and contraction could lead to novel treatment avenues for muscle-related diseases.
- Innovative Techniques: Emerging techniques in imaging and biotechnology allow us to observe muscle function in ways never before possible, enhancing the core understanding of muscle dynamics.
- Interdisciplinary Collaboration: There lies potential in collaborations across disciplines, merging insights from neurology, biochemistry, and even materials science to innovate clinical applications or sports performance enhancements.
Overall, as we deepen our grasp of muscle contraction mechanisms, the implications for health, performance, and rehabilitation strategies continue to grow, shedding light on numerous avenues yet to be explored.