Transmission of Energy: Mechanisms and Applications
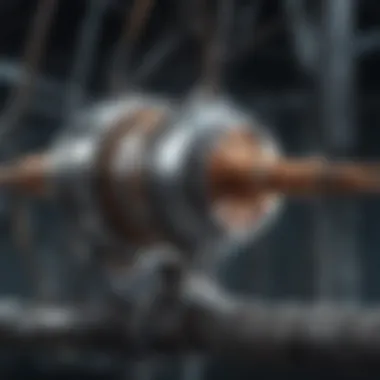
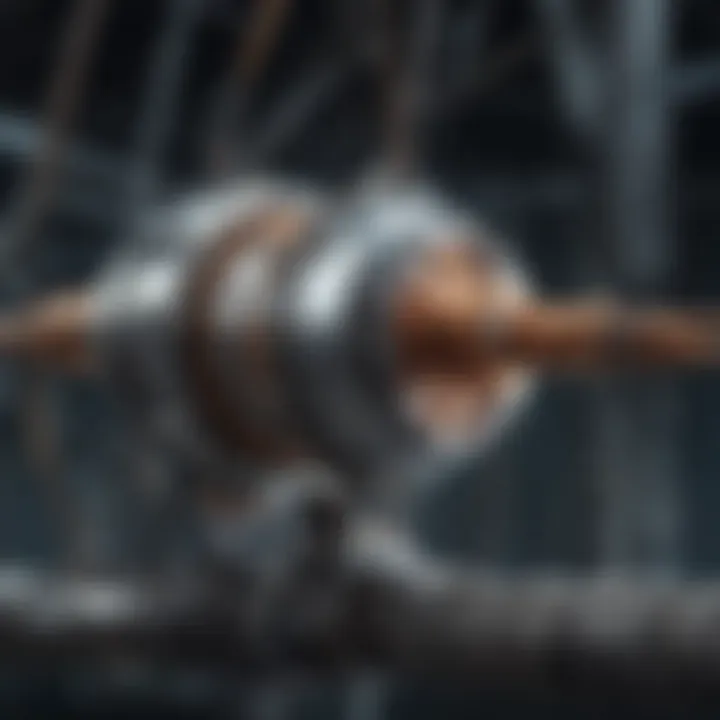
Intro
The study of energy transmission is fundamental across numerous scientific and engineering disciplines. It provides insight into how energy is moved from one point to another and the effects on various mediums. Understanding the mechanisms of energy transfer becomes crucial not only for theoretical advancements but also for practical applications in technology and industry. This article aims to break down the key elements involved in energy transmission, focusing on electrical, thermal, and mechanical processes. By exploring these concepts, readers will gain a better appreciation for the efficient transfer of energy and its implications in the real world.
Methodologies
The methodologies surrounding energy transmission involve various research techniques, designed to comprehensively examine how energy moves across different mediums.
Description of Research Techniques
Researchers primarily use experimental and theoretical methods to explore energy transmission. Experimental techniques might include:
- Laboratory experiments that simulate energy transfer in controlled environments.
- Field studies where energy transmission is observed in real-world settings, such as in power lines or thermal systems.
- Computational modeling, which utilizes simulations to predict energy transfer behavior in complex systems.
The combination of these techniques provides a holistic understanding, enabling researchers to validate theoretical models against actual data.
Tools and Technologies Used
To study energy transmission, various tools and technologies are employed. These include:
- Thermal cameras for measuring heat transfer in thermal energy systems.
- Oscilloscope for analyzing electrical signals in circuits.
- Force gauges and dynometers for mechanical energy studies.
Integration of advanced technologies enhances the precision of studies, allowing for more reliable results.
Discussion
Comparisons with previous research highlight the evolution of understanding energy transmission. Early studies focused mainly on basic principles, while recent investigations incorporate modern technology and complex modeling.
Comparison with Previous Research
Previous research laid foundational knowledge in energy mechanics. However, contemporary studies utilize interdisciplinary approaches, combining physics, engineering, and computer science.
"Understanding the mechanisms of energy transfer not only advances science but also improves technological applications vital to today's society."
The introduction of renewable energy sources, such as solar and wind, has shifted the focus towards efficient transmission methods, which were less emphasized in earlier works.
Theoretical Implications
The implications of energy transmission research extend beyond academic curiosity. They cater to practical needs, such as improving energy efficiency in systems and reducing waste, important for both economic and environmental sustainability. As energy demands continue to rise, refining these methods will be pivotal in managing resources effectively.
