In-Depth SEM EDS Analysis: Techniques & Applications
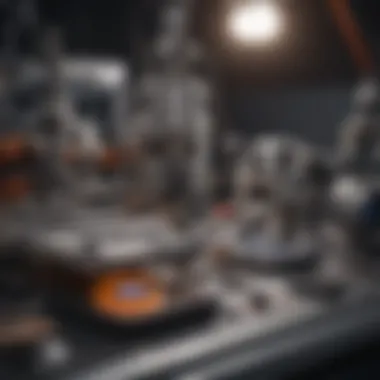
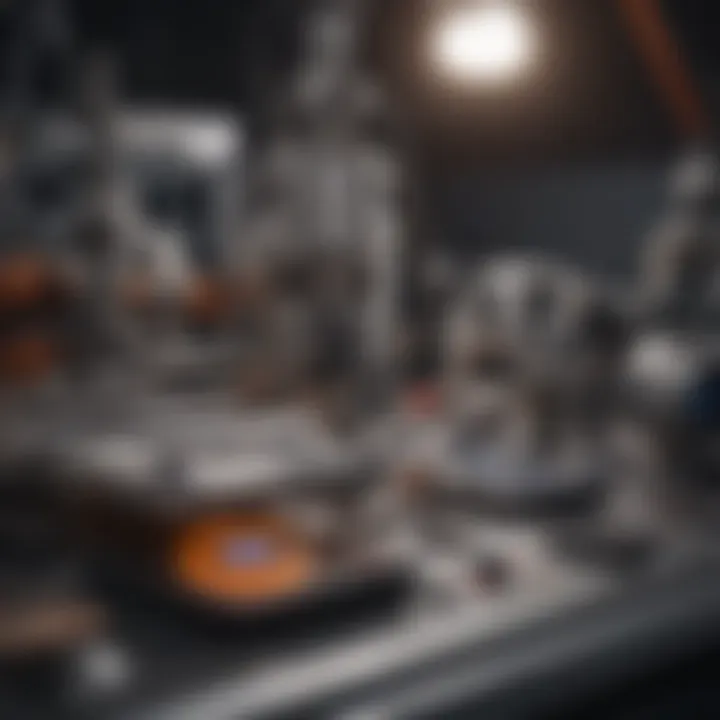
Intro
In the modern landscape of scientific investigation, the ability to analyze materials at a granular level is critical. Scanning Electron Microscopy Energy Dispersive Spectroscopy (SEM EDS) stands out as a premier technique that combines imaging and elemental analysis. This method provides valuable insights across various fields, including materials science, biology, and environmental studies. Throughout this article, we will dissect the methodologies, tools, and applications of SEM EDS analysis, emphasizing its significance in advancing our understanding of complex materials.
Methodologies
Description of Research Techniques
SEM EDS is a sophisticated technique that merges two complementary processes: scanning electron microscopy and energy-dispersive X-ray spectroscopy. Scanning electron microscopy creates high-resolution images of sample surfaces by focusing a concentrated beam of electrons. This technique offers exceptional depth of field, rendering intricate details that are often missed with traditional optical microscopy.
On the other hand, energy-dispersive X-ray spectroscopy identifies elemental composition. When the electron beam interacts with the sample, it displaces inner shell electrons, producing characteristic X-rays specific to each element. This allows researchers to gather quantitative and qualitative data about the elemental makeup of materials.
Several aspects of SEM EDS enhance its versatility:
- Spatial Resolution: Capable of resolving features at nanometer scales.
- Elemental Mapping: Provides maps showing the distribution of elements in a sample.
- Minimal Sample Preparation: Often requires limited preprocessing, preserving the sample's original state.
Tools and Technologies Used
The performance of SEM EDS relies on a combination of hardware and software technologies. Key components include:
- Electron Gun: Generates a focused beam of electrons.
- Sample Stage: Holds the specimen in place, often with the capability of tilt and rotation to achieve various angles.
- Detector Systems: Capture both secondary electrons for imaging and X-ray emissions for elemental analysis.
- Software Packages: Essential for data processing and visualization. Programs like ImageJ and commercial software from manufacturers such as FEI or JEOL facilitate detailed analysis of results.
"SEM EDS enables researchers to reveal the hidden structure of materials, providing insights that shape our understanding of both fundamental science and applied technologies."
Discussion
Comparison with Previous Research
When comparing SEM EDS with previous methods of elemental analysis, its advantages become evident. Traditional techniques like X-ray fluorescence or bulk chemical analysis often lack the spatial resolution that SEM EDS possesses. As such, SEM EDS has gained recognition in advanced research, particularly for its ability to conduct intricate studies across many disciplines.
