Understanding Electrical Hertz: Its Impact and Applications
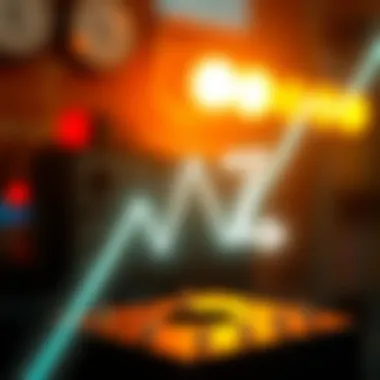
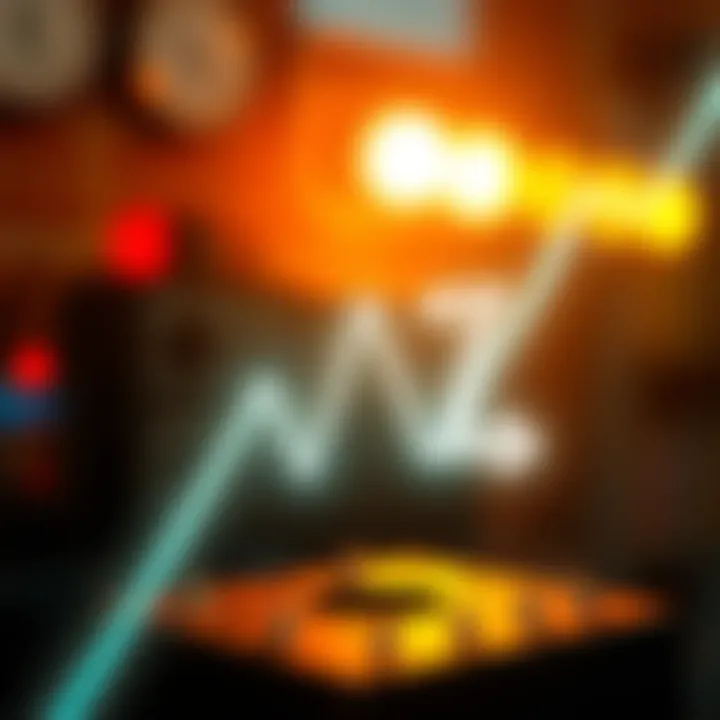
Intro
In the dynamic world of electricity and electronics, understanding the concept of hertz is crucial. The term hertz, abbreviated as Hz, is a standard unit of frequency that quantifies cycles per second. But this isn't just a number; it lies at the heart of numerous technologies that define modern life. Whether it’s the hum of an electric appliance, the transmission of signals through the air, or the synchronization of systems, hertz influences them all.
Preface to Electrical Hertz
A fundamental understanding of electrical hertz is critical not only for students diving into the depths of electrical engineering but also for veterans navigating the complexities of modern technology. The term "hertz," which is a measure of frequency, finds its roots deeply embedded in both historical and practical aspects of physics and electrical engineering. Its significance can’t be overstated; hertz is the heartbeat of oscillating systems. Whether you're talking about the buzzing of an electric current or the rhythm of radio waves, the hertz is what sets the pace.
Understanding hertz helps illuminate many underlying principles that govern electrical systems. Without grasping the implications of frequency, one might ponder endlessly over why circuits behave as they do or how signals traverse distances and maintain clarity. This section aims to highlight just how crucial knowledge of hertz is in everything from circuit design to consumer electronics.
Definition and Fundamental Principles
At its core, hertz measures the number of cycles per second of a periodic phenomenon. This concept may sound simple, but it is anything but. The beauty of hertz lies in its ability to describe a multitude of phenomena, from the flicker of a light bulb to the sound waves emitted from a speaker. In electrical engineering, 1 hertz equates to one cycle of electromagnetic activity or alternation in a second. This means if an electrical signal oscillates at 60 hertz, it’s completing 60 cycles every second.
The direction and behavior of these cycles also matter. For instance, in alternating current (AC) systems, the alternating nature of the current needs careful understanding to avoid accidents and ensure efficiency. Thus, studying hertz blankets a wide array of principles essential to understanding system behaviors.
