Research in Physical Chemistry: A Comprehensive Overview
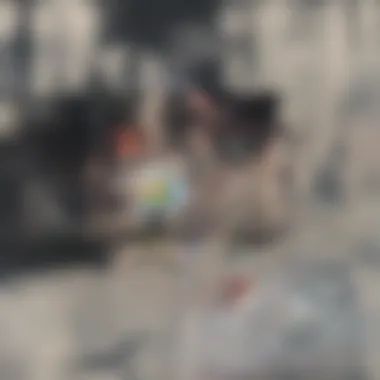
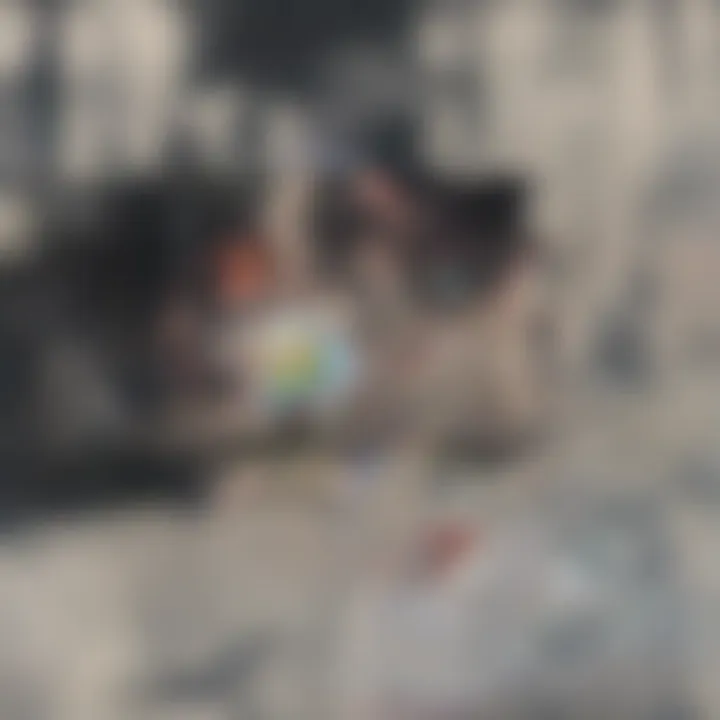
Intro
Physical chemistry occupies a critical space in the intersection between physics and chemistry, bridging the gap with rigorous scientific methods. The scope of research in this field is vast and touches upon various fundamental principles that govern the behavior of matter. As we delve into this topic, we will explore key areas like thermodynamics, kinetics, quantum chemistry, and spectroscopy.
The growing complexity of chemical systems demands sophisticated tools and methodologies, which we will discuss in detail. Understanding the current landscape of physical chemistry research is vital for students, researchers, and professionals striving to contribute to scientific advancements.
Methodologies
Description of Research Techniques
Research in physical chemistry employs numerous innovative techniques to study complex chemical systems. Techniques such as calorimetry provide insights into heat transfer in reactions, while chromatography allows for the separation and analysis of compounds.
Spectroscopic methods, including UV-Vis and NMR spectroscopy, enable the identification of molecular structures and interactions. These techniques are paramount in quantifying reaction rates and energy changes, laying the foundation for a broader understanding of chemical processes.
Tools and Technologies Used
Several advanced tools enhance research capabilities in physical chemistry. Some of the principal technologies include:
- Computational software like Gaussian and VASP, which facilitate simulations in quantum chemistry.
- Mass spectrometers, instrumental in analyzing the composition of chemical substances.
- Differential scanning calorimeters (DSC), used to measure thermal properties and phase transitions.
The integration of these tools into research creates a comprehensive framework for examining chemical phenomena beyond what traditional approaches could achieve.
Discussion
Comparison with Previous Research
Recent studies in physical chemistry build upon a rich tapestry of historical research. By comparing contemporary findings with previous theories, researchers can uncover commonalities and discrepancies, leading to refined models of chemical behavior. Notable studies from the past have set the stage for current techniques, just as modern innovations pave the way for future inquiries.
Theoretical Implications
The implications of physical chemistry research are profound. Not only do the findings contribute to theoretical knowledge, but they also shape practical applications across various industries, from pharmaceuticals to materials science. Current trends often challenge existing theories, prompting revision and adaptation that can enhance our foundational understanding of the subject.
"Research in physical chemistry not only unravels the mechanisms behind reactions but also drives technological innovations that can change the world."
In summary, physical chemistry plays a pivotal role in scientific research. By engaging deeply with its methodologies and implications, the field opens avenues for exploration that are both intellectually rewarding and beneficial to society.
