Single Cell DNA Sequencing: Techniques and Applications
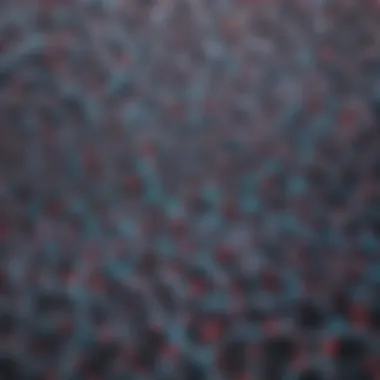
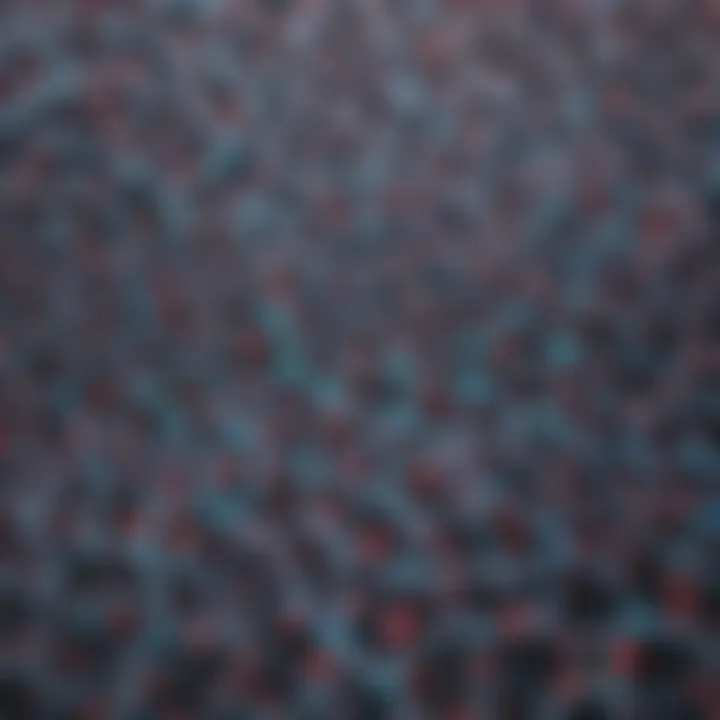
Intro
Single cell DNA sequencing has emerged as a revolutionary tool in the field of genomics. As researchers strive to unravel the complexities of genetic patterns at a granular level, single cell techniques promise breakthroughs previously unattainable. This method allows scientists to examine the genetic material of individual cells, providing insights that bulk sequencing methods cannot offer.
This article aims to dissect the methodologies behind single cell DNA sequencing, outline the tools and technologies that drive this approach, and explore its broad applications, particularly in cancer research, developmental biology, and personalized medicine. The potential benefits and challenges in the field will also be examined to provide a holistic view of the subject.
It is paramount to understand that the ability to investigate DNA at the single-cell level can lead to advancements in various scientific disciplines and medicine. It forces us to reconsider traditional views of genetic variability. Let us now move to the first critical component: Methodologies.
Foreword to Single Cell DNA Sequencing
Single cell DNA sequencing represents a significant advancement in genomic research. It allows for the dissection of genetic information from individual cells, revealing insights into cellular heterogeneity that standard bulk sequencing can overlook. The significance of this method lies not just in data acquisition but also in the interpretation of how diverse cellular environments influence disease mechanisms, developmental processes, and therapeutic responses.
The ability to analyze DNA sequences at the single-cell level has transformed our understanding of both normal and pathological states. By studying the genetic profiles of individual cells, researchers can explore the complexities of tumor evolution, cellular differentiation, and the personalized aspects of medicine. This technology is critical for advancing our grasp of heterogeneity in cancers, which is vital for devising more effective treatments.
Moreover, understanding single cell DNA sequencing involves recognizing its implications across various fields of study, including statistics, molecular biology, and bioinformatics. As the techniques evolve, challenges such as data interpretation and processing will also unfold, adding layers of complexity to this already intricate field. The integration of single cell sequencing within broader biological frameworks can potentially herald greatly improved outcomes in research and clinical settings, aligning genetic information with observable phenomena and diseases.