Recent studies highlight its applications in contaminated site analysis, failure analysis in manufacturing, and novel material development. The continuous advancements in SEM EDS technology also reflect a significant shift towards more precise and detailed investigations.
Theoretical Implications
The implications of SEM EDS extend beyond mere analysis. Understanding the interactions between high-energy electrons and matter aids in refining theoretical models in material science. The ability to visualize and quantify elemental distributions lends itself to deeper investigations into the relationships between material structure and properties, enhancing theoretical frameworks in several scientific domains.
In summary, SEM EDS serves as a pivotal tool in contemporary scientific inquiry. Through detailed methodologies and robust data acquisition techniques, it fosters significant advancements in research, bridging the gap between theoretical insights and practical applications.
Prologue to SEM EDS Analysis
Scanning Electron Microscopy combined with Energy Dispersive Spectroscopy plays a pivotal role in modern scientific research. This method provides intricate details about material composition and structure at a microscopic level. Understanding SEM EDS analysis helps researchers and professionals in various fields, such as materials science and biology, to analyze samples effectively.
Definition and Scope
SEM EDS is an analytical technique that facilitates material characterization. Scanning Electron Microscopy captures high-resolution images of a sample's surface, while Energy Dispersive Spectroscopy identifies elemental compositions. Together, they form a powerful toolkit for assessing physical structures and chemical properties of materials.
The scope of SEM EDS extends beyond simple imaging. It allows for quantitative analysis, where the concentration of elements can be measured. This capability is vital for various applications, including quality control in manufacturing and research in advanced material development. By offering precise elemental maps, SEM EDS aids in understanding the relationship between material structure and function.
Historical Background
The development of SEM EDS is rooted in the evolution of microscopy techniques. Scanning Electron Microscopy began gaining momentum in the 1960s as researchers sought to overcome the limitations of traditional light microscopy. The ability to visualize samples at significantly higher magnifications transformed the field of microscopy.
Energy Dispersive Spectroscopy emerged as an essential complementary technique. Initially integrated with Transmission Electron Microscopy, EDS became widely adopted in scanning electron microscopes. Its capacity to analyze the composition of materials quickly and accurately further solidified its importance in scientific research. As technological advancements shaped both SEM and EDS, researchers were enabled to explore more complex samples across diverse applications.
Fundamental Principles of SEM
Understanding the fundamental principles of Scanning Electron Microscopy (SEM) is crucial for anyone engaged in scientific research or material analysis. SEM provides high-resolution images and detailed information about the surface topography and composition of samples. The focus on these principles allows researchers to effectively harness the capabilities of SEM technologies for diverse applications.
Basic Mechanisms of Electron Microscopy
Electron microscopy operates on the principles of electron-beam interaction with a sample. In this context, electrons are emitted from a source, usually a tungsten filament or field emission gun, and focused into a beam. This beam scans across the sample, which results in various interactions with the specimen's atoms. The most important interactions are:
- Secondary Electrons: These are ejected from the sample surface due to the electron beam, allowing for the generation of surface topographical information.
- Backscattered Electrons: These electrons retain more energy compared to secondary electrons, providing insight into the sample's composition based on atomic number contrast.
- X-rays: Produced during the electron-solid interactions, they are crucial for elemental analysis via Energy Dispersive Spectroscopy (EDS).
Each type of electron contributes to a richer understanding of the sample, enabling detailed imaging and analysis that is central to SEM's applications.
