Understanding Electromagnetic Shielding Effectiveness
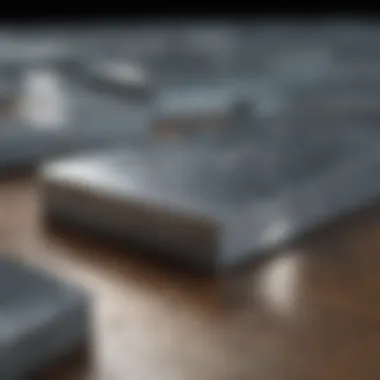
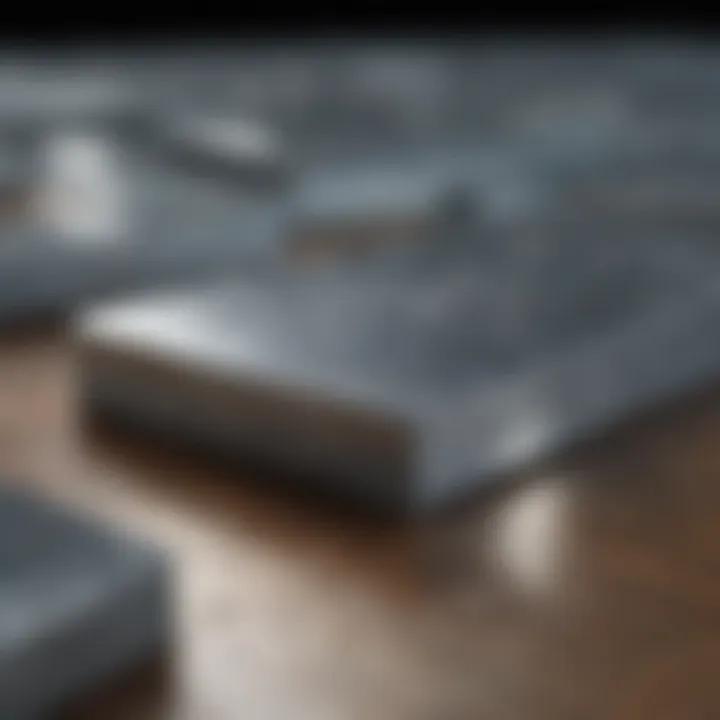
Intro
Electromagnetic shielding serves as a crucial barrier in today's technology-driven world, protecting sensitive electronic equipment from external electromagnetic interference (EMI). This article aims to carefully navigate the realms of electromagnetic shielding effectiveness, focusing on its principles, applications, and the various measurement techniques employed to assess its efficacy. The growing dependency on technology has amplified the importance of understanding how different materials can mitigate the disruptive impacts of EMI.
One might wonder why this topic holds such significance. As we venture deeper into an era dominated by digital communications, wireless networks, and intricate electronic devices, the risk of interference increases. This interference can compromise device performance, leading to data corruption, loss of functionality, and, in severe cases, complete system failure. Therefore, unraveling the complexities of electromagnetic shielding is not merely an academic exercise but a practical necessity for researchers, developers, and engineers.
This exploration will also cast light on the rapidly evolving technologies and material sciences that are reshaping the landscape of electromagnetic protection. By understanding the fundamental principles, assessing the tools available for measurement, and comparing contemporary findings with past research, the target audience—comprising students, researchers, and professionals—will be equipped to face the challenges posed by EMI.
Let’s embark on this journey now, starting with methodologies that underpin effective electromagnetic shielding.
Understanding Electromagnetic Interference
Understanding electromagnetic interference (EMI) is fundamental for comprehending how electromagnetic shielding operates effectively. This section aims to deconstruct the nature of EMI, its sources, and its implications on technology. With devices and systems becoming increasingly complex, the clarity surrounding EMI is critical. Identifying its origins and understanding its impacts allows for the creation of better shielding solutions, paving the way for innovation in electronics, medical devices, and communication technologies.
Definition of Electromagnetic Interference
Electromagnetic interference refers to the disruption caused by electromagnetic radiation emitted from an external source, affecting the performance of sensitive electronic devices. To put it simply, it's like unwanted noise interrupting a conversation. This interference can manifest in various forms—ranging from radio wave disruptions in communications systems to distorted images on a TV screen. The importance of recognizing EMI lies in its ability to impact functionality, reliability, and efficiency across numerous devices, making it an essential concern in the modern technological landscape.
Sources of Electromagnetic Interference
Natural Sources
Natural sources of EMI include phenomena such as lightning strikes, solar flares, and cosmic radiation. These sources exemplify the unpredictability of nature, where even a simple thunderstorm can interfere with radio frequencies. Lightning, for instance, generates broad-spectrum EM energy that can hinder communications. What’s interesting about natural sources is their inherent unpredictability; no matter how advanced our technology becomes, we can't control these environmental factors. They pose both risks and challenges in designing resilient systems, as one cannot simply ignore their existence; understanding them is vital for effective shielding strategies.