Definition and Significance
Single cell DNA sequencing is the process of analyzing the genetic material of individual cells. This approach contrasts with more traditional methods that analyze a bulk collection of cells, where the intricate differences among individual cells are lost. The significance of this technology cannot be overstated, as it opens a window into the genetic variation present within seemingly homogeneous populations. Understanding these variations is crucial for elucidating mechanisms underlying cancer progression, embryonic development, and numerous other biological processes.
The potential for single cell DNA sequencing extends into personalized medicine. By connecting genetic profiles to specific cellular behaviors, it becomes feasible to tailor therapies that suit the unique genetic landscape of a patient’s disease.
Historical Background
The journey of single cell DNA sequencing began in the late 20th century when researchers first envisioned the possibility of analyzing individual cells at a molecular level. The development of laser capture microdissection and microfluidics paved the way for isolating single cells efficiently. Initial efforts focused primarily on RNA sequencing, but advancements in technology have allowed for the amplification of DNA from single cells.
Over the past two decades, next-generation sequencing technologies have rapidly progressed, making single cell DNA sequencing more accessible and cost-effective. Essential contributions from this period include protocols for whole genome amplification that have enabled the comprehensive genetic analysis of single cells.
The progression of knowledge and technology in this field underscores the dynamic nature of genomics. As researchers continue to explore various applications of single cell DNA sequencing, it is becoming ever more clear how pivotal this technique will be in shaping the future of biological research and medicine.
Core Techniques in Single Cell DNA Sequencing
The exploration of core techniques in single cell DNA sequencing is essential for understanding this advancing field of genomics. Each method contributes distinct advantages, ultimately leading to a more precise analysis of genomic information at the cellular level. This section delves into three primary methodologies: microfluidics and droplet-based methods, whole genome amplification, and next-generation sequencing technologies. Understanding these techniques is vital for researchers, students, and professionals who aim to harness the power of single-cell sequencing in their work.
Microfluidics and Droplet-Based Methods
Microfluidics refers to the manipulation of tiny amounts of fluid, allowing for high-throughput analysis and experimentation with single cells. This technique enables researchers to isolate individual cells from a heterogeneous mixture, facilitating the examination of their unique genetic make-up.
Droplet-based methods utilize microfluidic technology to encapsulate single cells into droplets. This results in the formation of individual compartments where DNA can be extracted and amplified. The advantages of this approach include:
- High Throughput: Thousands of cells can be processed simultaneously.
- Cost-Effectiveness: Lesser reagents and materials are needed per sample.
- Reduced Contamination: Each droplet serves as a controlled environment.
However, this method also comes with challenges such as the requirement for specialized equipment and potential biases in droplet formation. These aspects must be carefully managed to ensure reliable data acquisition.
Whole Genome Amplification
Whole genome amplification (WGA) is a crucial technique in single cell DNA sequencing. It allows for the replication of the entire genome from minimal DNA quantities, which is often the case when working with single cells. By amplifying the genetic material, researchers can make comprehensive analyses without needing extensive amounts of genomic DNA. The primary methods for WGA include:
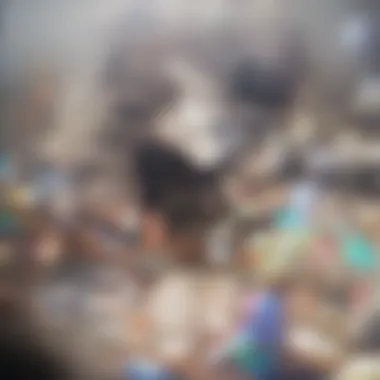
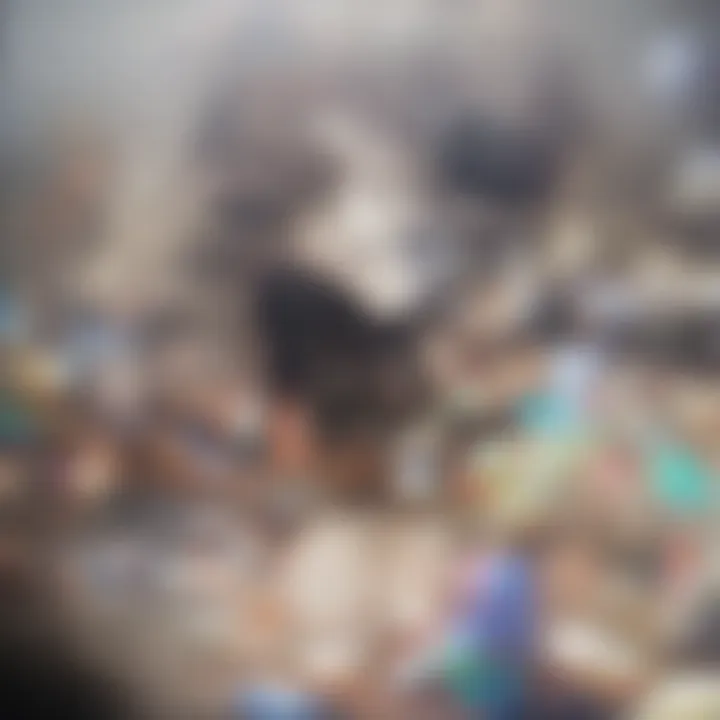
- Multiple Displacement Amplification (MDA): An enzymatic process that expands the DNA strand using specialized polymerases.
