Transgenic Models: Innovations in Research

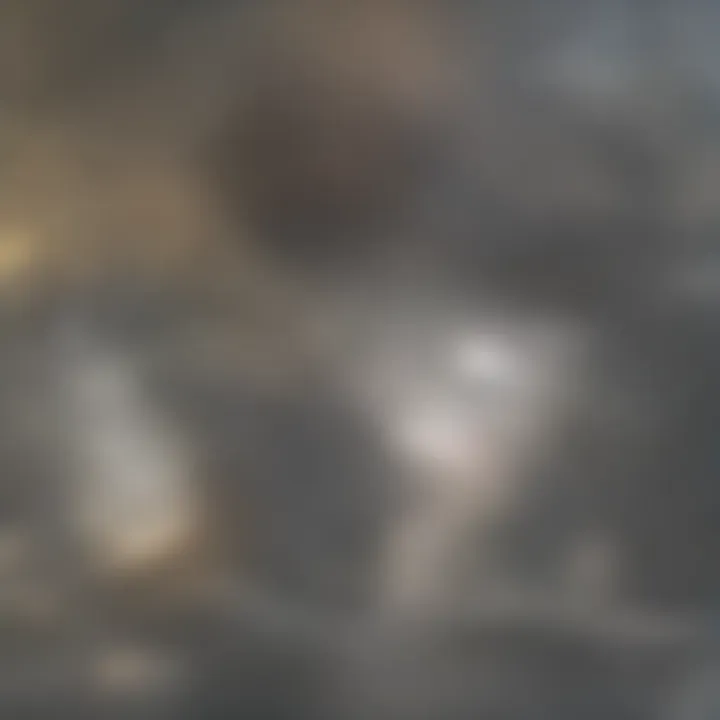
Intro
Transgenic models have revolutionized the landscape of scientific research across various domains. With the capacity to modify genes of organisms, these models serve as a pivotal tool in understanding biological processes and advancing therapeutic strategies. They are instrumental in fields like medicine and agriculture, underscoring the potentials of genetic engineering. This overview will detail the methodologies involved in developing these models, their applications, and the ethical considerations that accompany their use.
Methodologies
Description of Research Techniques
Creating transgenic organisms involves a sequence of intricate steps. The foremost technique is the gene transfer, where desired genes are inserted into the genome of an organism. Common methods include microinjection, electroporation, and viral vectors. Microinjection entails injecting DNA directly into the nucleus of a fertilized egg or an embryo. This method allows for a high degree of control but can be technically challenging. Electroporation uses electrical pulses to increase the permeability of the cell membrane, facilitating DNA uptake. Lastly, viral vectors utilize modified viruses to deliver genetic material into host cells, presenting a more efficient alternative.
Tools and Technologies Used
Several advanced tools and technologies are pivotal in this process. CRISPR-Cas9 is perhaps the most influential technology, enabling precise editing of the genome. This method allows researchers to cut DNA at specific locations and introduce desired genetic changes with high accuracy. Other technologies include TALENs and Zinc Finger Nucleases, which also serve in gene editing but have distinct applications and efficiencies. The integration of these tools supports the creation of models that are more representative of human diseases and other complex biological systems.
Discussion
Comparison with Previous Research
Transgenic models have evolved significantly compared to earlier genetic engineering attempts. The speed and efficiency of modern methods like CRISPR-Cas9 stand in stark contrast to traditional gene-targeting techniques. Earlier models, often reliant on slower methods, failed to provide the resolution and specificity that contemporary researchers can now achieve. This evolution reflects a broader trend towards more sophisticated and ethically sound scientific practices.
Theoretical Implications
The use of transgenic models raises important theoretical implications regarding our understanding of genetics and its role in disease manifestation. By establishing models that mirror human conditions, researchers can delve into underlying mechanisms and uncover new therapeutic avenues. Furthermore, the ethical dimensions of creating and utilizing these models warrant ongoing debate among scientists. The balance between innovation in research and responsible experimentation remains critical. Understanding these dynamics will enhance the research community's approach to future scientific challenges.