Components of a Scanning Electron Microscope
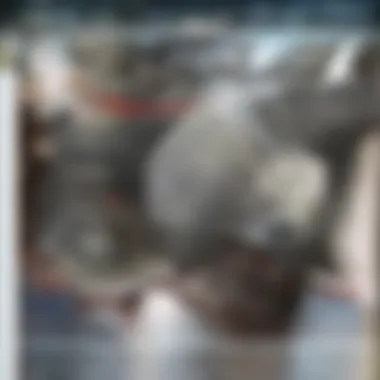
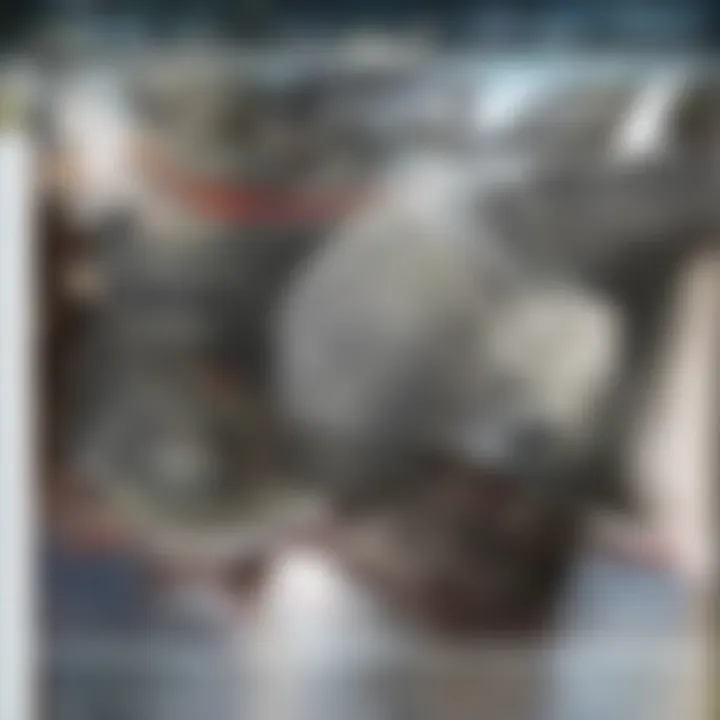
A Scanning Electron Microscope consists of several critical components, each serving specific functions to facilitate high-resolution imaging. Understanding these components helps in troubleshooting and optimizing operation. Notable components include:
- Electron Source: Provides electrons for imaging. Common sources include tungsten filaments and field emission guns.
- Electromagnetic Lenses: Focus and control the electron beam. They adjust the beam diameter, enabling high-resolution detail in imaging.
- Sample Chamber: Houses the specimen being analyzed, often in a vacuum to prevent scattering of electrons due to air molecules.
- Detectors: Collect signals generated from the interaction between the electron beam and the sample. Typical detectors include secondary and backscattered electron detectors, which contribute to surface imaging, and EDS detectors for composition analysis.
- Control and Imaging Software: Used to manipulate the SEM settings and collect data, ensuring accurate and efficient analysis.
In sum, the collaboration of these components empowers different SEM functionalities, making it a versatile analytical tool crucial across material science, biology, and more.
Introductory Principles of EDS
Energy Dispersive Spectroscopy (EDS) plays a pivotal role in the context of Scanning Electron Microscopy (SEM). This section elucidates how EDS complements SEM, enhancing its applications and effectiveness in various scientific analyses. Understanding EDS principles not only elevates the role of SEM but also solidifies its application across many fields, such as materials science, biology, and environmental studies.
Energy Dispersive Spectroscopy Overview
Energy Dispersive Spectroscopy is a technique used alongside SEM to provide elemental analysis of samples. It operates on the principle of detecting X-rays emitted from a sample when it is bombarded by a focused electron beam. When the high-energy electrons interact with the atoms in the specimen, they displace inner-shell electrons, causing an excited atom to release energy in the form of X-rays as electrons transition from higher energy levels to fill the vacancies.
The key advantages of EDS include the ability to determine the elemental composition of materials quickly and effectively. The method allows researchers to identify elements in a sample with a minimum detection limit often in the range of parts per thousand, depending on the element and the matrix. Additionally, the simultaneous acquisition of spectral data means that an entire area can be analyzed in a single scan, saving time and improving productivity.
Key points:
- EDS provides qualitative and quantitative elemental analysis.
- Real-time data acquisition aids in immediate feedback for researchers.
- Integration with SEM offers high spatial resolution for accurate mapping of elemental distributions.
Interaction of X-rays and Matter
The interaction between X-rays and matter is essential for EDS to function. When X-rays generated by the sample interact with other matter, various phenomena occur. Photoelectric effect, Compton scattering, and Rayleigh scattering are the primary interactions that influence how data is captured in EDS.
- Photoelectric Effect: This occurs when an X-ray photon knocks out an inner-shell electron from an atom, leading to the emission of characteristic X-rays for that element.
- Compton Scattering: This happens when X-rays collide with electrons, resulting in a change in energy and direction of the X-ray. This interaction can dilute the spectral data but provides essential information on elemental behavior.
- Rayleigh Scattering: Unlike Compton scattering, Rayleigh scattering preserves the energy of the X-ray while changing its direction. It does not contribute directly to the EDS spectrum but is important for understanding the sample's morphology.
The efficiency of X-ray detection depends on various factors, including the thickness of the sample, the geometry of the setup, and the energy resolution of the detection system. Therefore, understanding these interactions helps in optimizing SEM EDS results, leading to more precise elemental characterizations.
"Incorporating EDS into SEM analysis yields a comprehensive understanding of materials, promoting deeper insights in diverse scientific endeavors."