- Recombinant PCR: A method that uses a PCR process tailored for low quantities of DNA.
Despite its importance, WGA can introduce biases and errors in the amplification process. Therefore, validating WGA results is a critical step in ensuring accuracy in downstream applications.
Next-Generation Sequencing Technologies
Next-generation sequencing (NGS) technologies have revolutionized the field of genomics, enabling rapid and cost-effective sequencing of DNA. In single cell DNA sequencing, NGS provides the depth and resolution needed to unveil the intricacies of cellular genomic content. Key advantages of NGS include its:
- Massive Parallelization: Multiple sequences can be processed at once, vastly increasing throughput.
- High Resolution: Capable of detecting variations within genomic sequences, such as single nucleotide polymorphisms (SNPs).
- Scalability: Adaptable to different project needs, whether large-scale population studies or focused investigations.
NGS methods such as Illumina sequencing and Ion Torrent are widely employed in single cell workflows. However, these technologies also present challenges like handling the complexity of the data generated. Analyzing such vast datasets requires sophisticated computational techniques.
"The integration of advanced sequencing technologies in single cell analysis is driving a paradigm shift in our understanding of genomics."
Applications of Single Cell DNA Sequencing
Single cell DNA sequencing holds transformative potential for various fields, primarily due to its ability to analyze genetic material at the level of individual cells. This precision grants insights that collective analysis overlooks. Such capabilities enable scientists and clinicians to understand complex biological systems with unprecedented clarity. This section delves into the specific applications in cancer genomics, developmental biology, and personalized medicine, highlighting their significance and implications.
Cancer Genomics
Tumor Heterogeneity
Tumor heterogeneity refers to the presence of diverse cellular populations within a single tumor. This diversity can lead to varying responses to treatment, which complicates prognosis and therapy. Understanding this heterogeneity is vital to the development of effective cancer therapies.
Using single cell DNA sequencing enables researchers to dissect the genetic variations among tumor cells. It allows for the identification of distinct subpopulations within a tumor, contributing to a more nuanced understanding of cancer progression. The key characteristic of tumor heterogeneity is the dynamic nature of tumor cells, which can evolve in response to treatment, thereby promoting resistance.
Tumor heterogeneity is a popular focus in this article because it exemplifies the challenges that modern oncology faces. Additionally, the unique feature of heterogeneity is that it sheds light on why some treatments fail. This knowledge can significantly inform the development of targeted therapies. However, the complexity of tumor cell populations also poses challenges in standardizing approaches and interpreting data, which requires a sophisticated understanding of genomic methodologies.
Clonal Evolution
Clonal evolution describes the process by which cancer cells evolve over time, leading to the emergence of dominant clones. Each clone may carry distinct genetic alterations that influence its behavior, including its ability to metastasize or develop resistance to therapy. Thus, clonal evolution is critical in understanding how tumors adapt and survive.
A key characteristic of clonal evolution is its iterative nature, as genetic variants accumulate over time, creating a diverse pool of cells. This makes clonal evolution an essential choice for this article because it directly relates to treatment challenges and outcomes in oncology. The unique feature of this concept lies in its explanation of treatment failure and disease recurrence. By studying clonal evolution through single cell DNA sequencing, researchers can identify genetic markers that indicate resistance or aggressiveness of cancers.