"Transgenic models are more than just tools; they are windows into the complexities of biological systems, offering insights that are vital for scientific progress."
Prologue to Transgenic Models
Transgenic models hold a pivotal role in modern biological research. These models allow scientists to manipulate and study the genetic constitution of organisms. Understanding these models is essential for grasping advances in genetics, medicine, and agriculture. This section aims to clarify their importance, highlighting key benefits and considerations.
Definition and Overview
Transgenic models are organisms that have had foreign DNA introduced into their genome. This can be done through various techniques, allowing the expression of new genes. The primary purpose is to study specific functions of genes and their interactions. In biomedical research, transgenic models help simulate human diseases, enabling researchers to test new therapies effectively.
Transgenic animals, plants, and microorganisms are used widely.
Some benefits include:
- Innovative Disease Models: Transgenic mice, for example, can replicate human diseases. This allows for in-depth studies of pathology and treatment options.
- Enhanced Agricultural Practices: Plants can be genetically modified for better yield, resistance to pests, and environmental adaptability.
- Basic Science Advancements: Understanding gene function through transgenic organisms fosters essential discoveries across biological fields.
However, these models also raise questions about genetic manipulation's ethical, environmental, and safety aspects.
Historical Background
The inception of transgenic models traces back to the late 20th century. Early efforts focused on basic genetic manipulation techniques. The first successful transgenic mouse was produced in 1981, which marked a significant timeline in genetics. This development ignited a wave of research, leading to the refinement of various genetic engineering methods. Over the years, the advances made in technology, such as CRISPR, have facilitated broader applications of transgenic models. As scientists overcome earlier limitations, these models have become integral to genetic research, disease modeling, and agricultural innovation.
This evolution emphasizes the importance of transgenic models in understanding complex biological systems and enhancing therapeutic strategies.
"Transgenic models represent a leap forward in our ability to mimic and study dynamic biological processes that would be challenging to analyze using traditional methods."
Genetic Engineering Techniques
Genetic engineering is a crucial component in the development of transgenic models. These techniques allow scientists to manipulate genes to create organisms with desired traits or functions. The implications of this technology are vast, spanning various fields including medicine, agriculture, and environmental science. In this section, we will explore specific genetic engineering techniques that enable the creation of transgenic models, along with their significance and potential applications.
CRISPR/Cas9 Technology
CRISPR/Cas9 represents a breakthrough in genetic engineering. This technique utilizes a guide RNA to target specific DNA sequences, allowing precise modifications to the genome. CRISPR/Cas9's simplicity and efficiency have made it a preferred choice for many researchers. Unlike older methods of genetic modification, such as homologous recombination, CRISPR allows for multiple genes to be edited simultaneously. This capability is particularly valuable in creating models for complex diseases where multiple genes may be involved.
Benefits of CRISPR/Cas9 include:
- Precision: It enables targeted editing without unintended mutations.
- Speed: The process takes significantly less time compared to traditional techniques.
- Cost-Effectiveness: It reduces the overall costs associated with genetic manipulation.
However, there are considerations regarding off-target effects, where unintended parts of the genome may be altered. This raises concerns, especially in clinical applications. Researchers are actively working on enhancing the specificity of CRISPR technology to mitigate these risks.