In summary, the introductory principles of EDS lay the groundwork for understanding how elemental analysis is carried out in SEM. The combination of these technologies not only enhances the analytical capabilities of researchers but also opens up new avenues for exploration in scientific research.
Workflow of SEM EDS Analysis
The workflow of SEM EDS analysis is a critical foundation for understanding how this technique operates effectively in various applications. It involves several stages that guide researchers from initial sample preparation to final data interpretation. Recognizing the importance of each step within this workflow aids in achieving accurate results and mitigating potential errors.
Sample Preparation Techniques
Sample preparation is vital in SEM EDS analysis because it ensures high-quality imaging and accurate chemical characterization. The objective here is to create a surface that is both clean and representative of the sample under investigation. Effective sample preparation often includes the following procedures:
- Sectioning or Cutting: This is essential for bulk materials, allowing access to internal features.
- Cleaning: Use solvents or ultrasonic baths to remove contaminants from the surface. This is crucial as any residue can obscure results.
- Coating: Non-conductive samples often require a thin conductive layer, such as gold or carbon. This minimizes charging effects which can distort images.
- Mounting: Proper fixation of samples on stubs ensures stability during imaging and analysis.
By implementing these techniques, researchers achieve a sample that provides reliable data, facilitating accurate EDS spectra analysis.
Operating Procedures in SEM EDS
Operating procedures in SEM EDS analysis encompass the step-by-step protocol carried out during imaging and data collection. These procedures include:
- Calibration: Regular calibration of the SEM and EDS systems is essential for ensuring the accuracy of measurements. This should ideally include energy calibration and spatial resolution tests.
- Setting Parameters: You must select appropriate voltage and current settings, as these influence the interaction volume and resolution of the images.
- Imaging Setup: Choose magnification levels and adjust the working distance to optimize focus and depth of field. Adjusting these parameters can enhance the visibility of features of interest.
- Data Collection: Capture images and EDS spectra simultaneously. This integration allows for the correlation of morphology with elemental composition.
Proper execution of these operating procedures is paramount for delivering precise analysis results. Any oversight can lead to erroneous conclusions, thereby underlining the importance of training and rigor in this phase.
Data Acquisition and Acquisition Times
The part of SEM EDS that deals with data acquisition significantly impacts the depth of analysis possible. Understanding acquisition times is crucial for balancing resolution and data quality. Several factors influence acquisition:
- Detector Efficiency: A highly efficient detector can shorten acquisition times while maintaining spectral integrity.
- Sample Characteristics: Different materials exhibit varying signal intensities, thus influencing the required time for accurate measurement.
- Desired Quality: The trade-off between speed and precision often dictates how long you should record data.
- Analysis Strategy: Employing techniques like mapping or line scans involves different acquisition times and strategies.
In practice, an adequate acquisition time is essential for generating reproducible and reliable elemental data. Researchers often must calibrate their approaches based on experimental requirements and sample conditions.
Understanding each component of the SEM EDS workflow not only facilitates effective operation but also enhances the reliability of analytical results, which is vital for scientific inquiry.
Data Interpretation and Analysis
Data interpretation and analysis is a critical aspect of Scanning Electron Microscopy Energy Dispersive Spectroscopy (SEM EDS). This section focuses on the significance of understanding and analyzing the data collected from SEM EDS to extract meaningful information about the material under investigation. The process involves meticulous examination of the generated spectra and the subsequent categorization into qualitative and quantitative insights.
Reading EDS Spectra
Reading EDS spectra is essential to comprehend the elemental composition and distribution in a sample. The EDS spectrum plots counts per channel against energy levels. Each peak in the spectrum corresponds to a specific element, with its position indicative of the elemental energy levels. Understanding how to accurately identify these peaks requires a thorough grasp of energy calibration and background correction methods.