Artificial Sources
Artificial sources of EMI arise from human-made devices like televisions, microwaves, and cell phones. Usually, these sources emit significant electromagnetic fields that can interfere with the operation of nearby electronics. The telecommunications sector, in particular, has seen a surge in such sources with the advent of smart devices. What makes artificial sources significant is their controllability; unlike natural sources, they can be managed or mitigated. Their predictable nature allows researchers to design products and solutions that effectively limit interference, ensuring that devices remain operational even in close proximity to potential EMI sources. However, as they multiply in our everyday lives, the challenge of ensuring adequate shielding becomes more pronounced.
Impact of EMI on Modern Technology
Understanding the ramifications of EMI on various technological sectors is paramount as it influences device performance and user experience. Here, we’ll observe three key areas affected by EMI: digital devices, medical equipment, and telecommunication.
Digital Devices
Digital devices are particularly sensitive to EMI, as they rely on precise signals for smooth operation. For example, the performance of smartphones can degrade significantly in high EMI environments. Known for their intricate circuitry, digital devices can easily pick up interference, leading to problems like dropped calls, erratic performance, and a poor user experience. Hence, ensuring adequate electromagnetic shielding is crucial to maintaining functionality in these devices, which have become ubiquitous in our everyday lives.
Medical Equipment
In the realm of healthcare, EMI can pose severe risks, especially for devices like pacemakers and imaging systems. A small electromagnetic disturbance can disrupt the accuracy of scans or even lead to malfunction in life-saving devices. As such, the imperatives for shielding in medical applications extend beyond typical robustness; they demand utmost reliability. Specialists need to devise shielding solutions that not only protect against interference but also ensure that essential medical devices function optimally at all times.
Telecommunication
In the telecommunications sector, the fight against EMI is ever-present. Networks rely heavily on stable signals for data transmission. Interference can lead to disruptions that affect voice quality, data speed, and overall service. Telecommunication companies invest significantly in limiting EMI to secure clear communication channels. Moreover, as technology advances and bandwidth increases, the strategies to mitigate EMI must adapt, thus ensuring that users receive uninterrupted service.
"Understanding the sources and impacts of EMI is the first step toward developing effective shielding strategies."
Principles of Electromagnetic Shielding
Understanding the principles of electromagnetic shielding is paramount for effectively combatting electromagnetic interference (EMI) in various fields. This domain not only serves as the foundation for modern electronic design, but it also plays a crucial role in ensuring the reliability and functionality of devices across industries. By grasping the fundamental mechanisms, professionals can select appropriate materials and configurations, ultimately leading to enhanced performance and safety in technology. The key elements of this section focus on mechanisms of shielding, definitions of shielding effectiveness, and factors influencing this effectiveness.
Mechanisms of Shielding
Shielding mechanisms can be broadly categorized into two primary types: reflective shielding and absorptive shielding. Each of these methods has its own unique characteristics that contribute to their effectiveness in reducing EMI.
Reflective Shielding
Reflective shielding operates on the principle of reflecting incoming electromagnetic waves away from the protected area. One standout characteristic of reflective shielding is its ability to work efficiently over a broad range of frequencies. Materials such as metals—copper and aluminum—are commonly employed in this context due to their high conductivity. Reflective shielding is often favored because it is relatively less expensive compared to absorptive methods.
However, there’s a unique feature to consider; while reflective shielding is effective against certain frequencies, it may not suffice against low-frequency EMI generated by natural sources like lightning. This limitation highlights the importance of selecting the right shielding approach based on the specific EMI environment encountered. Thus, understanding these dynamics is vital for determining whether reflective shielding is advantageous for a given application.
Absorptive Shielding
Absorptive shielding, in contrast, functions by absorbing electromagnetic waves instead of deflecting them. This method relies on materials designed to convert EMI into heat, effectively neutralizing it. A key characteristic of absorptive shielding is its capacity to manage low-frequency interference, which is particularly beneficial in environments with persistent, disruptive signals. For example, specialized polymers or composite materials that incorporate absorptive properties could be a game-changer in certain applications.
One unique feature of absorptive shielding is its versatility. It can be engineered to serve specific frequency ranges or types of interference. Although it may be more expensive than reflective alternatives, the investment can pay off in terms of performance, especially in cases where EMI levels are exceptionally high. The choice between reflective and absorptive methods hinges on various factors, including budget, application, and the nature of the EMI.