Transposon-Mediated Methods
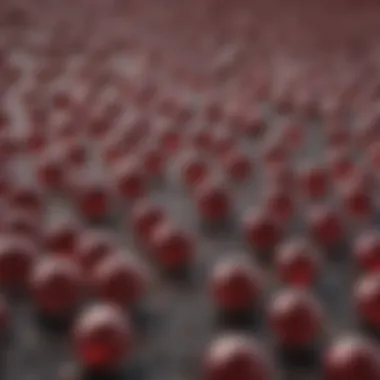
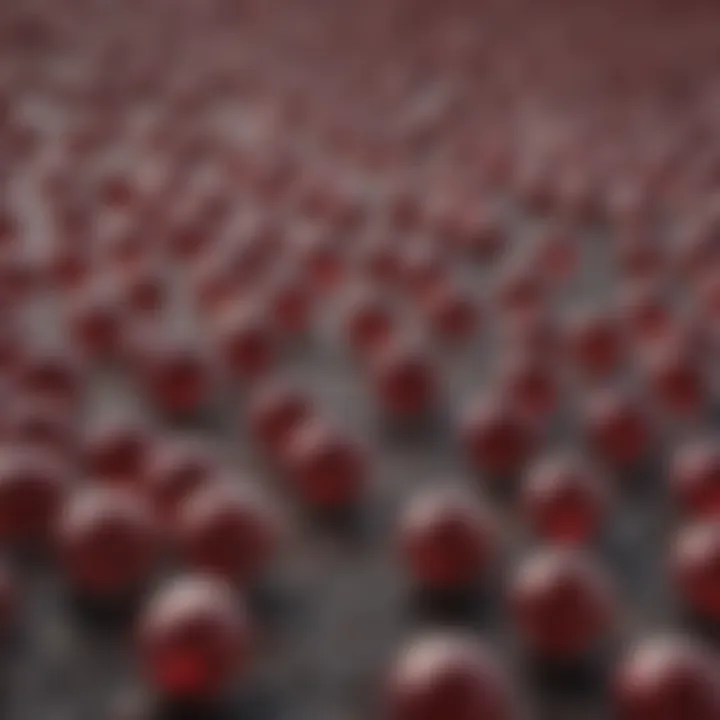
Transposons, also known as "jumping genes," can be used for genetic modification by inserting DNA into a genome at random sites. These methods harness the natural ability of transposons to move within the genome. They allow for the integration of new genes without the need for precise targeting.
Some key aspects of transposon-mediated methods include:
- Flexibility: They can accommodate larger DNA sequences, which is beneficial for inserting whole gene cassettes.
- Random Integration: This can lead to unexpected but potentially useful traits in the modified organisms.
Despite their advantages, random integration can also lead to gene disruptions, which needs to be carefully monitored. These methods have shown promise in plant genetic engineering and vector development.
Viral Vector Systems
Viral vectors represent another powerful tool in genetic engineering. They are modified viruses that can deliver genetic material into host cells effectively. Different types of viral vectors exist, such as lentiviruses, adenoviruses, and adeno-associated viruses, each with unique properties and applications.
Key features of viral vector systems include:
- Efficiency: They can infect a wide range of cell types, making them suitable for diverse applications.
- Stable Integration: Some viral vectors integrate efficiently into the host's genome, ensuring persistent expression of the transgene.
Considerations when using viral vectors:
- Immunogenicity: Some vectors may trigger immune responses, which can complicate their use in therapeutic settings.
- Safety: A thorough understanding of viral biology is required to minimize risks involved in their use.
Types of Transgenic Models
Understanding the various types of transgenic models is essential for comprehending their applications and significance in scientific research. These models serve as invaluable tools for elucidating complex biological processes and testing hypotheses in real-world systems. Each type offers unique benefits and considerations relevant to specific research inquiries.
Animal Models
Animal models have been central to biological research for decades. They provide insights into human diseases, enabling scientists to study pathology in a controlled environment. Commonly used animals include mice, rats, and zebrafish. Mice, for instance, are particularly favored due to their genetic similarity to humans, rapid reproduction cycles, and established genetics.
Transgenic mice are created by incorporating foreign DNA into their genome, allowing researchers to analyze disease mechanisms and evaluate therapeutic interventions. For example, mouse models for Alzheimer's disease have significantly advanced our understanding of neurodegenerative processes and drug efficacy.
However, ethical considerations about animal welfare arise. It is vital to balance scientific advancement with humane treatment. Protocols and regulations vary, emphasizing the importance of responsible conduct in research involving animals.