However, studying clonal evolution also has its disadvantages, primarily related to the complexity of tracking genetic changes over time. It requires robust methodologies and comprehensive data analysis tools, which can be resource-intensive and demanding.
Developmental Biology
Cell Fate Determination
Cell fate determination refers to the process by which a cell becomes specialized into a particular type. This process is fundamental in developmental biology as it contributes to tissue formation and regeneration. By applying single cell DNA sequencing, researchers can unravel the mechanisms that guide cells in making fate decisions.
One notable characteristic of cell fate determination is its multi-faceted influence on development. The choice to specialize involves a variety of signals, genetic interactions, and environmental factors. This aspect makes the topic relevant for this article because it aids in understanding complex developmental processes. The unique feature lies in the capacity to monitor and analyze gene expression variations among individual cells, highlighting the subtle influences on cell fate.
The advantages of utilizing single cell approaches for studying cell fate determination are evident in the resolution of data obtained. However, technical limitations can arise, particularly in isolating individual cells without compromising integrity.
Embryonic Development Analysis
Embryonic development analysis is crucial for comprehending how organisms grow and differentiate from a single fertilized cell to a structured multi-cellular entity. This aspect of developmental biology benefits significantly from single cell DNA sequencing as it allows for detailed insights into the early stages of development.
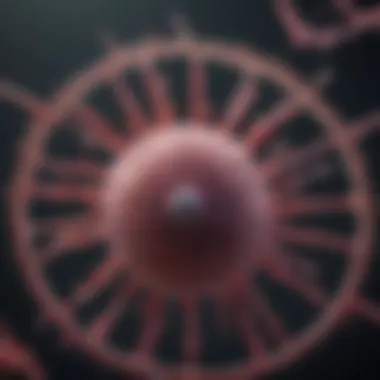
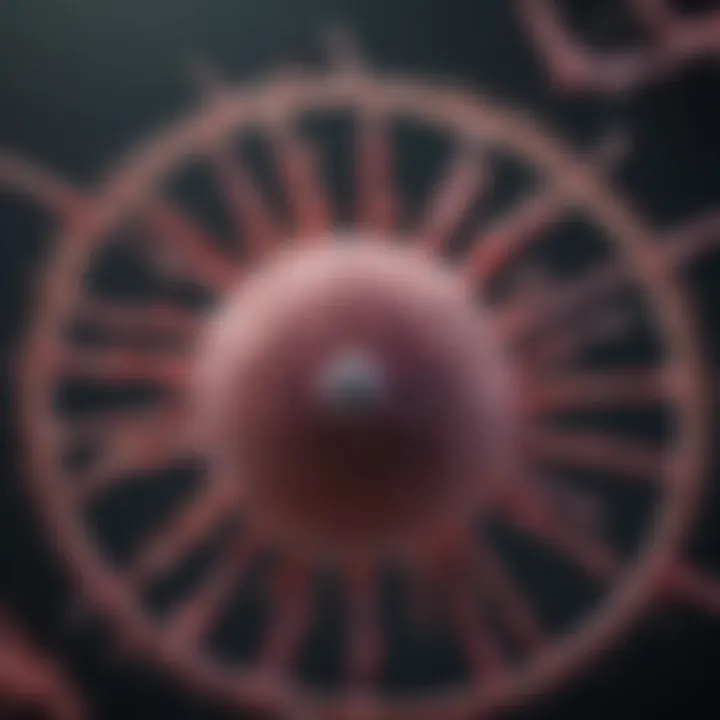
A key characteristic of embryonic development analysis is the representation of numerous developmental pathways occurring simultaneously. This complexity makes it a beneficial topic for discussion in this article, as understanding these pathways can inform not only basic science but also applied research in reproductive health. The unique aspect here is the potential to identify critical stages and markers that direct development.
However, challenges in studying embryonic development can stem from the ethical considerations surrounding embryonic research. Public sensitivity and regulatory constraints may limit the scope of studies, impacting the overall field.