Shielding Effectiveness Defined
Shielding effectiveness quantifies how well a given shielding material attenuates electromagnetic fields. This measure is typically expressed in decibels (dB), representing the ratio of incident power to transmitted power. A higher dB value indicates better shielding performance. In the context of electromagnetics, understanding this concept is critical for evaluating any shielding solution's performance.
This metric not only influences material selection but also shapes design decisions from the very start of a project. Materials that exhibit high shielding effectiveness are often preferable, but an optimal balance between cost-effectiveness and performance must be struck to align with project requirements.
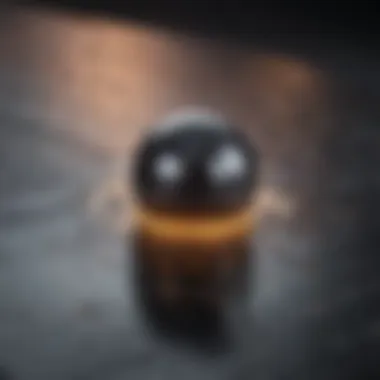
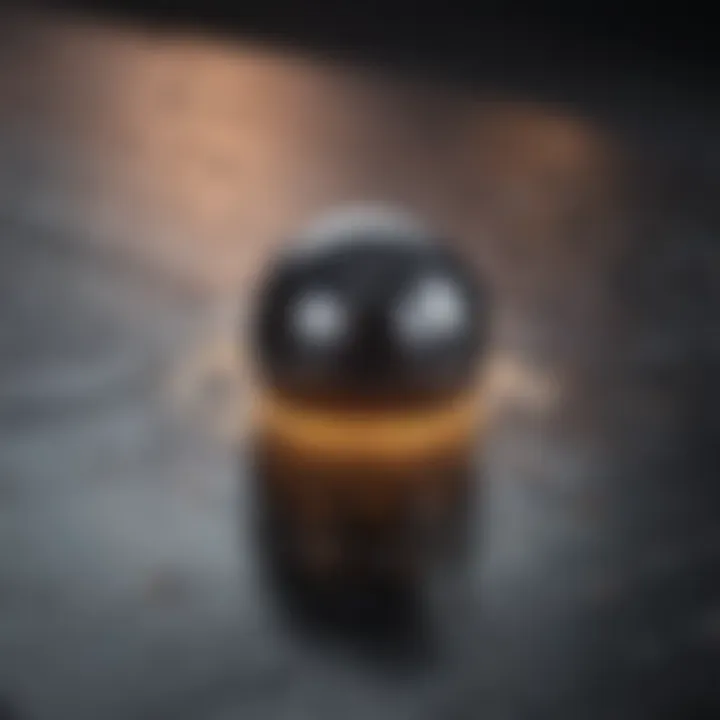
Factors Affecting Shielding Effectiveness
Several key factors influence the effectiveness of electromagnetic shielding, each deserving exploration. Understanding these factors allows practitioners to optimize their shielding strategies based on empirical evidence and applied science.
Frequency of Interference
The frequency of the electromagnetic interference plays a significant role in determining shielding effectiveness. Higher frequency signals often demand different materials and geometries compared to lower frequencies. A fundamental characteristic here is that many materials exhibit varied levels of effectiveness at different frequencies. For instance, conductive metals like copper excel at higher frequencies, but their performance can wane at lower frequencies.
This factor encourages a tailored approach in shielding design. Understanding the specific interference frequency enables manufacturers to select materials that optimize performance while reducing unnecessary costs. Balancing cost, complexity, and shielding needs is critical for successful implementation.
Material Properties
The base properties of materials utilized for shielding affect not only their performance but durability as well. The conductivity, permeability, and thickness of the chosen material significantly influence its effectiveness. For example, copper is known for its exceptional conductivity, making it an excellent choice for many applications.
However, the choice of material can also intertwine with economic considerations. Some materials may provide comparable performance yet come at a lower price point, offering viable alternatives in budget-sensitive scenarios.
Geometric Configuration
The geometric configuration of a shielding system holds notable implications for its performance. The orientation, spacing, and layout of shielding materials can either enhance or hinder effectiveness. For example, overlapping seams may increase protection against EMI compared to simple butted seams.
An additional layer of complexity arises in designs that involve multiple layers of materials. Layer configurations can be strategically employed to maximize electromagnetic attenuation. This geometric influence underscores the necessity for thoughtful design in shielding implementation.
Materials Used for Electromagnetic Shielding
Understanding the materials utilized for electromagnetic shielding is pivotal for ensuring efficient protection against electromagnetic interference (EMI). Different materials offer unique characteristics, impacting their effectiveness in various applications. Knowing the strengths and weaknesses of these materials can aid in selecting the appropriate shielding solution, enhancing device reliability and performance across technology spaces.