Prolusion to Energy Transmission
Energy transmission is a fundamental aspect of numerous scientific disciplines, underlying various technological advances and applications. Understanding how energy moves from one location to another is essential for optimizing systems that rely on the efficient transfer of energy. This section builds the groundwork for exploring concepts such as electrical, thermal, and mechanical energy transmission. It addresses how our comprehension of energy transfer impacts both scientific research and practical endeavors in our daily lives.
The relevance of energy transmission extends beyond theoretical knowledge; it affects real-world applications, from energy grids to heating systems. Efficiency in energy transmission can lead to significant economic savings and environmental benefits. Such insights contribute to advancements in technology and sustainability practices.
Defining Energy Transmission
Energy transmission refers to the process of transporting energy from one point to another, whether through cables, pipelines, or other mediums. This involves various forms such as electrical, thermal, and mechanical energy. Each type has its own set of principles and methods that dictate how effectively energy can be moved.
Understanding these definitions is crucial for both academic research and industry applications. Accurate definitions also help in communicating ideas clearly among researchers and professionals in related fields. For instance, electrical energy transmission generally involves conducting electricity through power lines, whereas thermal energy might travel through materials via conduction or convection.
Importance in Scientific Research
In scientific research, the importance of energy transmission cannot be overstated. Efficient energy transfer is vital for experiments and technologies that rely on precise conditions. The outcomes of such studies often influence how we understand energy, its sources, and implications for future innovations.
Moreover, energy transmission is integral to sustainability discussions, focusing on reducing carbon footprints and enhancing energy efficiency. Investigating various processes allows scientists to develop methods that improve transmission efficiency, whether it be through better materials or innovative designs. This research is critical for devising solutions that address pressing global challenges, such as climate change and energy crises.
"Understanding the intricacies of energy transmission enables researchers to create more effective systems, ultimately benefiting society as a whole."
While advancements in this field are crucial, they also pose several challenges. The need for collaboration across disciplines becomes apparent, blending physics, engineering, and environmental science. Therefore, research in energy transmission not only enriches academic knowledge but also holds the potential to alter industries and improve standards of living.
Types of Energy Transmission
Energy transmission encompasses various methods through which energy moves from one point to another. This section intends to dissect these methods, emphasizing their significance in both theory and application. Understanding the types of energy transmission is crucial for developing efficient systems, enhancing performance, and addressing energy needs effectively in society.
Electrical Energy Transmission
Electrical energy transmission is the most pervasive form of transmission in contemporary society. It involves the transfer of electricity from power generation facilities to end users through a network of electrical lines. This method is vital for powering homes, industries, and public services. As cities grow, the demand for electricity rises, making electrical energy transmission a pressing concern.
Several key elements highlight the importance of this transmission type:
- High Voltage Systems: Electrical transmission typically occurs at high voltages to minimize energy loss over long distances. This makes it possible to deliver power efficiently from remote generating stations, such as hydroelectric plants or wind farms.
- Grid Infrastructure: Modern power grids are complex networks that integrate various energy sources. They rely on robust technologies to ensure consistent power supply and stability. Advancements in smart grid technology enhance monitoring and control, improving overall efficiency.
Efficiency considerations also factor prominently in electrical energy transmission. Losses can occur due to resistive heating in conductors, which necessitates continued research into materials and technologies that can reduce these losses.
Thermal Energy Transmission
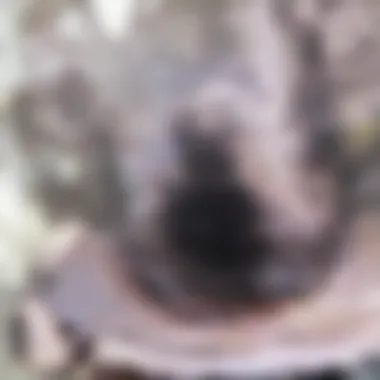
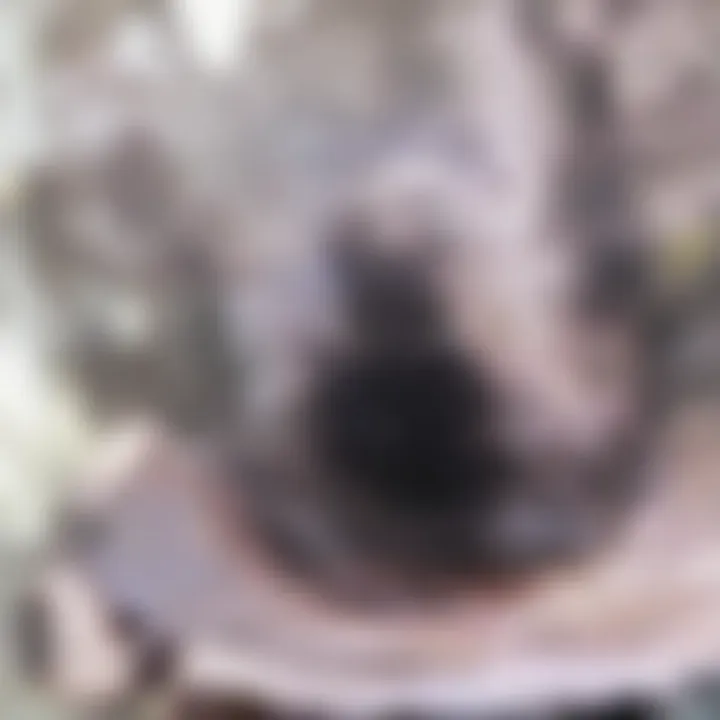
Thermal energy transmission deals with the transfer of heat between systems. This process can occur by conduction, convection, or radiation. Applications range from industrial heating systems to residential heating through radiators or underfloor heating.