Plant Models
Plant models play a crucial role in agricultural research and biotechnology. They offer insights into genetic traits that contribute to vital aspects such as yield, disease resistance, and stress responses. Notable examples include Arabidopsis thaliana, a model organism frequently used to study plant biology and genetics. Transgenic plants are developed to express specific traits by inserting genes associated with desirable characteristics.
For instance, genetically modified crops like Bt cotton are engineered to produce toxins against pests, reducing the need for chemical pesticides. This leads to more sustainable farming practices. However, public concerns about food safety, environmental impact, and the economic implications of genetically modified organisms cannot be overlooked. It is crucial to address these concerns through transparency and scientific validation.
Microbial Models
Microbial models, including bacteria such as Escherichia coli and yeast like Saccharomyces cerevisiae, are essential for molecular biology and genetic studies. These organisms are often employed to understand gene function, protein expression, and metabolic pathways. They reproduce quickly and can be modified easily using various genetic engineering techniques.
For example, E. coli is commonly used to produce recombinant proteins, which have numerous applications in medicine, such as insulin production. The manipulation of microbial genomes also allows for the exploration of antibiotic resistance and pathogenicity mechanisms, thus contributing significantly to medical microbiology.
Addressing the ethical dimensions of microbial research is also important. There is a need for responsible research practices that mitigate risks associated with pathogenic strains or genetically modified microbes.
"Transgenic models enable the dissection of complex biological questions and the innovation of viable solutions in both medicine and agriculture."
Applications in Biomedical Research
The field of biomedical research presents numerous challenges, particularly when it comes to understanding complex human diseases. Transgenic models serve as a fundamental tool in this arena. They allow scientists to create specific genetic modifications that can replicate human disease states in a controlled environment. This capability is crucial for several reasons.
Specific Elements of Applications in Biomedical Research
- Disease Modeling: These models help researchers to mimic the pathology of diseases. By introducing specific genes associated with a disease, scientists can observe the progression and understand the biological pathways involved. This directly translates to identifying potential therapeutic targets.
- Drug Development: Transgenic models play a critical role in the drug development process. Researchers can use these models to test the efficacy and safety of new drugs before moving to clinical trials. This step often involves evaluating drug responses in the context of genetically relevant environments.
- Gene Therapy Studies: The use of transgenic models facilitates research in gene therapy. Scientists can assess the delivery and integration of therapeutic genes into target cells. These studies provide insights into the optimization of gene therapy protocols for treating various genetic disorders.
"Transgenic models bridge the gap between basic research and clinical application, paving the way for significant advancements in medical science."
In summary, the applications of transgenic models in biomedical research are profound. They enhance our understanding of disease mechanisms and contribute to the development of new therapeutic strategies. As research continues to advance, the importance of these models will only grow, underscoring their role as pivotal instruments in the discovery and innovation processes.
Disease Modeling
Disease modeling using transgenic organisms has become a powerful approach in understanding the intricacies of various medical conditions. For instance, models developed to study conditions such as Alzheimer’s disease or certain types of cancer enable researchers to observe complex interactions that take place within living organisms. This real-time observation is often not possible with in vitro studies alone.
By utilizing specific genetic alterations, scientists can evaluate how diseases progress and respond to different treatments. Furthermore, transgenic models provide opportunities to identify novel biomarkers that may pave the way to personalized medicine, allowing for more tailored approaches to patient care.