Intro to Physical Chemistry
Physical chemistry is a branch of chemistry that bridges the gap between physics and chemistry, focusing on the physical properties and behavior of chemical systems. This integration allows for a deeper understanding of the nature of matter, its transformations, and the principles that govern these processes. In this article, we will emphasize the significance of physical chemistry in contemporary research, exploring its core concepts, methodologies, and applications that impact various technological advancements.
Definition and Scope
Physical chemistry can be defined as the study of the physical and chemical properties of substances and the changes they undergo during chemical reactions. This discipline encompasses several key areas such as thermodynamics, kinetics, quantum chemistry, and spectroscopy. Each of these areas provides unique insights into the interactions and behaviors of molecules.
The scope of physical chemistry extends beyond simple chemical reactions. It involves the exploration of various states of matter, phase transitions, and the effect of external factors such as pressure and temperature on chemical systems. By understanding these principles, researchers can predict the outcomes of reactions and design new experiments with precision. This discipline not only informs theoretical models but also aids in the development of practical applications in fields such as material science and pharmaceuticals.
Importance in Scientific Research
The importance of physical chemistry in scientific research cannot be overemphasized. It serves as a foundational pillar supporting various branches of chemistry and other scientific disciplines. Here are a few key aspects highlighting its relevance:
- Understanding Fundamental Principles: Physical chemistry allows researchers to grasp the fundamental principles governing chemical interactions at the molecular level. This understanding is critical for advancements in all areas of chemistry.
- Development of New Technologies: Research in physical chemistry has led to innovations in numerous technologies, including drug development, energy storage, and environmental protection. By understanding the mechanisms and properties of materials, scientists can create more efficient and sustainable options.
- Interdisciplinary Collaboration: The principles of physical chemistry inform and enhance various scientific fields such as biology, physics, and engineering. This interdisciplinary approach fosters collaboration, leading to breakthroughs not possible within a single discipline.
"The fusion of physics and chemistry opens a vast realm of possibilities in understanding and manipulating the world around us."
Historical Background
Understanding the historical development of physical chemistry is essential for grasping its current status and capabilities. This field has evolved significantly over the years, driven by various scientific discoveries and innovations. The historical context provides insight into how past research has shaped present methodologies and applications. By examining the origins and progress of physical chemistry, one can appreciate the interconnection between its historical milestones and modern research initiatives.
Development of Physical Chemistry
The roots of physical chemistry can be traced back to the late 19th century. During this era, scientists began to systematically investigate the principles underlying chemical interactions. The convergence of physics and chemistry emerged as researchers sought to explain phenomena using quantitative methods. Key developments included the formulation of the laws of thermodynamics and the introduction of concepts like chemical potential and equilibrium.
One pivotal figure in this development was Svante Arrhenius. His work on electrolytic dissociation laid essential groundwork for acid-base theory and significantly influenced later studies in reaction kinetics. In the early 20th century, the introduction of statistical mechanics by Ludwig Boltzmann provided a theoretical framework that united macroscopic and microscopic views of matter. This period also witnessed the birth of quantum chemistry, which arose from the need to describe electronic structures and their effects on chemical properties and reactions.
Key Figures and Milestones
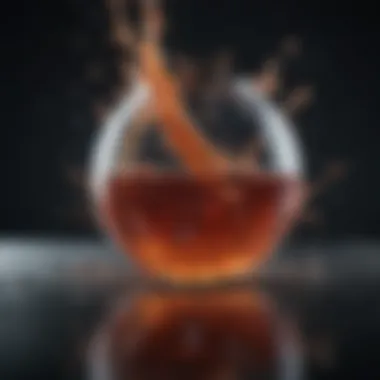
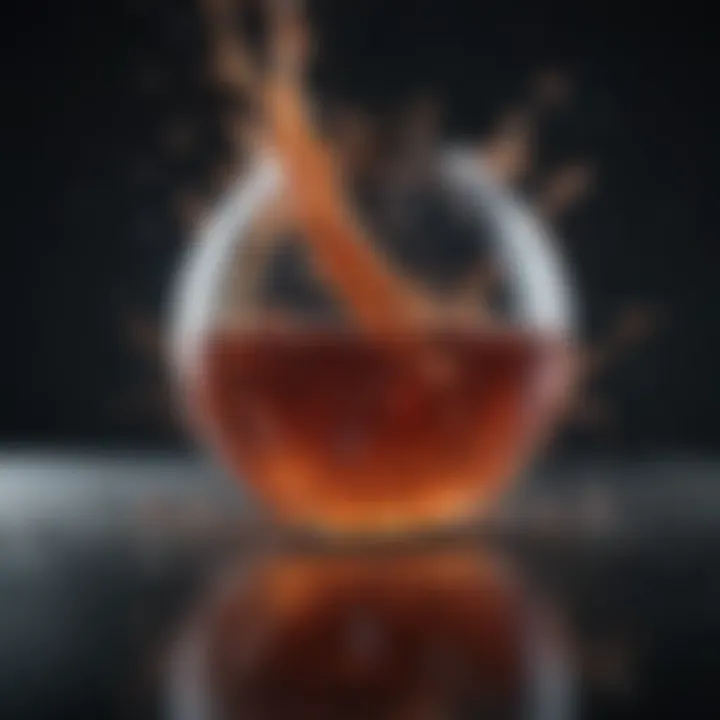
Several notable individuals have contributed to the advancement of physical chemistry. Their research laid the foundations and marked major milestones in the field:
- Daniel Bernoulli: His work in the 18th century laid early groundwork in fluid dynamics, which later influenced thermodynamic studies.