Importance of thermal energy transmission includes:
- Heating Efficiency: In many applications, maximizing thermal transfer is essential. For example, in district heating systems, hot water is produced centrally and distributed to multiple buildings. Efficient thermal transmission leads to energy saving, while poor transmission can increase costs dramatically.
- Material Choices: Different materials have varying thermal conductivities which directly affect the efficiency of energy transmission. Using high-quality insulating materials can prevent unwanted heat loss, enhancing overall efficiency and sustainability.
Mechanical Energy Transmission
Mechanical energy transmission involves moving energy through mechanical systems, including machines and structures. This type of transmission is common in industries where machines convert energy forms, like in engines, turbines, and conveyor belts.
Key aspects of mechanical energy transmission are:
- Efficiency of Mechanisms: The way energy is harnessed and transmitted mechanically directly influences productivity and performance. For instance, in vehicles, the efficiency of the transmission system is crucial for fuel economy and power delivery.
- Diverse Applications: Mechanical energy transmission spans a range of uses from simple hand-operated devices to complex automated factories. Each application necessitates a specific design consideration, affected by load, speed, and operational demands.
Each of these types represents a unique segment of the broader energy transmission landscape. Understanding their differences and applications can lead to better decision-making in energy management and technological innovations.
Mechanisms of Energy Transmission
Understanding the mechanisms of energy transmission is crucial for both the theoretical study and practical application of energy systems. Each mechanism—conduction, convection, and radiation—serves as a fundamental process through which energy moves from one point to another. The efficiency of these processes directly affects energy consumption, sustainability, and integration into modern technologies. By delving into these mechanisms, we can appreciate their roles in applications ranging from home heating to advanced electronic systems.
Conduction Mechanisms
Conduction is the transfer of energy through direct contact between molecules. This process occurs when heat moves through a material, typically from the hotter to the cooler area. The efficiency of conduction is influenced by the material's properties, such as thermal conductivity. Metals, like copper and aluminum, are known for their excellent conduction capabilities, making them ideal for electrical wiring and thermal applications.
The atomic structure plays a pivotal role in conduction. In solids, tightly packed atoms allow energy to pass more easily. For instance, in context of thermal applications, knowing which materials conduct heat well can significantly improve heating systems. Materials that poorly conduct heat, like wood or rubber, are often used as insulators to minimize unwanted heat loss.
Effective conduction can lead to significant energy savings in both residential and industrial systems by reducing the energy required for heating and cooling.
Convection Processes
Convection involves the movement of fluids—in liquid or gas form—where warmer regions of the fluid rise and cooler areas descend. This process is essential in many natural and man-made systems. In weather patterns, convection currents are responsible for wind and storm formation. In heating systems, convection helps distribute warmth beyond its initial source.
The efficiency of convection increases with fluid motion. Forced convection uses pumps or fans to enhance flow, thus speeding up the energy transfer process. A practical example can be found in convection ovens, where a fan circulates hot air to cook food more evenly and efficiently.
Environmental conditions, such as temperature gradients, also impact convection. Understanding these principles allows researchers and engineers to optimize designs for better thermal management in various applications, including HVAC systems and industrial cooling.
Radiation Principles
Radiation refers to energy transfer through electromagnetic waves. Unlike conduction and convection, radiation does not require a medium; energy can travel through the vacuum of space. Sunlight, for example, is a form of radiant energy that reaches Earth, providing warmth and enabling photosynthesis.