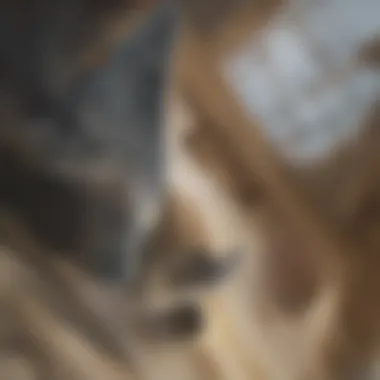
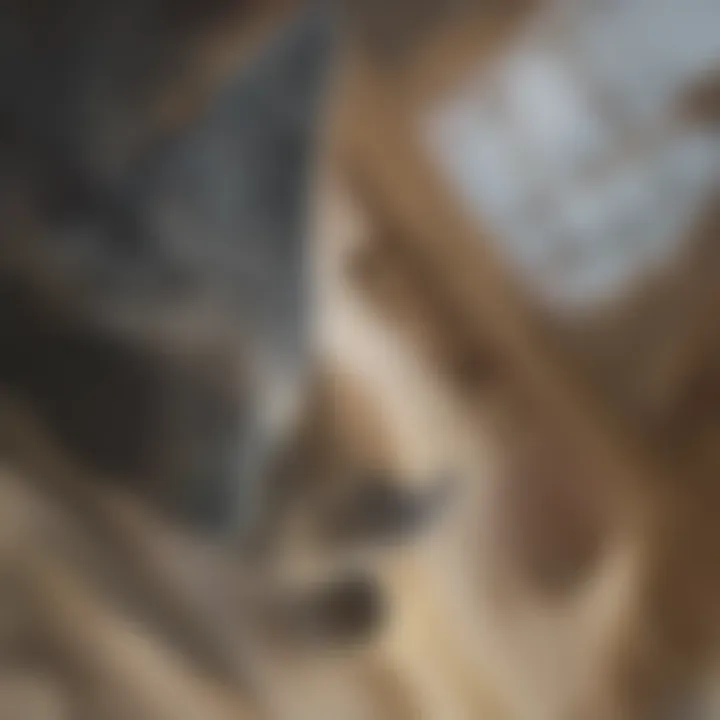
Drug Development
Drug development is a lengthy and costly process, often taking decades to bring a new drug to market. One major benefit of transgenic models is their ability to reduce the time and costs involved in this process. By testing new molecules in these models, researchers can gain insights into pharmacokinetics, toxicity, and optimal dosing strategies.
Moreover, using transgenic models allows researchers to ask more specific questions. For example, the effectiveness of a drug on a particular genetic background can be assessed before proceeding to human trials. This level of specificity enhances the chances of success in clinical phases and minimizes the potential for adverse effects in human populations.
Gene Therapy Studies
Gene therapy is an exciting area of research that seeks to correct genetic disorders at their source. Transgenic models provide critical insights into the delivery mechanisms and expression levels necessary for successful gene therapy. By understanding how transgenes are incorporated and expressed in a living organism, researchers can refine delivery methods and improve therapeutic outcomes.
In addition to developing the technology itself, transgenic models also help in ethical discussions concerning gene editing and therapy. As the field grows, so does the attention on regulatory matters and long-term implications, making comprehensive studies essential.
Agricultural Advancements
Agricultural advancements driven by transgenic models play a crucial role in enhancing food security and addressing global challenges such as climate change and population growth. By utilizing genetic engineering techniques, researchers have developed crops that not only improve yields but also possess traits that allow them to thrive in adverse conditions. Such innovations can lead to a more sustainable agricultural system while reducing the reliance on chemical pesticides and fertilizers.
Genetically Modified Crops
Genetically modified crops, often referred to as GMOs, are plants whose genetic material has been altered through biotechnology. This modification aims to introduce desirable traits that can significantly enhance agricultural productivity. For instance, crops like Bt corn and Roundup Ready soybeans have been designed to resist pests and tolerate specific herbicides, respectively. These traits help farmers reduce crop losses and decrease the workload associated with pest management.
Moreover, genetically modified crops have shown to improve nutritional content. For example, Golden Rice has been engineered to contain higher levels of Vitamin A, which can combat malnutrition in regions where rice is a staple food.
Disease Resistance
Disease resistance in crops has become increasingly important as plant pathogens evolve rapidly. Transgenic models enable scientists to identify and integrate resistance genes from various species into crops. This strategy helps in creating plants that can withstand diseases without the frequent application of fungicides or other chemical treatments.