Conductive Materials
Copper
Copper is one of the most effective materials in electromagnetic shielding, largely due to its excellent electrical conductivity. This property allows it to reflect electromagnetic waves effectively, making it a popular choice for enclosures and connectors. Its main advantage lies in its ability to form barriers that can disrupt the flow of EMI. One unique feature of copper is its antimicrobial properties, which can be beneficial in medical device applications. However, the downside to copper is its susceptibility to corrosion, which can limit its lifespan in certain environments.
Aluminum
Aluminum presents another robust option for electromagnetic shielding. It is lighter than copper, offering a distinct advantage in applications where weight is a critical factor, such as in aerospace. The metal’s high reflectivity and resistance to corrosion make it suitable for outdoor enclosures. One of aluminum's unique features is its malleability, allowing for various shapes and forms in design. However, while it can be effective for moderate shielding needs, it does not match copper's conductivity levels, which might be a concern in high-interference environments.
Steel
Steel is often utilized in industrial settings for electromagnetic shielding. Its strength and durability add a layer of protection against physical impacts, making it ideal for robotic enclosures and heavy machinery. The key characteristic of steel is its density, granting it excellent absorption capabilities of electromagnetic waves. What sets steel apart is its widespread availability and cost-effectiveness compared to other conductive materials. However, the weight of steel can be a disadvantage in applications where mobility is essential.
Non-Conductive Materials
Carbon-Loaded Plastics
Carbon-loaded plastics are becoming increasingly popular in shielding applications due to their mix of lightweight and effective EMI shielding properties. These materials combine traditional plastic with carbon fillers to enhance conductivity while maintaining ease of use. The key advantage is their versatility, as they can be molded into complex shapes suitable for various applications, from consumer electronics to automotive parts. However, their effectiveness often falls short in high-frequency scenarios compared to metals, making it essential to assess their limitations carefully.
Specialized Coatings
Specialized coatings are applied to surfaces to enhance their shielding capabilities without adding significant weight. These coatings can be formulated from a variety of materials, including conductive polymers and metal-filled coatings. Their main advantage is that they can easily be applied to existing objects, upgrading their performance without drastic redesigns. A unique feature is their adaptability – coatings can be tailored for specific frequencies and applications. However, the durability of these coatings under harsh environmental conditions can be a concern, and they might require regular maintenance to ensure their effectiveness over time.
Hybrid Shielding Materials
Hybrid shielding materials combine the advantages of both conductive and non-conductive materials to create comprehensive solutions. They offer a unique approach by integrating multiple layers, enhancing performance without compromising on weight or cost. This versatility allows for applications in critical fields such as aerospace and military, where reliable shielding is paramount. The challenge lies in the complexity of designing these hybrid solutions to balance effectiveness, cost, and manufacturability, making them suitable for a diverse range of industries.
Measurement Techniques for Shielding Effectiveness
Understanding how to measure shielding effectiveness is fundamental for researchers and professionals involved with electromagnetic shielding. This section explores the various measurement techniques utilized to evaluate how well different materials can protect against electromagnetic interference (EMI). Selecting the appropriate measurement method is crucial as it greatly impacts the accuracy of the results and the subsequent decision-making regarding material and design choices.
Standard Testing Methods
When it comes to measuring shielding effectiveness, adhering to standardized testing methods is essential. Two prominent standards in this domain are IEEE and MIL-STD, each serving unique purposes and industries.
IEEE Standards
The Institute of Electrical and Electronics Engineers, or IEEE, has developed guidelines that provide rigor in measuring shielding effectiveness. One key characteristic of these standards is their focus on replicable and consistent testing environments. They often emphasize parameters such as frequency ranges and measurement protocols, which ensures a utility that is widespread across various electronic applications. The accessibility of IEEE standards is one of its strengths; many laboratories worldwide adhere to them, making it a familiar reference for engineers.
A unique feature of the IEEE standards is the flexibility they afford in terms of material types and configurations. This adaptability allows for evaluations under diverse conditions, from consumer electronics to industrial components. However, while these standards are advantageous for providing a structured approach, they can sometimes be criticized for being too broad, potentially leading to ambiguity in specific applications.
MIL-STD Standards
Conversely, MIL-STD standards are primarily used in military and aerospace applications. A significant aspect of these standards is their rigorous requirements, designed to ensure performance under extreme conditions. This makes them invaluable for defense-related projects where high reliability is paramount. Users adopting MIL-STD often appreciate the detailed specifications for different shielding scenarios, which enhances trust in their outcomes.
The unique feature of MIL-STD is its stringent criteria for durability and resilience. These standards often take into account various factors such as temperature, humidity, and mechanical stresses. However, this rigidity can also be a double-edged sword, as the stringent testing procedures can sometimes be impractical for commercial products or smaller-scale projects.