The efficiency of radiant energy transfer depends on factors such as surface area and the emissivity of materials. Surfaces that are dark and textured tend to absorb and emit thermal radiation more effectively than smooth, shiny surfaces. This principle can be utilized in energy-efficient designs, such as solar panels, where maximizing sunlight absorption is crucial for performance.
Understanding radiation is essential for developing strategies to manage heat in environments ranging from buildings to spacecraft. Innovations in materials science continue to improve how we harness and control radiant energy for various applications.
Factors Affecting Energy Transmission Efficiency
Understanding the factors that influence energy transmission efficiency is crucial for optimizing energy systems. Efficiency impacts both the performance of energy delivery and the associated costs. Inefficient energy transmission can result in significant losses, leading to increased emissions and financial waste. Focusing on this aspect allows for the uncovering of potential improvements across various energy sectors.
Material Properties
The properties of materials used for energy transmission play a pivotal role in determining overall efficiency. These properties include conductivity, thermal resistance, and tensile strength. For instance, in electrical energy transmission, materials like copper and aluminum are preferred due to their high conductivity. Copper, in particular, has superior electrical properties but is often more expensive than aluminum.
Also, the insulation properties of materials can significantly affect heat loss in thermal systems. Insulating materials such as fiberglass or foam can minimize energy loss, thereby enhancing efficiency. Each application demands a careful analysis of material selection to meet efficiency standards while considering cost and safety.
Environmental Conditions
Environmental conditions can dramatically influence energy transmission efficiency. Factors such as temperature, humidity, and other local environmental factors should be examined. For example, high ambient temperatures can reduce the performance of electrical transmission lines, leading to increased resistance and energy loss.
Moreover, external weather conditions, like storms or high winds, can impact the stability and efficiency of mechanical systems. Understanding these variables prevents unforeseen losses and enhances system resilience. Designers and engineers must assess and adapt to potential environmental impacts when planning and constructing transmission systems.
System Design
The design of energy transmission systems has direct implications for efficiency. A well-planned system can minimize energy loss and improve reliability. Important design considerations include the arrangement of components, the length of transmission pathways, and the selection of technology.
For electrical grids, the topology should allow for optimal load distribution to prevent overheating in parts of the system. For thermal systems, efficient routing of heated fluids can minimize energy expenditure. It is essential that engineers adopt an integrated approach to system design, utilizing simulations and models to predict performance under various scenarios.
"Incorporating smart design principles can lead to significant cost savings and improved efficiency."
Considering these factors promotes advancements in energy transmission systems, leading to sustainable practices and greater overall performance.
Applications of Energy Transmission
The applications of energy transmission stand as a crucial aspect of both scientific understanding and day-to-day functionality. Efficient systems for transmitting energy facilitate modern conveniences and industry standards. Applications range from basic home heating to the elaborate energy grids that support entire cities. Understanding these applications helps to appreciate their effects on technology, economy, and the environment.
Energy Grids
Energy grids are the backbone of modern electrical supply systems. These grids manage the distribution of electricity from power plants to consumers. They consist of transmission lines, substations, and distribution networks. The efficiency of these grids directly affects energy availability and economics. Without a well-functioning grid, societies may struggle with energy shortages, outages, or considerable waste.
The development of smart grid technologies enhances monitoring and management. Advanced sensors and communication technologies allow for real-time adjustments. This integration improves energy efficiency and reliability.