Historical Context and Development
The historical underpinnings of hertz recall a journey of discovery and innovation. The term hertz is actually named after Heinrich Hertz, a German physicist who made pioneering contributions to electromagnetic waves in the late 19th century. His experiments laid the groundwork for future developments in radio transmission, inspiring generations of engineers and scientists to explore the electromagnetic spectrum.
Interestingly, the adoption and standardization of hertz as a unit of frequency came much later, around the mid-20th century. This period saw a surge in technological advancements, and the need for a consistent unit for measuring frequency became apparent. Organizations like the International System of Units had a profound influence in this regard, helping usher in a new age of clarity and standardization in technology. Understanding the history of hertz, then, is not just about remembering a name; it’s about acknowledging our technological evolution and the insightful minds that have contributed to it.
Mathematical Representation
Mathematics plays a crucial role in understanding electrical hertz, focusing on the relationship between frequency and time, which form the backbone of various electrical systems. Grasping these mathematical concepts helps in predicting behaviors and outcomes of circuits and signals. Engineers and scientists rely on accurate calculations to design and optimize systems, ensuring reliability and efficiency.
Understanding Frequency and Hertz
The term frequency refers to how often a repeating event occurs within a specific timeframe. When we mention hertz (Hz), we're talking about a unit that measures this frequency, where one hertz equals one cycle per second. Imagine a light bulb that flickers: if it flashes on and off 60 times per second, its frequency is 60 Hz. It’s not just about blinking lights; it applies to sound waves, radio signals, and just about everything that revolves around periodic phenomena.
In electrical systems, understanding frequency is paramount. For instance, the frequency can affect the performance of circuits, impacting components like capacitors and inductors. If an electrical system operates at a frequency outside its optimal range, it can lead to inefficiencies or even failure. The design decisions engineers make, based on frequency considerations, can significantly influence the final product.
It’s also worth mentioning that frequency impacts how we perceive audio and video. Higher frequencies yield sharper images and clearer sounds, while lower frequencies may result in more muted or dull outcomes. Thus, not only does frequency shape our electrical systems, but it also plays an integral part in our everyday experiences with technology.
Formula Derivation and Calculations
Deriving the formulas that govern frequency and hertz typically involves some elementary physics principles, such as concepts of time and periodicity. One fundamental equation is:
[ f = \frac1T ]
Where:
- f is the frequency in hertz,
- T is the period in seconds (the time it takes for one complete cycle).
This formula provides insight, showing the inverse relationship between frequency and time period; as the time taken for each cycle decreases, the frequency increases. This relationship is crucial in various practical applications, such as signal processing and communications. Engineers often find themselves manipulating this foundational equation to design systems that function efficiently under various operating conditions.
When considering alternating current (AC) circuits, the notion of impedance comes into play, and calculations can grow more complex. The relevant formulas can include not just frequency but also resistance, inductance, and capacitance. For simple calculations involving a resistive AC circuit, the formula might be expressed as:
[ Z = R + jX ]
Where:
- Z is the impedance,
- R is resistance, and
- X is reactance (which can again involve frequency).
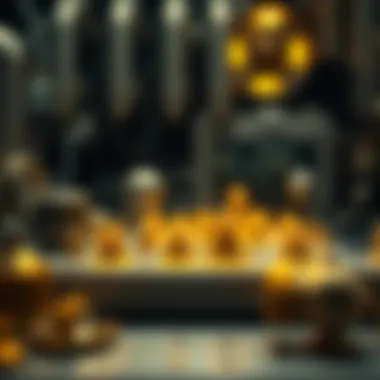
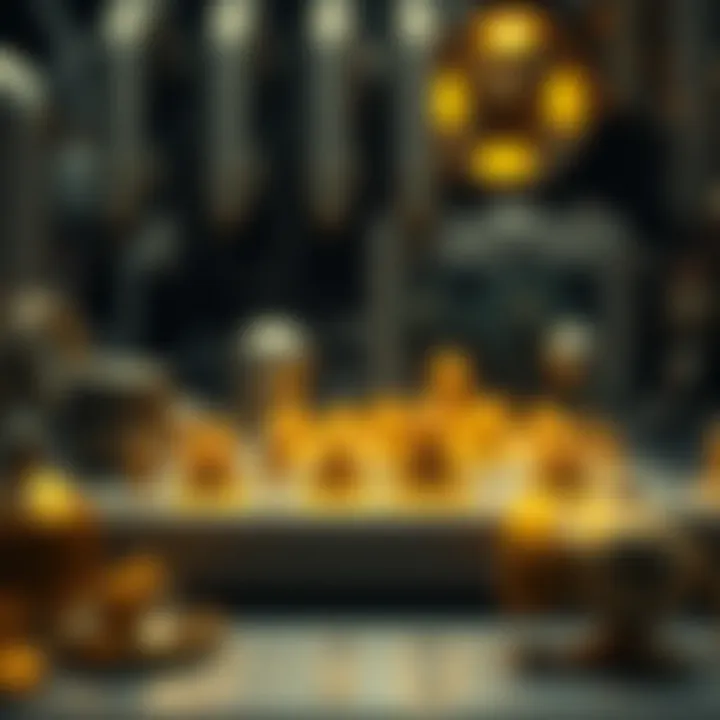
Understanding these formulas ensures that engineers can predict how electrical systems will behave under different frequencies, leading to better designs and improved performance.