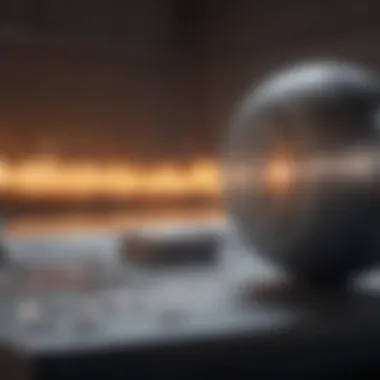
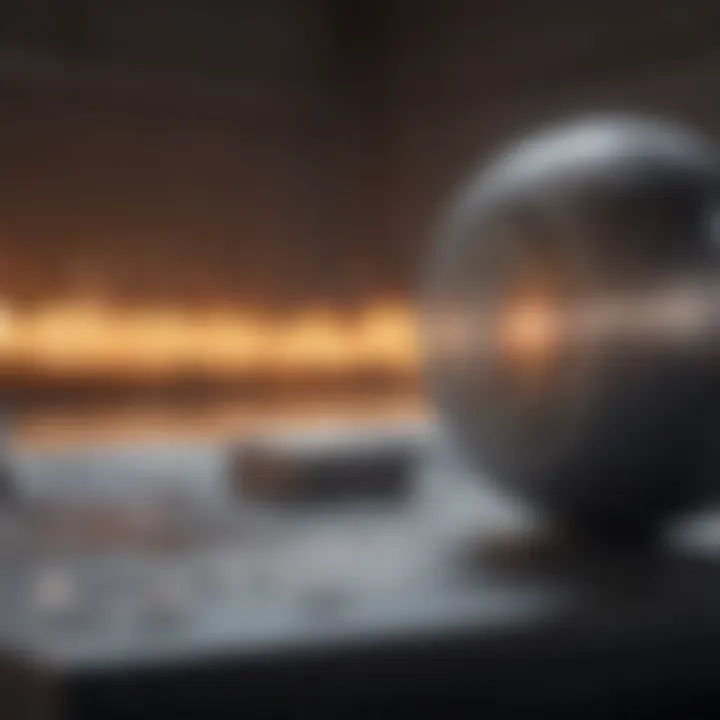
Experimental Techniques
In addition to standard methods, experimental techniques provide insights through hands-on measurement. Two prominent approaches are near-field scanning and far-field measurements, each with its own set of methodologies and outcomes.
Near-Field Scanning
Near-field scanning is a technique that utilizes a probe to measure the electromagnetic fields in close proximity to the shielding material. One of its standout characteristics is its ability to provide detailed spatial information regarding the electric and magnetic field distributions. This granularity can be incredibly beneficial for identifying weak spots in the shielding effectiveness of a material.
Near-field scanning allows for rapid results and can be performed in various environments, making it a popular choice among researchers in both academic and commercial settings. However, the downside lies in its potential complexity. This method often requires sophisticated equipment and expert interpretation of the data, which may not be readily available in all laboratories.
Far-Field Measurements
Far-field measurements, on the other hand, involve assessing the shielding effectiveness at greater distances from the source. One of the primary advantages of this method is its simplicity and ease of execution, making it a go-to for many applications where a broad overview of the shielding performance is sufficient.
A unique feature of far-field measurements is that they are less sensitive to the specific geometries of the shielding and can provide an overall average effectiveness. Yet, this advantage comes at a cost; these measurements may overlook subtle inefficiencies that could be critical in specialized applications, such as in high-frequency devices or environments with complex EMI challenges.
Simulation Methods
Simulation methods offer a complementary approach to experimental measurements. They allow for predictive assessments of how materials will behave under different electromagnetic conditions. The two notable methods are the Finite Element Method (FEM) and Boundary Element Method (BEM).
Finite Element Method
The Finite Element Method (FEM) is an advanced computational technique that breaks down complex geometries into smaller, manageable elements. One key characteristic of FEM is its ability to model the physical properties of materials and simulate how they respond to electromagnetic fields accurately. This method is particularly beneficial for optimizing designs before physical prototypes are developed, resulting in cost and time savings.
FEM's unique feature lies in its versatility; it can be applied to various scenarios, including frequency-dependent behaviors. However, this complexity requires significant computing power and expertise, which may be a barrier for some users.
Boundary Element Method
On the other hand, Boundary Element Method (BEM) focuses specifically on the boundaries of materials, significantly reducing the computational load compared to FEM. One advantage of BEM is that it offers precise calculations for problems with infinite domains, making it effective for understanding radiation and scattering phenomena.
However, while BEM is efficient, it may not handle complex volumetric interactions as robustly as FEM. This limitation means users must understand their specific shielding challenges to choose the most appropriate simulation method.