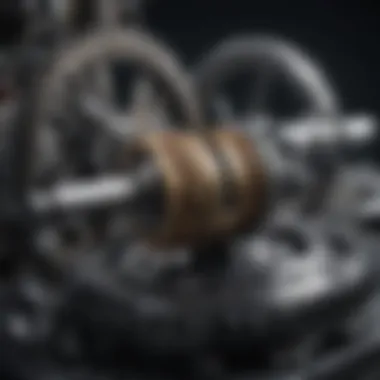
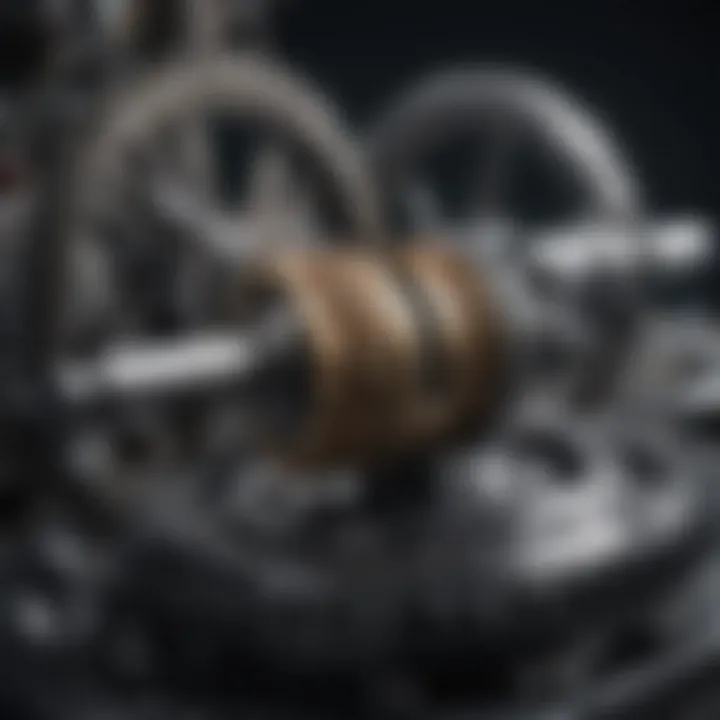
Key elements of energy grids include:
- Transmission Lines: High-voltage lines transport electricity over long distances.
- Substations: They convert high voltage to a lower voltage for delivery.
- Distribution Networks: Distribute the electricity directly to homes and businesses.
"The reliability of energy grids can affect not only economic growth but also the quality of life for residents."
Heating Systems
Heating systems utilize thermal energy transmission to provide warmth in buildings. This process can involve several methods: conduction through walls, convection using air circulation, or radiant heat. Each method has its efficiencies and considerations.
In modern infrastructures, central heating systems play a significant role. These systems often use water or steam to transfer heat from a central source to various rooms. The choice of energy source may vary, including natural gas, electricity, or renewable energy systems like solar heating.
Efficiencies of heating systems can depend on factors such as:
- Insulation: Proper insulation retains heat, reducing energy consumption.
- System Design: Optimized schematics can improve overall heat distribution.
- Energy Source: The choice of energy strongly impacts expenses and environmental footprint.
Mechanical Systems
Mechanical systems often involve the transmission of energy through physical means, such as gears, belts, and chains. These systems convert one form of energy into another, commonly from electrical to mechanical energy.
For example, electric motors convert electrical energy to rotational kinetic energy, driving machinery in factories. Industrial applications heavily rely on these systems for efficiency and productivity. Improved design and engineering can significantly enhance their performance.
Considerations in mechanical energy systems include:
- Friction: Minimizing friction increases efficiency.
- Material Selection: Choosing materials that can withstand stress while conducting energy efficiently.
- Precision Engineering: Accurate assembly ensures reliable performance and longevity.
Understanding these applications of energy transmission reveals their far-reaching implications across science and technology. Whether addressing energy security in grids, enhancing residential comfort in heating, or boosting productivity in machinery, such applications remain vital to advancing human capability.
Technological Innovations in Energy Transmission
The field of energy transmission has seen significant advancements in recent years. These innovations not only enhance the efficiency of energy distribution but also address various environmental and economic challenges. They play a crucial role in integrating renewable energy sources into existing infrastructure, which is vital in the face of rising energy demands and environmental concerns.
Smart Grid Technologies
Smart grid technologies represent a revolutionary step in energy transmission systems. By incorporating advanced communication technologies and digital controls, smart grids optimize the distribution and use of electricity.
The key benefits include:
- Increased Reliability: Smart grids can quickly detect outages and can reroute power, minimizing disruption.
- Enhanced Efficiency: Through real-time data analysis, energy load management becomes more effective, reducing waste.
- Integration of Renewables: They facilitate the incorporation of solar, wind, and other renewable sources into the grid, enhancing sustainability.
Smart grid infrastructures employ sensors and automated systems to monitor and manage the flow of energy dynamically. The shift from traditional to smart grid technology is vital for addressing the energy demands of modern society.
Wireless Energy Transfer
Wireless energy transfer is another cutting-edge innovation reshaping energy transmission. This technology enables energy to be transferred without wires, using electromagnetic fields. This is particularly beneficial in applications where traditional wiring is impractical or impossible, such as in mobile devices or electric vehicles.
Key considerations include:
- Convenience: Users can charge devices without plugging in, enhancing user experience.
- Safety: Reducing the risk of electric shocks and optimizing space by eliminating cables.
- Flexibility: Wireless systems can be installed in various locations without the constraints of physical wiring.