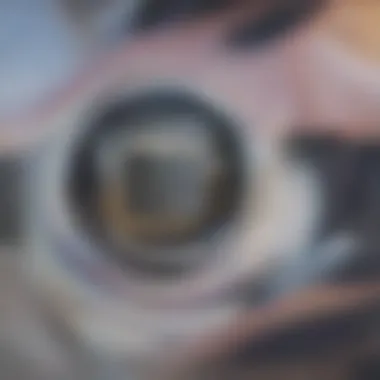
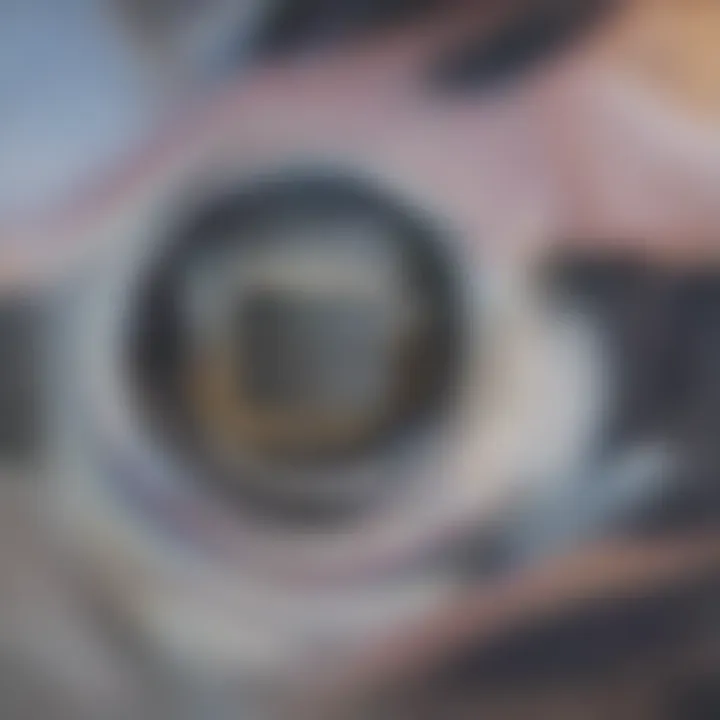
In practice, one must pay attention to the peak heights and areas. Higher peaks generally signify larger elemental concentrations, while smaller peaks indicate trace elements. It is crucial to differentiate between overlapping peaks, which is often a challenge. Expertise in reading these spectral nuances can significantly enhance the analysis outcomes.
"Accurate reading and interpretation of EDS spectra are vital for reliable elemental analysis in diverse applications."
Quantitative vs. Qualitative Analysis
The distinction between quantitative and qualitative analysis in SEM EDS cannot be overstated. Qualitative analysis focuses on identifying which elements are present in the sample, primarily through peak detection in the EDS spectrum. This analysis serves as a preliminary step to understand the composition of materials.
Quantitative analysis, on the other hand, aims to determine the exact concentration of each element. This involves using specific software and calibration standards to obtain reliable results. The proper techniques employed in quantitative analysis can yield extensive data on the elemental proportions and their distributions.
There are several considerations for both analysis types:
- For qualitative analysis, accuracy depends on resolving power and detector efficiency.
- Quantitative analysis needs proper calibration standards for accurate results.
- The selection of sample preparation methods can influence both analysis types.
Advantages of SEM EDS Analysis
The advantages of Scanning Electron Microscopy Energy Dispersive Spectroscopy (SEM EDS) analysis are significant, making it a valuable tool across many scientific domains. Understanding these advantages is crucial for students, researchers, educators, and professionals who aim to enhance their analytical capabilities. SEM EDS combines the strengths of imaging and elemental analysis, enabling a comprehensive investigation of samples.
One of the most compelling aspects of SEM EDS is its ability to provide high-resolution images of samples. This capability allows for detailed examinations at the nanoscale, which is essential for characterizing materials with intricate structures. High-resolution imaging can reveal critical features that are invisible to other techniques, offering insights into the morphology and topography of the material under investigation.
High Spatial Resolution
High spatial resolution is a defining feature of SEM EDS analysis. This quality is attributed to the focused electron beam used in SEM, which interacts with the sample at a very fine scale. Typically, resolutions reach below 10 nanometers, which is a remarkable feat for imaging techniques. Such precision enables researchers to observe not only the surface structure of materials but also their fine details, leading to a better understanding of their properties and behaviors.
The importance of high spatial resolution cannot be understated in fields such as materials science and nanotechnology. In materials science, for example, being able to analyze grain boundaries and defect structures at a nanoscale offers insight into how materials behave under stress or in various environments. Furthermore, this precision plays a significant role in quality control processes across industries, such as electronics and metal fabrication.
Elemental Characterization Capabilities
The elemental characterization capabilities of SEM EDS stand as another significant advantage of this analytical technique. SEM EDS allows for the determination of the elemental composition of materials with a high degree of accuracy. By analyzing the X-rays emitted from a sample when it is bombarded with electrons, researchers can identify and quantify the elements present.
This elemental analysis is particularly beneficial for several reasons:
- Identification of Unknown Materials: When confronted with an unknown material, SEM EDS can quickly provide crucial information about its composition, aiding in material identification and selection.
- Assessment of Material Homogeneity: The technique enables an assessment of the uniformity of elemental distribution within a sample, which is essential in applications such as alloys or composite materials.
- Trace Element Detection: SEM EDS can detect trace elements, which can often be critical in understanding material properties and behaviors.