Using methods such as CRISPR, researchers can specifically target and edit genes in cultivated species, thereby enhancing their resistance to diseases like late blight in potatoes, which had previously led to devastating crop failures. Increasing disease-resistant crops can also minimize economic losses for farmers and ensure a more stable food supply.
Enhanced Yield Traits
Improving yield traits through transgenic models is fundamental for meeting the demands of a growing global population. By enhancing crop characteristics such as drought resistance, nutrient utilization, and photosynthetic efficiency, scientists can develop varieties that produce more food on the same or lesser amount of land.
For instance, scientists are working on genetically modifying rice to optimize its growth under limited water supply, an innovation that directly addresses water scarcity in agricultural regions. Such enhancements not only support productivity but also promote sustainable farming practices.
Understanding these advancements in agricultural biotechnology highlights the significant impact they can have on food production systems. As research in transgenic models continues, the potential for positive agricultural transformation becomes increasingly apparent.
Ethical Considerations
Understanding the ethical considerations surrounding transgenic models is essential in their implementation and development. These considerations not only affect scientific research but also resonate with public perception and acceptance. The integration of advanced genetic engineering techniques to create transgenic organisms raises profound questions about the moral implications of manipulating life forms. Engaging with these issues is crucial in ensuring responsible research that prioritizes both human and environmental well-being.
Animal Welfare Issues
Transgenic models often involve animal subjects, prompting significant welfare concerns. The process of creating these models can lead to suffering due to genetic alterations. Various techniques may introduce unintended consequences, which can compromise the animals' quality of life. As a result, researchers must adhere to strict guidelines that safeguard animal welfare.
- Key Impacts on Animal Welfare:
- Genetic modifications can result in health complications like deformities and chronic illnesses.
- Environmental stressors often influence how these animals adapt.
- The necessity for enrichment in housing is critical for mental well-being.
Institutional Review Boards (IRBs) typically evaluate proposed research projects involving animals. This review process ensures that researchers demonstrate the necessity of using transgenic models and articulate strategies to minimize suffering.
Impact on Biodiversity
Transgenic models also spark debate regarding biodiversity. The introduction of genetically modified organisms into the ecosystem poses risks that can alter existing ecological balances. On one hand, these innovations have the potential to enhance traits like disease resistance, which can contribute to agricultural sustainability. On the other hand, they can lead to unforeseen consequences.
- Concerns Regarding Biodiversity:
- Dominance of genetically modified species over native species can lead to extinction.
- Altered gene pools may reduce genetic variation essential for resilience against diseases.
- Potential for transgenes to spread to wild populations can create ecological havoc.
Ethical consideration is a vital component of responsible scientific practice, ensuring potential benefits do not come at the expense of biodiversity and ecological stability.
Balancing the benefits of transgenic innovation with the preservation of biodiversity remains a complex challenge. Researchers must engage in continuous dialogues with ecologists and policy makers to develop strategies that mitigate risks while harnessing the advantages offered by transgenic models. Through such collaborative efforts, it is possible to identify responsible pathways for advancing scientific knowledge while safeguarding our natural environment.
Regulatory Framework
The regulatory framework surrounding transgenic models is essential for ensuring their safe and ethical application in both scientific research and industry. These guidelines provide a structured approach to the development, testing, and deployment of transgenic organisms. By maintaining a balance between innovation and safety, regulations help foster public trust and integrity in scientific advancements.