"Selecting the right measurement technique for electromagnetic shielding involves understanding the trade-offs between precision, complexity, and applicability to the specific materials or devices involved."
Through these diverse measurement techniques, one gains insight into both the theoretical and practical aspects of electromagnetic shielding. As technology advances and materials evolve, an ongoing commitment to improving measurement methods is essential for staying at the cutting edge of this vital field.
Applications of Electromagnetic Shielding
The role of electromagnetic shielding cuts across various sectors, proving invaluable in maintaining the functionality of sensitive equipment in the face of electromagnetic interference (EMI). Each application not only enhances device efficacy but contributes to safety and regulatory compliance. Understanding these applications is crucial for academics and professionals as technology becomes increasingly integrated into daily life.
Consumer Electronics
In consumer electronics, features such as smartphones and laptops heavily rely on effective shielding to prevent disruptions caused by external electromagnetic waves. Without proper shielding, devices would suffer from poor performance, data loss, or even complete failure.
- Importance: Shielding ensures that users enjoy uninterrupted service while using devices.
- Design Considerations: Components like housing cases or circuit boards often incorporate conductive materials like copper or aluminum. The thickness and configuration of these materials are reflected in their ability to block interference effectively.
- Regulatory Compliance: Many countries enforce strict EMI regulations to protect consumers. Manufacturers must meet these requirements to bring their products to market, making design and material choice essential.
Military and Aerospace
The military and aerospace sectors present unique challenges where electromagnetic shielding plays a pivotal role. With equipment operating in diverse environments and extreme conditions, the need for reliability cannot be overstated.
- Significance: Communication systems, navigation tools, and radar technologies depend critically on shielding to ward off EMI from both natural and artificial sources. Even slight interference could result in severe operational failures, leading to mission risks.
- Material Choices: Specialized materials, such as stainless steel and composite alloys, are often tailored for specific applications like aircraft skins or electronic warfare systems. They not only shield against EMI but also withstand harsh conditions, adding to their durability.
Healthcare Devices
Within the healthcare domain, electromagnetic shielding serves as a lifeline. Medical equipment, from MRI machines to patient monitoring systems, must function without interference to ensure patient safety and treatment efficacy.
- Essential Role: EMI could skew data readings or, worse, compromise a device's ability to deliver accurate information about a patient's condition. For instance, an ECG monitor's reliability hinges on undisturbed signals for precise diagnosis.
- Shielding Strategies: Many healthcare devices employ multi-layered shielding approaches using both conductive and non-conductive materials to enhance protection without exposing patients or operators to electrical hazards.
Automotive Industry
The automotive sector is witnessing a paradigm shift towards smarter vehicles integrated with numerous electronic systems. As cars become increasingly dependent on technology, shielding has become more critical than ever.
- Impact on Functionality: Modern vehicles now utilize electronic control units (ECUs) for everything from engine management to advanced driver-assistance systems (ADAS). If these systems were not shielded appropriately, electromagnetic noise could lead to malfunctions, jeopardizing both driver and passenger safety.
- Innovative Techniques: Manufacturers are turning towards innovative materials such as carbon-loaded plastics, which provide shielding capabilities while also being lightweight. This trend not only enhances performance but can also lead to better fuel efficiency and lower emissions.
"In the rapidly evolving landscape of technology, the effectiveness of electromagnetic shielding is undoubtedly crucial for ensuring the seamless operation of devices across all sectors."
Each sector's specific needs highlight how crucial it is to stay ahead of advancements in materials and technology. Understanding these applications is a step in advancing the dialogue on electromagnetic shielding effectiveness and its future direction.
Challenges in Electromagnetic Shielding
Understanding the challenges faced in electromagnetic shielding is crucial for anyone involved in designing or working with electronic devices. These obstacles often dictate how effective a shielding solution can be, ultimately impacting both technology performance and user experience. The intricacies behind these challenges can often be overlooked, but addressing them is vital for advancing the field of electromagnetic compatibility. In this section, we will explore three primary challenges: material limitations, design constraints, and environmental factors, each contributing to the overall effectiveness of shielding.