The combination of high-resolution imaging and precise elemental analysis makes SEM EDS a powerful tool for advancing research in various scientific fields.
In summary, the advantages of SEM EDS analysis are profound, primarily characterized by high spatial resolution and elemental characterization capabilities. As scientific exploration grows and material complexity increases, the role of SEM EDS in analytical routines becomes ever more vital.
Applications of SEM EDS Analysis
The applications of SEM EDS analysis are vast and varied, reflecting its significance in multiple scientific fields. This technique empowers researchers to delve into the microscopic world, offering critical insights into material properties, biological structures, and environmental phenomena. By analyzing samples at a microscopic scale, SEM EDS analysis enables precise elemental identification and characterization, which enhances both qualitative and quantitative analysis. As a result, it plays an important role in advancing scientific knowledge.
Materials Science
In materials science, SEM EDS analysis is invaluable for examining metals, ceramics, and polymers. Materials scientists leverage this technique to understand composition and phase distribution, which are crucial for creating advanced materials. It helps in determining the microstructure of materials and assessing their mechanical and thermal properties. Through EDS, scientists can identify the presence and concentration of elements within a sample, assisting in quality control and developing new composite materials.
"The ability to visualize and measure the composition of materials at the micro-level fosters innovation in developing stronger and lighter materials."
Additionally, SEM EDS analysis contributes significantly to failure analysis. By investigating the elemental composition of fractured materials, researchers can trace back the cause of failure and suggest improvements. This capability is essential for industries such as aerospace, automotive, and consumer electronics, where material integrity is paramount.
Biological Applications
In biology, SEM EDS finds numerous applications ranging from cellular studies to the analysis of tissues and biomaterials. By providing detailed images of biological specimens, this technique allows researchers to explore the morphology of cells and tissues. EDS complements this by adding the ability to assess elemental composition, which is crucial for understanding biological processes at a molecular level.
Researchers utilize SEM EDS analysis to study the interactions between biological specimens and materials, such as implants or tissue scaffolds, to ensure compatibility and safety. This can lead to improved medical devices and treatment strategies.
Moreover, this analysis contributes significantly to microbiology, where it can be used to examine the elemental composition of microbial cells. Such studies help elucidate the nutritional requirements and metabolic pathways of different organisms, providing insights that can guide antibiotic development and environmental microbiology studies.
Environmental Studies
SEM EDS analysis is also pivotal in environmental studies, where it aids in analyzing pollutants, sediments, and particulate matter. Understanding the elemental composition of environmental samples is vital for assessing pollution levels and sources of contaminants. It helps in identifying heavy metals in soil and water, offering crucial data for environmental monitoring and remediation efforts.
Furthermore, researchers employ SEM EDS to study mineralogy and geochemistry, providing insights into soil formation and mineral processes. By analyzing rock and soil samples, scientists can make predictions about resource availability, land use, and ecosystem health.
In summary, the applications of SEM EDS analysis across materials science, biology, and environmental studies illustrate its versatility and importance. This technique not only enhances research capabilities but also contributes to practical advancements in various fields.
Challenges in SEM EDS Analysis
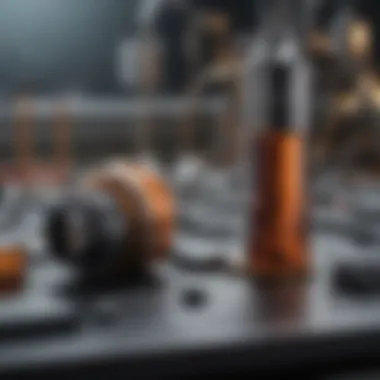
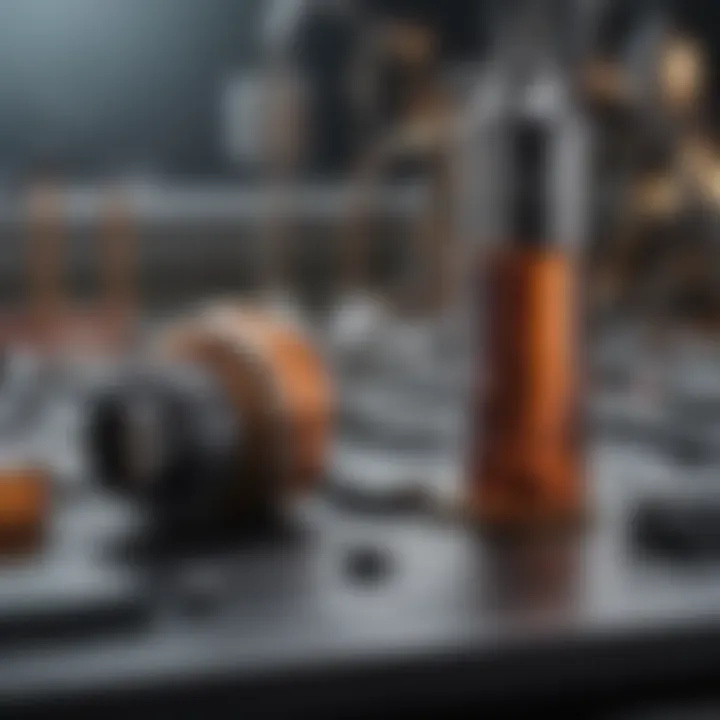
The exploration of Scanning Electron Microscopy combined with Energy Dispersive Spectroscopy (SEM EDS) is vast and significant. However, challenges in its application cannot be overlooked. Understanding these challenges is pivotal for enhancing the effectiveness and reliability of SEM EDS analysis.