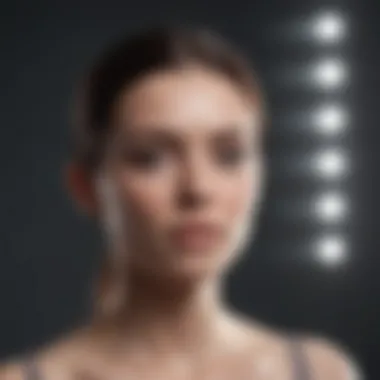
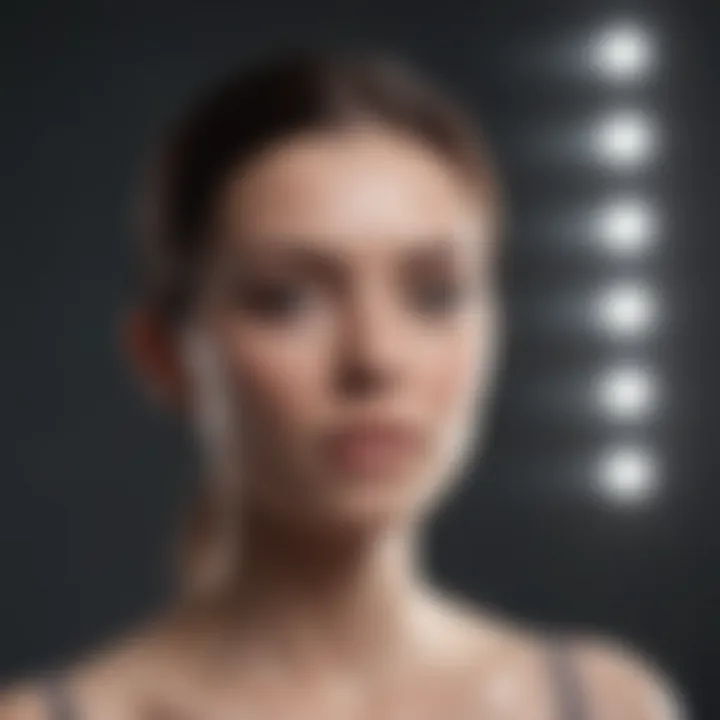
In many cases, regulatory bodies assess the potential risks associated with transgenic organisms, guiding scientists to mitigate unintended consequences related to health, environment, and biodiversity. This scrutiny is crucial given the complex interactions that transgenic organisms may have with ecosystems.
Furthermore, effective regulation helps streamline the approval process for transgenic products. Without clear regulations, the path to market can be fraught with delays and complications, hindering the potential benefits that these innovations can offer. Thus, an established regulatory framework facilitates responsible science while promoting advancements in technology and agriculture.
"A robust regulatory framework is not a mere bureaucratic necessity; it's a safeguard for our society on the brink of biotechnological revolution."
Global Guidelines
Global guidelines, such as those put forth by the World Health Organization and the Food and Agriculture Organization, establish fundamental principles for the safe use of transgenic models. These guidelines offer a baseline for countries seeking to develop their own regulatory systems. They encompass critical areas, such as the assessment of environmental risks, food safety, and ethical considerations.
Some crucial aspects of global guidelines include:
- Risk Assessment: Evaluating potential health and environmental risks associated with transgenic products before approval.
- Transparency: Promoting openness in the development and testing phases, enabling public engagement and trust.
- Sustainability: Encouraging practices that align with sustainable agricultural and ecological principles.
These global benchmarks aim to foster collaboration among nations, ensuring standardization in safety practices while addressing regional needs and concerns.
Local Legislation
Local legislation plays a pivotal role in shaping the regulatory landscape for transgenic models. Each region or country may have unique legal frameworks that take into consideration local environmental contexts, agricultural practices, and public sentiment.
Key elements of local legislation may include:
- Approval Processes: Detailed procedures that govern the submission and evaluation of transgenic organisms for research and commercial use.
- Compliance and Monitoring: Systems to ensure ongoing compliance with regulatory standards after approval. This may include regular inspections and reporting requirements.
- Public Engagement: Mechanisms for local communities to voice concerns or provide input regarding transgenic applications.
The harmonization of local laws with global guidelines is crucial for fostering a research-friendly environment while addressing ethical concerns. This complementarity ensures that scientific innovation occurs within a framework that not only prioritizes safety but respects cultural and regional nuances.