"Mathematics is not just a tool; it's the language of electrical systems, speaking volumes about their frequencies and behaviors."
These mathematical representations are foundational. They serve as a guiding compass for researchers and professionals navigating through the complexities of electrical engineering, ensuring precision in design and function.
Hertz in Electrical Engineering
In the realm of electrical engineering, understanding hertz is not just crucial, it's foundational. Hertz, as a unit of frequency, dictates how circuits operate and influence the effectiveness of electrical designs. Electrical engineers leverage hertz to engineer, analyze, and optimize circuit performance, ensuring that systems function harmoniously without wasting energy or interfering with other devices. It's about making electricity perform to its fullest potential while meeting the demands placed on modern technology.
Implications for Circuit Design
When engineers sit down to design a circuit, they are fundamentally concerned with the frequencies that the circuit will encounter. The implications of hertz in circuit design stretch far and wide. Not only does it determine the crucial parameters of component selection, but it also shapes the feedback loops, filters, and even the stability of the system.
For instance, consider an audio amplifier circuit operating at a frequency of 20 kHz. An engineer must choose components that can handle signals in this range to prevent distortion and ensure high-quality sound reproduction. The choice of resistors, capacitors, and even transistors can determine whether that 20 kHz signal is amplified cleanly or ends up muddied with noise.
- Component Selection: Choosing capacitors that can handle the frequency without heating up or failing is paramount.
- Signal Integrity: Ensuring that signals do not degrade through the circuit involves calculating impedance at various hertz levels.
- Feedback Design: The stability of a circuit often comes down to the feedback frequency response. Correcting phase shifts at critical frequencies can prevent feedback loops from becoming oscillatory.
In today’s technology-driven age, where devices are interconnected, designing circuits with a fundamental understanding of frequency has become all the more critical. Just like a symphony needs accurate pitching to resonate, circuits need precise frequency handling to coexist without interference.
Frequency Modulation Techniques
Diving into frequency modulation, this technique is pivotal for enabling efficient communication systems. Frequency modulation (FM) enhances the quality of signal transmission, mitigating issues that arise from noise or interference. By altering the frequency of carrier waves, information can be embedded, allowing for clearer signals.
Looking at FM in practical contexts, consider the role hertz plays in radio broadcasting. A radio station transmits at a specific frequency — for example, 101.1 MHz. Using frequency modulation, the station can encode music or voice into variations of that frequency. This method improves resistance to static and other forms of distortion, making the listening experience far superior than when amplitude modulation (AM) is used.
Applications of Hertz
The concept of hertz extends far beyond mere definitions and calculations; it permeates various domains, influencing technologies that are vital to modern life. Understanding how hertz functions in practical applications is essential for grasping its full significance. This section emphasizes three critical areas where hertz plays a pivotal role: telecommunications and signal processing, power generation and distribution, and consumer electronics and appliances. Each of these sectors bears unique considerations which highlight the importance of frequency in ensuring efficiency, reliability, and innovation.
Telecommunication and Signal Processing
In telecommunications, hertz serves as the bedrock of transmission efficiency and clarity. The ability to transmit signals without degradation relies heavily on the frequency associated with each signal. For instance, in mobile networks, frequencies can dictate the bandwidth available, directly impacting the speed and quality of data transfer.