Each challenge presents specific elements that impact how results are obtained. Every researcher should weigh these considerations while planning and executing experiments and data interpretation. Acknowledging limitations and potential errors strengthens the overall analytical approach.
Limitations of the Technique
SEM EDS, while robust, has notable limitations. First, its spatial resolution, although high, can be insufficient for nanoscale studies. Techniques like Transmission Electron Microscopy (TEM) may be favored for such analyses due to their superior resolution capabilities. Second, the thickness of the sample can impact the accuracy of the results. Too thick samples may cause absorption of X-rays, resulting in inaccuracies in elemental quantification. Additionally, SEM EDS can struggle with light-element detection. Elements like hydrogen or lithium may not produce detectable X-rays, which limits the material characterization potential.
Here are some specific limitations to keep in mind:
- Depth of analysis: Limited penetration depth often results in surface layer bias.
- Sample charging: Non-conductive samples may charge during analysis, affecting image quality and data accuracy.
- Complex geometries: Irregular shapes and sizes can lead to uneven sampling and challenges in securing reliable data.
Common Errors in Analysis
Common errors in SEM EDS analysis can arise from numerous sources. One frequent issue is improper calibration of the EDS system. Calibration must be conducted properly to ensure the accuracy of elemental quantification. Failing to do so can lead to significant discrepancies in the analysis. Moreover, users may not correctly interpret peak intensities, leading to misleading conclusions regarding elemental composition. It is crucial to distinguish overlapping peaks from different elements to avoid overestimation or underestimation of certain components.
Another critical error can stem from the selection of operating conditions. Settings such as accelerating voltage and beam current must be carefully adjusted based on the sample. Incorrect selections can result in sample damage or poor-quality data.
To mitigate these errors, consider the following steps:
- Regular calibration: Frequent calibration checks ensure accurate measurements.
- Understanding of spectra: A deep dive into spectra analysis aids in better interpretation and identification of elements.
- Proper sample preparation: Engaging in thorough and suitable sample preparation improves overall data quality.
Experts often advise careful attention to these challenges. Overcoming them enhances the overall quality and effectiveness of SEM EDS analysis, paving the way for insightful research and meaningful conclusions.
Future Directions of SEM EDS Analysis
The future of Scanning Electron Microscopy Energy Dispersive Spectroscopy (SEM EDS) analysis is poised to witness significant advancements. As technology evolves, the capabilities of SEM EDS are expanding. Researchers and professionals in various fields are increasingly recognizing the need for enhanced precision and efficiency in their analytical techniques. The future directions will focus on integrating newer technologies, improving resolution, and expanding the applications of SEM EDS in diverse domains.
Emerging Technologies
Emerging technologies are reshaping SEM EDS analysis. New detectors, for instance, enhance the sensitivity and speed of elemental detection. Devices such as parallel EDS detectors allow for quicker acquisition of spectral data without sacrificing resolution. These advancements lead to improved spatial resolution as well, permitting analysis at the nanoscale. Another relevant technology is cryogenic SEM, which maintains a sample's properties by minimizing damage while observing its topography or composition. Additionally, advancements in software algorithms improve the accuracy of data interpretation significantly. The integration of artificial intelligence in data processing promises to elevate analytical capabilities. This can reduce human error and enhance user experience.
Integration with Other Analytical Techniques
The integration of SEM EDS with other analytical techniques is an important evolution in the field. Methods like X-ray diffraction, transmission electron microscopy, and atomic force microscopy can complement SEM EDS results, providing a more comprehensive understanding of materials.
- X-ray Diffraction (XRD): When combined with SEM EDS, XRD provides structural information about the crystalline properties of a sample. The combination enhances the ability to characterize complex materials thoroughly.