However, challenges such as efficiency and range are ongoing areas of research.
High-Efficiency Conductors
High-efficiency conductors are critical for maximizing energy transmission performance. Using materials with low resistance, such as superconductors, can dramatically reduce energy loss during transmission.
The benefits of high-efficiency conductors include:
- Reduced Energy Loss: Less energy is wasted as heat during transmission, making the system more efficient.
- Cost Effectiveness: While the initial investment may be higher, the long-term savings on energy losses can be substantial.
- Improved Performance: They enable higher current densities, essential for advanced applications.
Despite their advantages, these technologies often face limitations in terms of cost and material availability, presenting challenges for widespread adoption.
"Technological innovations in energy transmission are not merely enhancements; they represent a foundational shift in how we understand and manage energy use."
Environmental Impact of Energy Transmission
The environmental impact of energy transmission is a crucial aspect that deserves thorough exploration. Energy transmission systems have vast implications for ecosystems, air quality, and overall environmental sustainability. Understanding these impacts is essential, not only for developing efficient energy systems but also for ensuring that we prioritize ecological health.
Energy transmission processes can lead to significant carbon emissions, pollution, and habitat disruption. As energy demand continues to rise, addressing these issues becomes even more pressing. The transition to more sustainable energy solutions requires careful consideration of how energy is transmitted and the broader implications of these choices.
Carbon Footprint Analysis
Carbon footprint analysis involves assessing the net emissions of carbon dioxide and other greenhouse gases throughout the energy transmission lifecycle. This includes extraction, generation, transmission, and distribution phases. Each step contributes differently to the overall carbon footprint, making it critical to analyze every part of the process.
- Source of Energy: The type of energy produced greatly affects the carbon footprint. For example, fossil fuels such as coal and natural gas have high emissions compared to renewable energy sources like solar or wind.
- Efficiency of Transmission: Energy losses during transmission can increase the carbon footprint. Modern technologies aim to optimize efficiency, thus reducing emissions. Systems like high-voltage transmission lines minimize losses over distance.
- Infrastructure: The construction and maintenance of transmission infrastructures, such as power lines and substations, require substantial resources. The energy footprint of these facilities is an important consideration when evaluating overall emissions.
Encouragingly, many countries are implementing carbon accounting practices to better understand the impact of energy transmission. This facilitates informed policy-making and technological advancements, helping shift towards greener alternatives.
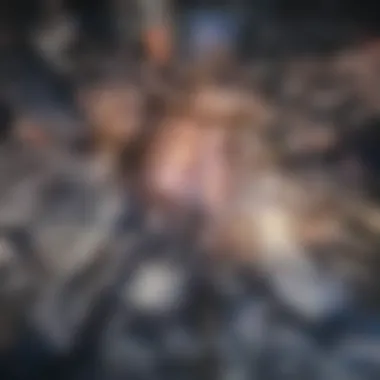
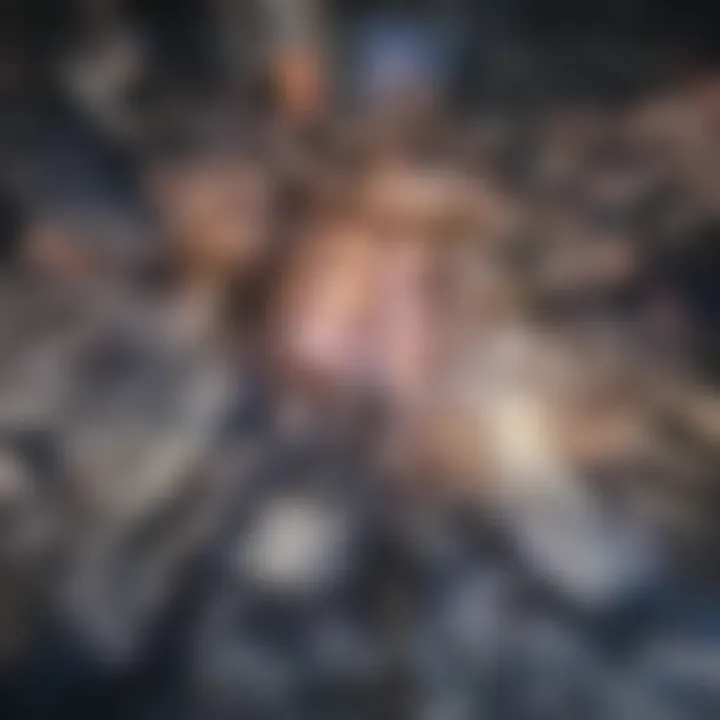
Sustainability Practices
Sustainability practices aim to reduce the negative impacts of energy transmission while promoting environmental health. Implementing these practices can lead to lower resource depletion and minimized ecological disturbances. Key strategies include:
- Integration of Renewable Energy Sources: Utilizing wind, solar, and hydroelectric energy for transmission leads to a marked reduction in carbon emissions.