For instance, different mobile communication standards utilize varied frequency bands. GSM (Global System for Mobile Communications) phones typically operate on frequencies around 900 MHz or 1800 MHz. It is in this segment that understanding hertz becomes crucial; if frequencies are crowded or improperly allocated, service disruptions can occur.
Moreover, within digital signal processing, hertz can influence the clarity of audio or visual signals. Sample rates, which are expressed in hertz, determine how accurately a system can capture the nuances of sound and light. A higher sample rate allows for a more detailed reproduction of original signals, whereas lower rates may lead to potential data losses or distortions.
"The clarity of a signal is not just about the transmission medium, but also about the frequencies utilized to convey that signal effectively."
Power Generation and Distribution
In power generation, understanding hertz is equally critical. The standard electrical frequency in many countries is either 50 Hz or 60 Hz. This frequency impacts how generators and transformers are designed. For example, electric motors are often optimized to run at a specific frequency, and deviations can lead to inefficiencies or failures.
Additionally, the synchronization of power systems across regions is contingent on maintaining standardized frequencies. If one power station operates out of sync with others, this can create challenges in managing the grid efficiently. The consequences extend beyond mere operational hiccups; they can affect large populations in terms of electricity supply stability.
For example, the North American power grid operates on a frequency of 60 Hz. When fluctuations occur, the system's reliability can be jeopardized, and corrective actions must be prioritized to restore normal operation. This careful balance underscores the necessity of maintaining consistent frequencies in power distribution systems.
Consumer Electronics and Appliances
The consumer electronics sector showcases how hertz directly influences everyday devices. From washing machines to microwave ovens, the frequency components are intricately designed into their operations. Many appliances rely on AC power that aligns with the local frequency standards, making hertz essential for functionality.
Take the case of a microwave oven, which typically operates efficiently at the standard frequency of 60 Hz. The design of its circuit components needs to match this frequency for optimal performance. An improper frequency can lead to inefficient heating, potentially creating safety hazards for users.
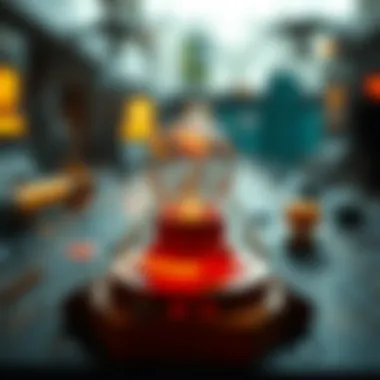
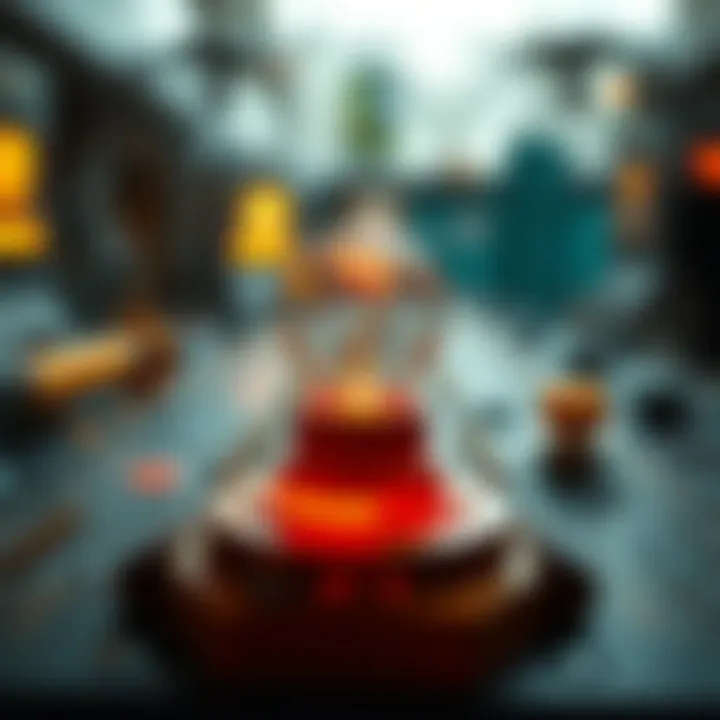
Moreover, audio and video equipment also rely on precise frequency management. For instance, speakers and amplifiers are designed to handle specific frequency ranges, and failing to adhere to these can result in poor sound quality or speaker damage.