Personalized Medicine
Targeted Therapies
Targeted therapies are designed to directly target specific molecular pathways involved in disease. In the context of cancer treatment, these therapies have emerged as a means to enhance efficacy while minimizing damage to healthy tissues. Single cell DNA sequencing plays a vital role by allowing for the accurate identification of genetic mutations that can drive treatment decisions.
The importance of targeted therapies in this article lies in their potential to revolutionize patient treatment plans. By understanding the genetic profile of a patient's tumor, clinicians can customize therapies to address the specific characteristics of that tumor. The unique feature here is the ability to monitor the response of individual patients to treatments over time, providing insights into effectiveness and resistance.
However, challenges remain in scaling these therapies for broader populations, where genetic variability can introduce complexity. Ensuring equitable access and understanding the implications of targeted approaches continue to be pressing issues.
Genomic Profiling
Genomic profiling refers to the comprehensive analysis of an individual's genetic makeup. This analysis provides crucial information that can guide decisions in personalized medicine, particularly in how treatments are tailored to patients' unique genetic backgrounds. Single cell DNA sequencing enhances genomic profiling by revealing variations at an unprecedented resolution.
The key characteristic of genomic profiling is its ability to illuminate not only static genetic information but the dynamic alterations occurring within cells in response to therapies or environmental factors. This focus is a beneficial choice for this article because it broadens the understanding of how genomics can influence medical treatments. The unique aspect lies in the depth of insight that single cell analysis provides, allowing for a more accurate prediction of treatment responses.
Nevertheless, genomic profiling also carries disadvantages including the need for extensive data management and interpretation. The complexity of data analysis can overwhelm traditional workflows, necessitating advanced computational resources and skilled personnel.
Challenges in Single Cell DNA Sequencing
Single cell DNA sequencing presents a groundbreaking advancement in the field of genomics. However, it also brings with it a series of challenges that need to be addressed to fully exploit its potential. Understanding these challenges is crucial for researchers and practitioners aiming to employ these techniques in their work. This section discusses important aspects, benefits of identifying these limitations, and considerations that arise from the challenges faced in single cell DNA sequencing.
Technical Limitations
One of the most significant technical limitations in single cell DNA sequencing is sample preparation. The process of isolating individual cells maintains the integrity of genetic material but introduces a risk of contamination and loss of cellular information. Inadequate handling may result in incomplete data, which can mislead research conclusions. Moreover, current amplification methods, such as whole genome amplification, can introduce biases in the representation of genetic material, affecting downstream analysis.
Another aspect is the sequencing depth and coverage that is often insufficient for heterogenous samples. The variability among individual cells means that some genetic variants may not be detected if they are present at low frequencies. This poses a significant risk in applications such as cancer genomics, where the detection of minor clonal populations is crucial for understanding tumor evolution.
In addition, technical challenges in the data acquisition phase, including errors during sequencing, can produce noise that complicates interpretation. Illumina technology, while advanced, still encounters issues of accuracy that limit the fidelity of the results one can derive from single cell sequencing data.
Data Analysis and Interpretation
Once sequencing is completed, the next hurdle involves data analysis and interpretation. Single cell DNA sequencing generates vast amounts of data, necessitating sophisticated bioinformatics tools for their analysis. Current software tools may struggle to manage data and derive meaningful biological insights from it. Hence, there is a need for enhanced algorithms that can integrate multi-dimensional datasets more effectively.
Additionally, interpreting the implications of the data remains complex. Variability among single cells can lead to different interpretations of the same genetic variant. Thus, researchers must account for this heterogeneity in their analyses. Advanced statistical methods are needed to make meaningful comparisons across samples, which can often be resource-intensive and time-consuming.
Moreover, there is a growing concern over reproducibility. Given the variability in single cell studies, it requires rigorous validation for the findings to be accepted within the scientific community. It is essential for researchers to standardize protocols and share methodologies openly.
"The ability to analyze genomic data at the single-cell level is revolutionary. Yet, without addressing these challenges, the full value of this innovation may be limited."
Ethical Considerations in Single Cell DNA Sequencing
In the realm of single cell DNA sequencing, ethical considerations are paramount. This innovative technology has the potential to yield significant insights into individual cellular characteristics and contribute to advancements in various scientific fields. However, these capabilities also raise critical questions regarding privacy and the implications for genetic editing. Addressing these concerns is essential to ensure responsible and equitable use of single cell DNA sequencing.