- Smart Grid Technologies: Employing smart technologies enhances efficiency and reduces wastage. These systems allow for real-time monitoring and management of energy flows, providing opportunities to reduce the carbon footprint.
- Routine Assessments: Regular environmental assessments can identify areas for improvement within existing transmission systems. By continuously monitoring ecological impacts, companies can better mitigate their environmental footprint.
- Community Engagement: Collaborating with local communities on energy projects can foster shared values and goals. This can help reduce local resistance and incorporate environmental safeguards in energy plans.
"The path to sustainable energy transmission requires a balance between technology, community involvement, and ecological awareness."
As we move forward in managing energy transmission's environmental impacts, integrating sustainability practices across all levels becomes imperative. This not only benefits local habitats but contributes significantly to global efforts against climate change.
Future Trends in Energy Transmission
Understanding the future trends in energy transmission is crucial for several reasons. It identifies current technological developments and anticipates challenges that may arise. By analyzing these trends, we can better quantify innovations and apply them to enhance energy efficiency. More importantly, it serves as a compass for those in policy-making, research, and industry sectors. The continuous evolution of energy systems reflects the pressing need for sustainability and resilience in our daily lives.
Emerging Research Areas
Research is increasingly focusing on innovative solutions to improve energy transmission. Some promising areas include:
- Energy Harvesting Technologies: This involves capturing waste energy from various systems. Whether from vibrations or heat, energy harvesting can contribute to a more sustainable energy landscape. Advances in piezoelectric materials and thermoelectric generators are noteworthy.
- Superconducting Materials: High-temperature superconductors are being studied for their potential to minimize energy losses in electrical transmission. The reduction of resistance could lead to significant advancements in efficiency.
- Grid-Scale Energy Storage: With the growing reliance on renewable energy sources, effective energy storage becomes vital. Research into innovative battery technologies, such as solid-state batteries or flow batteries, remains a hot topic.
Additionally, the integration of artificial intelligence and machine learning with energy systems augments predictive capabilities. This can improve grid management and optimize energy distribution.
Global Energy Transition
The global energy transition marks a shift from fossil-based systems to renewable energy sources. This change is not merely environmental but involves economic and social dimensions. The implications are vast:
- Decarbonization Goals: Many countries have committed to reducing carbon emissions. Transitioning to renewable energy sources like solar, wind, and hydro is significant. This is essential to meet international climate agreements.
- Regulatory Changes: Emerging policies encourage investments in clean technology. Governments and institutions are revising standards to facilitate a faster transition, which also presents opportunities for research and development.
- Public Awareness and Participation: Educating communities about renewable energy benefits fosters greater acceptance. Increased public engagement can stimulate more extensive support for local and national projects.
"The shift to renewable energy will require cohesive action from governments, industries, and consumers alike to create a sustainable future."
In summation, the investigation into future trends in energy transmission is not just an exercise in intellectual curiosity. It provides vital perspectives and pathways leading to a more resilient energy future. This underscores the necessity of continued vigilance in research and adaptability in policy frameworks.
With thorough exploration into these topics, professionals can make informed decisions that drive innovation and efficiency in energy systems.
The Role of Government in Energy Transmission
The government plays a crucial role in energy transmission. This involvement is not just administrative, but also fundamental in shaping the policies that influence how energy is generated, transmitted, and utilized. Effective governance ensures that energy systems are not only reliable but also efficient and sustainable. As energy demands continue to rise, the government's role becomes more pertinent in steering the developments within this field.
Governments must create a conducive environment for the advancement of energy transmission technologies. This is done through the establishment of policy frameworks that guide both public and private investment in the energy sector. Such policies not only encourage innovation but also ensure long-term planning aligns with national energy goals. Proper frameworks foster collaboration between government, industry players, and research institutions. This collaboration is essential for overcoming challenges such as integrating renewable energy sources into existing grids or enhancing the efficiency of transmission lines.
"Regardless of the type of energy, the good functioning of transmission systems relies heavily on government policies and regulations."