In summary, the applications of hertz span a diverse range from telecommunications to consumer products. It is clear that the effective management of frequencies can vastly improve technology, making lives more convenient and reliable.
Impact of Frequency on System Behavior
Understanding how frequency affects system behavior is fundamental to grasping the intricate relationship between electrical signals and their performance in various applications. This section delves into critical concepts such as resonance, harmonics, and distortion. These elements not only influence system efficiency but can also determine the longevity of equipment and the quality of signal transmission.
Resonance and Its Effects
Resonance is a phenomenon that occurs when a system is driven at its natural frequency, resulting in an increase in amplitude of oscillation. Imagine a child on a swing; the greater the synchronization between the pushes and the swing's timing, the higher it goes. In electrical systems, resonance can lead to exceptionally high voltages and currents if not properly managed.
In practical terms, this can be seen in circuits like LC resonant circuits, which consist of inductors and capacitors. These components can either store energy or release it, and at resonance, the energy exchange between these elements reaches its peak. This can lead to beneficial applications, such as in radio transmission, where specific frequencies are amplified to transmit signals effectively.
However, resonance can also pose challenges. In structures like transmission lines, it can cause unwanted oscillations, ultimately leading to equipment damage or failure. Thus, understanding the conditions that lead to resonance is essential, particularly in the design process. Key considerations include:
- Component Selection: Choosing components that can handle expected frequencies and voltage levels is crucial.
- Damping Mechanisms: Adding resistors or other damping materials can mitigate excessive oscillation.
- Frequency Analysis: Conducting thorough analysis allows engineers to predict potential resonant frequencies and avoid them.
Harmonics and Distortion
Harmonics arise when a system does not operate solely at its fundamental frequency. These are higher frequency components that can distort the original signal, akin to a musical chord sounding different when out of tune. This is particularly noticeable in power systems, where non-linear loads such as rectifiers and inverters create harmonics.
For example, in a standard three-phase power system, the presence of harmonics can cause:
- Increased Heat: Higher currents flowing through transformers and motors can lead to overheating and damage.
- Reduced Efficiency: Power quality deteriorates, causing increased losses.
- Equipment Malfunction: Sensitive devices may fail to operate correctly under distorted signals, leading to costly downtime.
To address these issues, measures are often employed, including:
- Harmonic Filters: These devices can help reduce harmonics to acceptable levels, improving overall power quality.
- Regular Monitoring: Implementing systems to monitor harmonic levels can lead to early detection of potential problems.
- Design Adjustments: Modifying load configurations or power electronics can mitigate distortion risks.
"Understanding resonance and harmonics is not just an academic exercise; it's a crucial aspect of ensuring electrical systems run smoothly and efficiently."
Recognizing the impact of frequency on system behavior is essential for professionals in the field. It aids in enhancing performance, minimizing faults, and extending the lifespan of electrical systems. Engaging with these concepts not only bolsters theoretical knowledge but significantly contributes to practical, real-world applications.
Standardization and Regulations
Standardization and regulations surrounding electrical hertz play a crucial role in ensuring safety, interoperability, and efficiency across various industries. As electrical systems grow in complexity and application, having a uniform set of standards becomes essential. Not only do these standards guide manufacturers in their design and production processes, but they also provide a framework that promotes consistency in performance.
One notable benefit of standardization is the establishment of international norms that help to eliminate ambiguity. For instance, when an engineer in Japan communicates system specifications to their counterpart in Germany, relying on common standards mitigates the risk of misinterpretation due to differences in local practices. This streamlining is vital in the age of globalization, where systems and components cross borders with remarkable frequency.
Further, compliance with established standards contributes to safety in electrical systems. It is not merely about conformity but also involves rigorous testing protocols that can prevent accidents and malfunctions. The implications of not adhering to these regulations can be catastrophic, ranging from device failure to potentially life-threatening situations. Thus, continuous reinforcement and updates of these standards are necessary to address emerging technologies.
International Standards for Hertz
When we talk about international standards, organizations like the International Electrotechnical Commission (IEC) and the Institute of Electrical and Electronics Engineers (IEEE) come into play. These organizations set forth standards that help harmonize the definitions and applications of hertz globally.