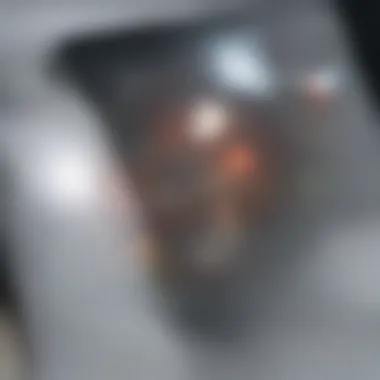
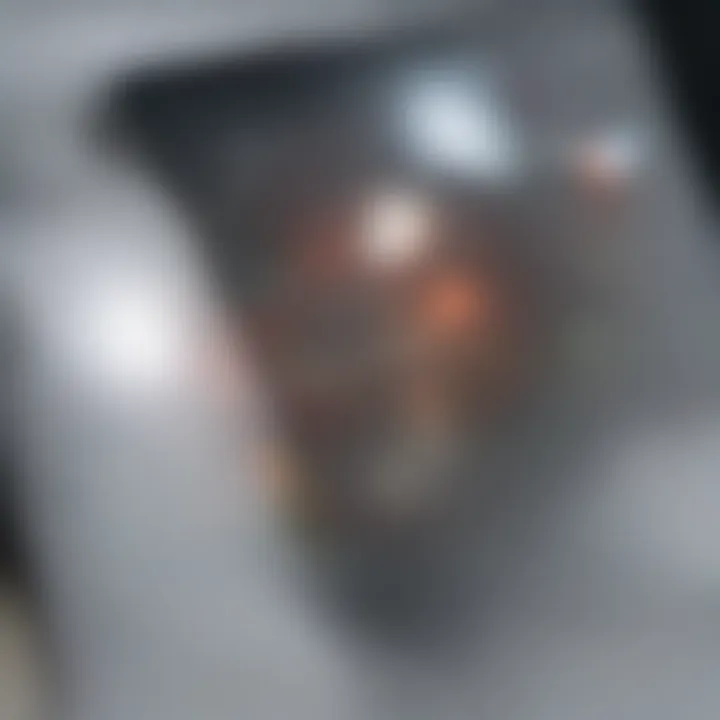
Privacy Concerns
Privacy remains a vital issue within the single cell DNA sequencing landscape. As researchers analyze genetic data at the single-cell level, the potential for misuse or unauthorized access to sensitive information increases. The individual nature of this data raises questions not only about what should be shared but also with whom.
- Personal Identifiability: Genetic data obtained from single cell sequencing can be used to trace familial relationships and predispositions to certain diseases. This information, if not handled appropriately, could lead to discrimination in areas such as employment and insurance.
- Anonymization Practices: It's critical to implement robust anonymization and data protection protocols to safeguard personal identities. Researchers must prioritize these practices to minimize risks associated with data breaches.
- Regulatory Framework: Developing a regulatory framework is essential for guiding how genetic data should be collected, stored, and shared. These guidelines must respect individuals' rights while enabling scientific progress.
"Ethical considerations must guide the development and application of genomic technologies to protect individual rights and promote societal trust."
Implications for Genetic Editing
Single cell DNA sequencing intersects closely with genetic editing technologies, amplifying the ethical discourse surrounding these fields. The capability to edit genes based on sequencing data can lead to remarkable advancements in medicine and biology. However, it also presents complex moral dilemmas that must be addressed.
- Editing Considerations: The ability to edit genetic material raises questions about designer babies and the potential societal ramifications of genetic modifications.
- Unintended Consequences: Genetic editing can have unforeseen effects on both the individual and broader ecological systems. Understanding these implications through single cell sequencing could illuminate mechanisms of gene function but demands rigorous ethical scrutiny.
- Informed Consent: The ethical principle of informed consent becomes particularly important when integrating genetic editing with single cell sequencing results. Participants must be clearly informed about what their data will be used for, ensuring they can make educated choices about their participation.
Future Directions in Single Cell DNA Sequencing
The field of single cell DNA sequencing is rapidly evolving, with new techniques and applications emerging continually. Understanding future directions in this domain is essential for those involved in genomics research. As challenges are identified, innovative solutions are proposed, leading to improved methodologies and expanded usage.