- Transmission Electron Microscopy (TEM): Integrating TEM with SEM EDS facilitates detailed analysis. TEM allows researchers to view thin samples at atomic resolution, while SEM EDS can identify elemental composition, yielding a more complete picture of a material's characteristics.
- Atomic Force Microscopy (AFM): AFM can provide topographical data that, when coupled with SEM EDS, offers insights into surface properties and composition at a high resolution.
Further research into effective integration strategies will be crucial. By sharing data between methods and enhancing the information obtained from any single technique, researchers can push the boundaries of material analysis.
Culmination
The conclusion serves as a pivotal aspect of this article, synthesizing the key insights derived from the exploration of Scanning Electron Microscopy Energy Dispersive Spectroscopy (SEM EDS) analysis. It encapsulates the fundamental principles and practical applications of this technique, aiming to present a clear perspective on its relevance in a diverse range of scientific fields. Given the increasing complexity of materials and biological systems, understanding SEM EDS analysis offers significant advantages for researchers and professionals alike.
In this article, we have discovered that SEM EDS not only provides high spatial resolution and elemental characterization but also combines these aspects into a cohesive method for analyzing materials at the micro and nanoscale. The ability to visualize samples at various magnifications, coupled with energy-dispersive capabilities, significantly enhances the interpretation of complex data, vital for advancing scientific research.
Moreover, this techniqueโs integration with emerging technologies and other analytical methods presents a fertile ground for future investigations, potentially leading to breakthroughs in various disciplines. The future applications of SEM EDS continue to expand, with implications that could reshape our understanding of material properties, biological interactions, and environmental impacts. This potential for continued development underscores the importance of SEM EDS analysis in contemporary research.
Cumulatively, the understanding of SEM EDS is integral not just for materials science but also for biology and environmental studies. The insights gained from mastering this technique accordingly equip professionals with the tools necessary to innovate and address challenges in their specific fields.
Summary of Key Points
- High Spatial Resolution: SEM EDS provides detailed images of sample surfaces, offering insights into their topography and composition at the microscale.
- Elemental Characterization: This technique enables the identification and quantification of elements present in the sample, which is crucial for various scientific inquiries.
- Operational Procedures: A well-defined workflow includes rigorous sample preparation, operational protocols, and data acquisition, ensuring effective analysis.
- Data Interpretation: Accurate reading of EDS spectra is essential, differentiating between qualitative and quantitative analyses to obtain meaningful results.
- Future Directions: Ongoing advancements and integration with other methods promise exciting developments in how materials and biological systems are studied.
Implications for Future Research
The future of SEM EDS analysis is bright, primarily due to its adaptability and the increasing demand for detailed material characterization. As research progresses, emerging technologies like machine learning and artificial intelligence may enhance data analysis of complex SEM EDS datasets. The automation and refinement of data interpretation processes will likely foster efficiency and precision in results.
Additionally, the collaboration with other analytical techniques, such as X-ray diffraction or atomic force microscopy, can offer multifaceted perspectives on material properties, further enriching the understanding of their behaviors.
Research in fields such as nanotechnology, biomaterials, and environmental science may particularly benefit from these advancements. Addressing challenges related to sample complexity and heterogeneity remains crucial. Future studies should focus on exploring innovative methodologies for improving sample preparation and analysis techniques, which could lead to groundbreaking discoveries.
In summary, SEM EDS analysis is not only a powerful tool today but also a key driver for future innovation across multiple research domains.
"The future lies in the hands of those who are willing to grasp the tools of today and reshape them for tomorrow's challenges."
Citing SEM EDS Literature
When referencing SEM EDS literature, it is essential to prioritize sources that present both historical context and current advancements. This dual-layer approach provides clarity and depth. Here are elements to consider when citing:
- Scholarly Articles: Citing peer-reviewed articles is essential. These articles undergo rigorous evaluation by experts and therefore offer reliable data and insights. They help validate the claims made during analysis.
- Books and Textbooks: Books provide broader overviews of SEM EDS methods and principles. They often compile multiple studies, making them valuable for comprehensive learning.
- Conference Proceedings: These sources reflect the latest research, showcasing cutting-edge findings and innovations in the SEM EDS domain.
- Online Resources and Databases: Websites like Wikipedia or Britannica can serve as starting points for background information. However, they should not replace scholarly sources for in-depth research.
Proper citation practices are not just an academic formality; they represent respect for the work of others and help maintain academic integrity. By accurately attributing sources, researchers highlight the collaborative nature of scientific progress, acknowledging previous discoveries that lay the groundwork for new insights.
"References transform research into a shared conversation, blending ideas and fostering innovation."