For instance, one of the IEC standards focuses on the measurement of frequency in electrical systems, defining how hertz should be used and understood universally. By creating a common language, these standards facilitate better cooperation among nations and companies.
Additionally, international standards for hertz ensure that devices designed and manufactured in one country can operate seamlessly in another. This compatibility drives market growth and gives consumers access to a wider array of products.
Compliance and Safety Considerations
Compliance with these international standards is not just a box-ticking exercise; it is a critical aspect of electrical engineering that cannot be overlooked. To put it simply, when manufacturers commit to compliance, they are also committing to a set of safety benchmarks set forth by industry experts. This effort culminates in enhanced product reliability and user safety.
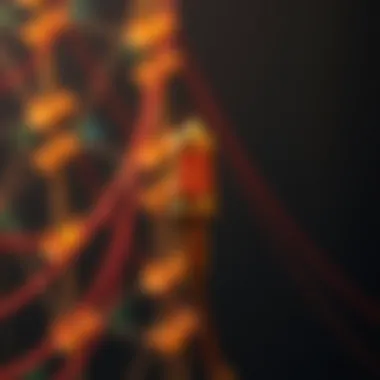
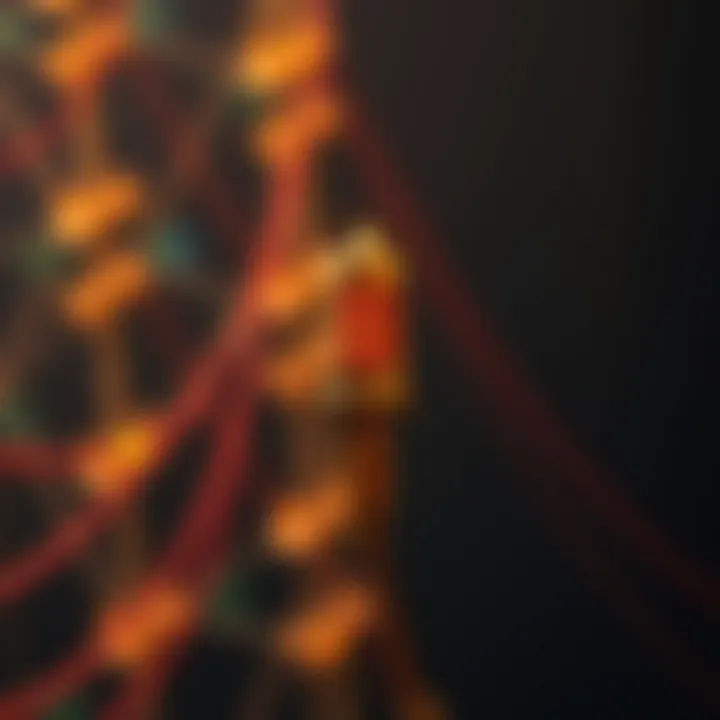
Take, for example, the safety features built into consumer electronics. Compliance with electrical safety standards means that devices go through rigorous testing for voltage fluctuations, electromagnetic interference, and thermal performance. These tests ensure that products not only work effectively but also remain safe during use, reducing the risk of hazards.
In practice, companies failing to comply with these standards may face regulatory actions, including fines or, worse, product recalls. Being out of compliance can harm a brand ’s reputation and lead to the erosion of consumer trust—a fate no business would seek.
As electrical systems become more intricate, the necessity for stringent regulations and standardization becomes more pronounced, influencing everything from design to safety to market competition.
Future Perspectives on Electrical Hertz
As we look ahead, the concept of electrical hertz gains even greater significance. With technology evolving at a breakneck pace, the role frequency plays in electrical systems is ever more critical. The upcoming trends and technologies provide a lens through which we can examine hertz's future, fostering a deeper understanding and appreciation for its implications.
Emerging Technologies and Trends
The integration of hertz in emerging technologies marks a pivotal shift in the landscape of both electrical engineering and everyday technology. 5G networks, for instance, leverage high-frequency signals, drastically improving data transfer rates and reducing latency. This advancement entails a fundamental re-evaluation of how hertz influences telecommunication.
Newly developed alternatives such as quantum computing challenge traditional paradigms. Quantum devices often operate at frequencies that require a fine-tuned understanding of hertz, as they exploit resonance states in an entirely different manner than classical systems.