Key areas to focus on include the integration of single cell sequencing with cutting-edge technologies and interdisciplinary approaches. These directions promise to enhance our comprehension of complex biological systems.
Emerging Technologies
Emerging technologies play a vital role in shaping the future of single cell DNA sequencing. Several innovations are worth noting:
- CRISPR-based Techniques: The development of CRISPR technology can enable more precise DNA editing. Researchers can target specific genes within single cells, leading to insights about gene function and regulation. This precision can further enhance genomic profiling.
- Nanopore Sequencing: This technique allows for real-time sequencing of DNA strands as they pass through a nanopore. It is highly promising due to its ability to produce long reads of genetic material from single cells. Such capacity can aid in reconstructing complex genomes and understanding structural variations in cancer cells.
- Integrated Microfluidic Systems: Advances in microfluidics technology facilitate high-throughput analysis of single cells. These systems can streamline workflows and reduce costs. The integration with sequencing technologies makes it easier to capture and process data efficiently.
These technologies collectively contribute to a comprehensive understanding of cellular behavior at an unprecedented resolution, which can catalyze breakthroughs in various biological disciplines.
Integration with Other Omics Approaches
Single cell DNA sequencing does not exist in isolation. Integration with other omics approaches can strengthen findings and provide a holistic view of cells and their environments. Combining transcriptomics, proteomics, and metabolomics enables:
- Enhanced Insights into Cellular Function: By integrating genomic information with transcriptomic and proteomic data, researchers can develop a clearer picture of how genes influence cell function and behavior.
- Identification of Biomarkers: Integration may reveal biomarkers that signify disease states or cellular responses. This could be particularly important in areas like cancer diagnosis and treatment, where early detection is crucial.
- Understanding Cellular Interactions: Considering how single cells communicate and interact with their surroundings can unearth valuable insights. By integrating omics data, researchers can improve our understanding of tissue heterogeneity and microenvironments.
In summary, the future of single cell DNA sequencing is tied closely with technological advancements and interdisciplinary collaborations. As these areas develop, they will undoubtedly enhance our understanding of various biological processes, leading to significant advancements in research and applications.
As we look to the future, the synergy between emerging technologies and integrated approaches will likely define the trajectory of single cell DNA sequencing, unveiling discoveries that may currently seem unattainable.
Epilogue
The conclusion of this article serves an essential role in summarizing the vast and complex landscape of single cell DNA sequencing. By reviewing the significant findings and discussions throughout the sections, it highlights how single cell DNA sequencing is transforming the fields of genomics and molecular biology. The implications of this technique are numerous, creating opportunities for groundbreaking discoveries that go beyond traditional methods.
Summary of Key Points
The article covered several key elements regarding single cell DNA sequencing:
- Definition and Significance: Established the foundational understanding of what single cell DNA sequencing is and its importance in genomics.
- Core Techniques: Discussed the methodologies involved, including microfluidics, whole genome amplification, and advanced sequencing technologies.
- Applications: Explored various fields such as cancer genomics, developmental biology, and personalized medicine, revealing how these areas benefit from enhanced genomic insights.
- Challenges: Addressed the technical limitations and data analysis issues that researchers face in the implementation of these sophisticated techniques.
- Ethical Considerations: Evaluated the privacy and ethical implications of sequencing at the single-cell level, emphasizing the need for careful consideration.
- Future Directions: Looked at emerging technologies and the integration with other omics approaches, shedding light on the anticipated advancements in this area.
By synthesizing these points, we can appreciate the full scope of single cell DNA sequencing and its profound impact on genetic research.
The Future of Genomic Research
The future of genomic research is closely tied to advancements in single cell DNA sequencing techniques. As more sophisticated technologies become integrated into everyday research practices, the potential for new discoveries will only increase. The push towards personalized medicine will drive further investments in this area, leading to more precise treatments tailored to individuals based on their unique genetic information. In addition, the integration of single cell sequencing with other omics approaches will provide a more holistic view of biological systems, offering clearer insights into complex diseases and development processes.
In summary, single cell DNA sequencing represents a pivotal advancement in genomics. As we continue to explore its capabilities and address its challenges, the foundation for new discoveries expands. This field holds potential not only for academic research but also for clinical applications that may redefine standards of care in medicine.