Additionally, governments have the responsibility to monitor and enforce regulatory standards. These standards ensure that energy transmission is conducted safely and reliably. Regulatory bodies can mandate testing procedures and safety measures to protect both consumers and the environment. For instance, they can set limits on emissions from energy transmission systems or require routine inspections to ensure compliance with safety protocols. Strict adherence to these regulations leads to improved public trust and fosters a better functioning energy system.
In summary, the role of government in energy transmission is multifaceted. It involves not only establishing frameworks and enforcing regulations but also actively engaging all stakeholders in the energy ecosystem. The importance of this engagement cannot be understated, given the complexities of modern energy systems and the pressing need for innovation and sustainability.
Policy Frameworks
Effective policy frameworks are foundational to advancing energy transmission initiatives. These frameworks dictate how resources are allocated, guide research priorities, and set the direction for technological development. For example, clear incentives for renewable energy adoption can stimulate significant investment and innovation in transmission technologies that support clean energy sources.
Key elements of successful policy frameworks include:
- Clear objectives: Goals must be well-defined and measurable to assess progress.
- Funding mechanisms: Strategies should be in place to ensure adequate financial support for energy projects.
- Incentives for innovation: Policies should encourage research and developments in energy transmission technologies.
These components work collectively to ensure a coherent approach, minimizing overlaps and conflicts that can arise in energy policy.
Regulatory Standards
Regulatory standards are essential for establishing the operational norms within the energy transmission sector. These regulations provide benchmarks for safety, reliability, and efficiency. When regulatory standards are implemented effectively, they protect both the public and the environment from potential hazards associated with energy transmission.
Several critical aspects of regulatory standards include:
- Safety regulations: Mandates to ensure all infrastructures meet safety guidelines.
- Efficiency standards: Requirements for the energy losses during transmission to be minimized.
- Environmental impact assessments: Evaluation of potential environmental effects before new energy transmission projects are approved.
By adhering to these regulations, energy transmission systems can operate within defined safety and performance margins, fostering stability and user confidence. This regulatory oversight assures stakeholders that energy services maintain high standards essential for overall system integrity.
Maintaining these standards is important as they adapt to evolving technologies and public expectations. The ongoing dialogue between stakeholders will be key as energy transmission continues to evolve in response to new challenges and opportunities in the energy landscape.
Epilogue
The conclusion section of this article serves a pivotal role in synthesizing the insights gathered throughout the discourse on energy transmission. It encapsulates key points, highlighting the importance of understanding the mechanisms and applications of energy transfer across different forms, such as electrical, thermal, and mechanical. In both theoretical and practical contexts, recognizing these aspects equips readers with the knowledge needed to engage with the complexities of energy systems.
Summary of Findings
In summarizing the findings from the previous sections, it becomes evident that energy transmission is not merely a technical concept but a fundamental component of modern society. The interplay of conduction, convection, and radiation as mechanisms of energy transfer establishes a framework through which efficiency and performance can be evaluated. Not only does the material composition of conductive pathways impact transmission efficacy, but environmental factors, such as temperature and pressure, also play critical roles. Furthermore, we observe that enhancements in technological innovations, like smart grid technologies and high-efficiency conductors, are driving the evolution of energy systems.
"Understanding the nuances of energy transmission mechanisms is crucial for developing sustainable and efficient technology in the future."
- It is vital for students, researchers, educators, and professionals to be aware of the latest advancements in energy transmission.
- The analysis of energy grids, heating systems, and mechanical systems provided context on their applications in real-world scenarios.
- Thus, the findings underscore that efficient energy transmission is not just beneficial, but essential for scientific advancement and practical implementations across various disciplines.
Implications for Future Research
The implications for future research in energy transmission are considerable. As global demand for energy increases, researchers will need to explore materials and methods that can enhance transmission efficiency while minimizing environmental impacts. Topics such as nanomaterials for energy conduction, advanced thermal insulation techniques, and innovative wireless energy transfer systems are ripe for exploration. Furthermore, understanding the role of government policy and regulatory frameworks in shaping energy transmission can lead to more cohesive strategies in energy management.
As energy transmission systems continue to develop, interdisciplinary collaborations will likely yield new insights and technologies. Future research should focus on:
- Sustainability Practices: Investigating how energy transmission can be made more eco-friendly.
- Emerging Technologies: Exploring the integration of artificial intelligence in optimizing energy networks.
- Global Energy Transition: Understanding how policy shifts can facilitate a smoother transition to renewable energy technologies.
Being attuned to these implications will enable a proactive approach to innovations in energy transmission. This focus will help not only in solving current challenges but also in anticipating future needs as our energy landscape continues to evolve.