In the field of renewable energy, the use of inverters to convert DC to AC power shows the importance of optimizing frequencies for maximum efficiency. Improved technology is allowing engineers to better manipulate these frequencies, reducing energy loss and enhancing the stability of the grid.
Consider these points about technology and frequency:
- Smart Appliances: Devices like smart refrigerators operate better on optimized frequency protocols, reducing energy consumption while maintaining functionality.
- IoT Devices: The Internet of Things expands the need for effective signal processing, with hertz acting as a cornerstone in ensuring seamless communication between devices.
- Medical Technologies: Advances in imaging technologies, where precise frequency applications can significantly enhance clarity and resolution.
"Emerging trends highlight a future where hertz not only defines electrical standards but alters the very fabric of our technological experience."
Challenges and Opportunities Ahead
While the vistas ahead gleam with prospects, challenges are evident. One of the foremost concerns is the increasing need for bandwidth due to the surging demand for high-speed internet and seamless connectivity. The frequency spectrum is finite, leading to potential bottlenecks in spectrum allocation.
Furthermore, regulatory hurdles and compliance standards continue to present obstacles. Different countries adopt varying hertz regulations influencing global technology deployment. Navigating these waters demands careful planning and innovation.
The conversation about hertz also encompasses sustainability. Electrical engineers are tasked with balancing frequency optimization against energy consumption, striving to devise solutions that are both efficient and eco-friendly.
To harness the opportunities, strategies must be developed, such as:
- Investment in Research: Ongoing exploration of new frequency applications across fields.
- Interdisciplinary Approaches: Collaborating with fields such as physics and environmental science can yield innovative solutions.
- Public Awareness: Educating stakeholders about the importance of maintaining frequency standards and the benefits of compliance.
As we navigate these developments, it becomes increasingly evident that the future of electrical hertz will not only shape engineering practices but will also have profound implications for the world’s technological infrastructure.
Ending
The exploration of electrical hertz concludes with an understanding of its profound importance in both theoretical and practical realms of science and engineering. As highlighted throughout this article, hertz is not just a unit of measurement; it is the heartbeat of electrical systems that underpin modern technology. By grasping the principles of frequency and its ramifications on various applications, one can appreciate how hertz shapes the functionalities we often take for granted in our everyday lives.
Summary of Key Points
To encapsulate the discussion on electrical hertz:
- Definition and Significance: Hertz, representing cycles per second, forms the basis for measuring frequency in electrical systems. Its relevance stretches across disciplines, from engineering to telecommunications.
- Historical Foundations: Understanding hertz necessitates some historical context, tracing back to innovators like Heinrich Hertz, whose work laid the groundwork for modern electromagnetic theory.
- Mathematical Implications: The relationship between frequency and system behavior is encapsulated through various formulas, echoing how intricacies of hertz impact everything from signal processing to power distribution.
- Technological Applications: In telecommunications, hertz determines bandwidth and signal quality, while in power generation, it guides the efficiency of electrical systems. Consumer electronics also rely heavily on frequency considerations for optimal performance.
- Future Trends: As technology evolves with the advent of higher frequency systems and emerging applications, challenges persist. These involve managing harmonics, ensuring compliance with international standards, and addressing safety concerns.
By delineating these key points, this article underscores that a robust understanding of electrical hertz is essential for anyone engaged in the fields of science and technology.
Final Reflections on the Role of Hertz
As we reflect on the role of hertz, it becomes clear that its importance extends well beyond mere equations and theoretical discussions. Hertz is fundamentally woven into the fabrics of electrical engineering, influencing how we design circuits, manage power, and ensure compliance with safety standards. Moreover, as we venture into an era marked by rapidly advancing technologies, the anticipated challenges and opportunities underscore the need for continuous research and adaptation.
Thus, the future of electrical hertz is not just a matter of maintaining the status quo; instead, it beckons a call for innovation and foresight. Embracing this unit of measure means embracing the future of efficient communication, innovative power systems, and ultimately, a deeper understanding of the electrical world that surrounds us.
Understanding the pulse of electrical systems through hertz offers insights that are pivotal for future advancements in technology.
As we stand on the brink of new discoveries, one can only speculate the heights to which these electrical principles will elevate us, resonating through the modern age in ways yet to be fully comprehended.