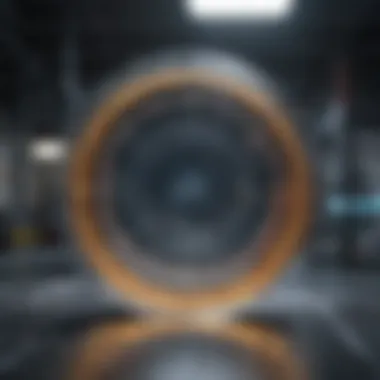
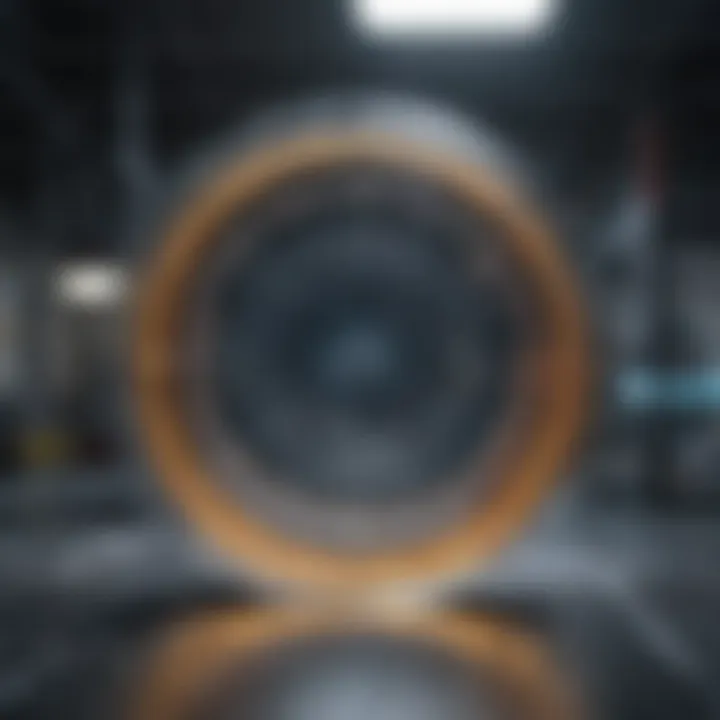
Material Limitations
Material selection plays a keystone role when it comes to electromagnetic shielding. Each type of material has its own set of properties, strengths, and weaknesses. For instance, while copper offers excellent conductivity, it may not be practical for all applications due to its weight and cost. Similarly, lighter alternatives like aluminum might be more cost-effective but do not provide the same level of attenuation, particularly at lower frequencies.
In certain cases, even the composition of alloys can affect shield performance. Non-conductive materials, like carbon-loaded plastics, can provide some level of shielding, but tend to be less effective when compared to metals. Furthermore, material degradation over time due to environmental stressors can also diminish the shielding characteristics.
“Your choice of material does not just determine performance but can also make or break your design’s longevity.”
Design Constraints
The design of electromagnetic shielding is not a walk in the park. Numerous factors come into play, including geometric configuration, thickness, and layering. Any oversight in design could result in unforeseen gaps or weaknesses, allowing electromagnetic interference to seep through. For example, a poorly designed enclosure might create unintended apertures, compromising the shielding effect.
Moreover, the integration of shielding into existing structures can present additional hurdles. Designers must balance aesthetic considerations with functional requirements, which can lead to compromises that affect the efficacy of the shielding. Additionally, cost constraints might force teams to choose suboptimal designs, which can be detrimental in sensitive applications, like in medical devices or aerospace technology.
Environmental Factors
Environmental conditions can serve as both a friend and foe to electromagnetic shielding. High humidity, temperature fluctuations, and exposure to corrosive substances can impair the shielding's effectiveness over time. This deterioration occurs silently, making it difficult to assess when a shield has become compromised.
Moreover, the presence of external electromagnetic fields, such as those from communication towers or industrial equipment, can overwhelm inadequately shielded devices, leading to operational failures. A shielding solution that works well in a lab setting may falter in the field due to these real-world variabilities.
In navigating these challenges, researchers and engineers must continually innovate. Deeper understanding and proactive solutions are necessary for maximizing the potential of electromagnetic shielding.
By addressing these challenges, we engage in not only improving current technologies but also paving the way for future advancements in electromagnetic compatibility.
Future Trends in Electromagnetic Shielding
Electromagnetic shielding has come a long way since its inception, with new developments continually shaping its effectiveness. As technology marches forward, it’s essential to stay tuned to emerging trends that hold promise for enhancing shielding materials and techniques. These trends not only address current challenges but also push the boundaries of what is possible in electromagnetic interference (EMI) management. By embracing advancements in nanotechnology, material science, and smart technologies, we can address the ever-increasing demands for effective shielding solutions across various sectors.
Nanotechnology in Shielding Materials
Nanotechnology represents a significant leap in the field of electromagnetic shielding. The incorporation of nanoparticles into traditional shielding materials like metals can drastically improve their performance. Using materials such as carbon nanotubes can lead to higher conductivity and lower weight, which is crucial for applications in aerospace and consumer electronics. The small scale of these materials allows them to exhibit properties not found in bulk materials, creating a new avenue for enhancement.
Benefits of Nanotechnology:
- Enhanced Performance: Nanostructured materials exhibit superior shielding effectiveness due to their increased surface area.
- Lightweight Solutions: The reduction in weight can transform sectors like automotive and aerospace, where every gram reduction matters.