Future Trends in Transgenic Research
The exploration of transgenic models is not static; it evolves as scientific capabilities advance. The future of transgenic research holds remarkable promise. This is essential for the ongoing evolution of methodologies in creating and utilizing transgenic models. As technology improves, so do the potentials of these models in effectively addressing complex biological questions.
In the next few years, the focus will be on making genetic manipulations more precise. This will include advancements in gene editing techniques. The decreasing costs and increased accessibility of these technologies will enable wide-scale applications across various fields. Furthermore, regulatory frameworks are anticipated to adapt to these advancements. These changes will ensure that innovations can progress responsibly while considering ethics and safety.
Advancements in Technology
Technology is at the core of transgenic research. Innovations will likely lead to higher efficiency and effectiveness. For instance, advancements in genome editing technologies such as CRISPR will be pivotal. These tools allow for precise alterations in organisms, greatly expediting research processes.
Moreover, developments in synthetic biology are expected to integrate with transgenic models. This integration will enhance our ability to design organisms with novel traits. Additionally, improvements in delivery systems for genetic material will make the process of developing transgenic models simpler and more reliable. A few key areas showing promise include:
- Nano-delivery systems for targeted gene transfer.
- Progress in optogenetics allowing for real-time manipulation of gene expression.
- Enhanced plant breeding techniques that involve markers linked directly to desirable traits.
Integration of AI and Machine Learning
The integration of artificial intelligence and machine learning into transgenic research is set to revolutionize how data is analyzed and models are developed. Machine learning algorithms can quickly process large datasets. This has significant implications for identifying gene functions and understanding complex interactions in biological systems.
In future research, AI can optimize the design of transgenic models by predicting outcomes based on genetic modifications. This ability to simulate various genetic alterations significantly reduces time and resources required for experimentation. Some promising applications include:
- Use of pattern recognition to identify genetic variations that confer beneficial traits.
- Development of predictive models using historical data to forecast the success of transgenic applications in fields like agriculture and human health.
- Enhanced image analysis for monitoring phenotypes in transgenic organisms, making phenotyping much more efficient.
The synergy between advancing technology and AI will likely lead to unprecedented breakthroughs in transgenic research.
In summary, future trends in transgenic research will focus on technological advancements and the integration of AI. These changes not only promise to enhance our understanding of genetic mechanisms but also foster developments that can benefit medicine, agriculture, and beyond.
Finale
In this article, we delved into the multifaceted domain of transgenic models, uncovering the significant impacts these innovations have on scientific research. The importance of transgenic models lies not only in their capacity to enhance our understanding of genetic functions but also in their practical applications across diverse fields such as medicine, agriculture, and environmental science.
Summary of Potential Impacts
The potential impacts of transgenic models are profound. They serve as effective tools in:
- Disease understanding: Transgenic animal models contribute to our comprehension of genetic diseases, providing insights that can lead to novel therapeutic strategies.
- Agricultural productivity: Genetically modified crops help address food security challenges by increasing yield and resistance to pests and diseases.
- Environmental sustainability: By altering microbial models, researchers can create bio-remediation systems that efficiently clean polluted environments.
These models not only push the boundaries of current scientific knowledge but also provoke essential discussions about their ethical implications and long-term consequences on biodiversity.
The Path Forward
Looking ahead, the path forward for transgenic research appears promising yet requires careful navigation.
- Technological Advancements: Continued innovations in genetic engineering techniques, particularly with the integration of CRISPR technologies, offer improved precision in gene editing. This can lead to breakthroughs in various scientific fields.
- Ethical Frameworks: As research evolves, developing robust ethical guidelines will be crucial to address concerns around animal welfare and ecological impacts.
- Global Collaboration: Enhanced collaboration among international research institutions can accelerate the translation of findings from laboratory settings to practical applications.
By fostering a balanced approach that emphasizes both scientific advancement and ethical responsibility, the future of transgenic models promises to yield significant benefits while minimizing potential risks. In summary, understanding the essence and impact of transgenic models will serve as a cornerstone for future scientific inquiries.