- Flexibility: Incorporating nanomaterials can lead to more adaptable designs suited for a variety of applications.
The use of nanotechnology isn't just about adding new materials; it's about rethinking how we approach shielding. For instance, graphene-based materials are coming to the forefront, displaying promising results in EMI shielding while being eco-friendly.
Advancements in Material Science
The exploration into advanced materials is a game-changer in the quest for effective electromagnetic shielding. Innovations such as metamaterials, which are engineered to have properties not found in natural materials, can be designed specifically to target certain frequencies of electromagnetic waves. This level of customization has the potential to vastly improve shielding effectiveness beyond traditional methods.
Moreover, ongoing research is examining how hybrid materials can combine the best traits of both conductive and non-conductive materials.
Considerations in Material Science Advances:
- Combining Properties: Hybrid materials can offer both shielding and thermal management, helping in designs where heat dissipation is also a concern.
- Scope for Innovation: Constant material innovations push the envelope, driving capabilities in various applications, from medical devices to telecommunications.
These advancements not only enhance performance but also provide new options for professionals designing components for environments challenged by EMI.
Integrating Smart Technologies
With the rise of the Internet of Things (IoT) and smart technologies, integrating EMI shielding solutions into smart devices is becoming a necessity. Smart technology is often susceptible to interference due to its reliance on wireless communication. The importance of implementing robust shielding around these devices cannot be overstated, as it ensures functional resilience and reliability.
Benefits of Smart Technology Integration:
- Real-time Monitoring: Incorporating sensors that monitor EMI levels in real-time can enable dynamic adjustments to be made to shielding effectiveness.
- Self-regulating Systems: Future smart systems can potentially self-adjust their shielding mechanisms based on the types of interference detected nearby.
By merging shielding solutions with smart technologies, we are setting the stage for more resilient devices that can operate even in the most challenging electromagnetic environments.
"The future of electromagnetic shielding lies not just in the materials we use but in how we integrate these materials with today's advanced technology."
Ending
In summarizing the intricate landscape of electromagnetic shielding effectiveness, it becomes evident that this subject is both timely and essential. The role of electromagnetic shielding goes beyond just blocking unwelcome signals; it serves as a vital component in ensuring the performance of numerous technologies across various sectors. The importance of shielding lies in its ability to protect sensitive equipment from electromagnetic interference (EMI), which, if left unchecked, can wreak havoc on the operational integrity of devices ranging from consumer electronics to critical healthcare instruments.
Recap of Key Points
As we reflect on the discussions throughout the article, several key points emerge:
- Understanding EMI: We began by examining what electromagnetic interference entails, identifying its natural and artificial sources and emphasizing its significant impact on our tech-dependent lives.
- Shielding Mechanisms: The principles behind electromagnetic shielding are multifaceted. We discussed both reflective and absorptive shielding, shedding light on how these mechanisms work together to mitigate interference.
- Material Matters: Different materials play crucial roles in the effectiveness of electromagnetic shielding. From conductive materials like copper and aluminum to innovative non-conductive solutions such as carbon-loaded plastics, each material has unique properties that contribute to shielding performance.
- Measurement Techniques: We explored the various measurement techniques for evaluating shielding effectiveness. Standard testing methods, experimental approaches, and simulations provide practitioners with a robust toolkit for assessing their shielding solutions.
- Real-world Applications: The applications of electromagnetic shielding are far-reaching—from consumer electronics to aerospace and medical devices—signifying its essential role in modern technology.
- Challenges and Trends: Lastly, we touched on the challenges faced in this field, including material limitations and design constraints, while also considering promising trends such as nanotechnology and smart technology integration that are shaping the future of electromagnetic shielding.
Implications for Future Research
The discussion around electromagnetic shielding effectiveness opens up a myriad of avenues for future exploration. As technology continues to evolve, the following considerations will likely shape ongoing research and development:
- Nanotechnology: Advancements in nanomaterials could lead to groundbreaking improvements in shielding capabilities and material strength, paving the way for lighter and more efficient designs.
- Sustainable Solution: Research into environmentally friendly and recyclable materials could provide an avenue to address the pressing concerns related to electronic waste while still achieving robust shielding performance.
- Smart Shielding Technologies: Integrating shielding materials with smart technologies could facilitate adaptive responses to varying electromagnetic conditions, enhancing the protection offered in dynamic environments.
- Cross-disciplinary Collaboration: Future breakthroughs may arise from collaboration between fields like material science, electrical engineering, and computing, fostering innovation in shielding techniques and materials.
In summary, the ongoing exploration into electromagnetic shielding effectiveness will not only enhance our ability to safeguard technology but also usher in a new wave of innovative solutions in the quest for improved electromagnetic compatibility.