- Julius von Mayer: He formulated the principle of conservation of energy, one of the cornerstone concepts in thermodynamics.
- Gustav Kirchhoff: His contributions to spectroscopy and thermodynamics further advanced understanding of how light interacts with matter.
- Linus Pauling: A notable chemist who utilized quantum mechanics to explain bond formation and molecular structure, shaping modern chemical theory.
The progression from these seminal figures to contemporary collaborative research showcases not only the evolution of theories but also the adaptability of physical chemistry as a discipline.
Quote: "Scientific discovery is not a solitary endeavor; it builds upon the knowledge and insights of predecessors."
The historical background provides a broader context, showing how physical chemistry has continuously adapted to incorporate new technologies and theories. As this field progresses, understanding its development can guide future innovations and interdisciplinary collaborations.
Fundamental Concepts
Fundamental concepts in physical chemistry form the backbone of this discipline. Understanding these concepts is essential for researchers, students, and professionals because they govern how we interpret chemical phenomena and reactions. These concepts provide insight into the underlying principles that explain why substances behave the way they do under various conditions. Engaging with these ideas allows one to predict outcomes in experimental settings, thereby enhancing both theoretical and practical applications of chemistry.
Thermodynamics
Thermodynamics studies energy changes and transfers in chemical systems. This area is crucial in understanding how energy influences reactions and the feasibility of processes. The first law of thermodynamics establishes the principle of energy conservation, stating that energy cannot be created or destroyed, only transformed. As such, thermodynamic principles help in designing chemical processes that maximize energy efficiency.
Understanding thermodynamics is vital for predicting reaction spontaneity and stability, which influence everything from industrial processes to biochemical reactions.
In thermodynamics, key concepts include enthalpy, entropy, and Gibbs free energy. Each plays a unique role in defining how systems interact. For example, entropy aids in understanding disorder within a system, while Gibbs free energy determines if a reaction is energetically favorable under particular conditions.
Kinetics
Kinetics examines the rates of chemical reactions and the factors influencing them. Recognizing how different conditions affect reaction rates can lead to significant advancements in chemistry. Kinetics also provides insights into the mechanisms of reactions, allowing for a deeper understanding of how reactants transform into products.
Factors such as temperature, concentration, and catalysts significantly impact reaction kinetics. Temperature increases often lead to higher kinetic energy, resulting in more frequent collisions among reactants. By studying these factors, researchers can optimize reaction conditions for industrial and laboratory settings.
Quantum Chemistry
Quantum chemistry merges quantum mechanics with chemical systems to explain electronic behavior at the atomic and molecular levels. This field is pivotal in determining the structures, stabilities, and properties of molecules. Quantum chemistry employs mathematical models to predict outcomes that classical physics cannot adequately address.
Key principles of quantum chemistry include the wave-particle duality of electrons and the Pauli exclusion principle, which explain electron configurations within molecules. Understanding these principles equips researchers with the tools needed to explore complex molecular interactions and develop new materials and drugs.
Spectroscopy
Spectroscopy encompasses techniques that analyze the interaction between light and matter. This field is essential for identifying the composition and structure of substances. By studying the light spectrum emitted or absorbed by a sample, researchers can infer critical information about molecular structure and dynamics.
Various types of spectroscopy, including infrared spectroscopy, nuclear magnetic resonance, and ultraviolet-visible spectroscopy, are widely used. Each provides unique insights and has specific applications in fields ranging from pharmaceuticals to materials science. Knowing how to interpret spectroscopic data is paramount for chemists aiming to uncover the intricacies of chemical systems.
Through exploring these fundamental concepts, one gains a comprehensive understanding of physical chemistry's relevance and its profound implications in scientific research.
Methodologies in Physical Chemistry Research
Understanding the methodologies in physical chemistry is crucial. These methodologies dictate how research is conducted, what tools are utilized, and how results are analyzed. They form the backbone of experiments and provide insights into chemical phenomena. Methodologies in this field can be categorized into two primary areas: experimental techniques and computational methods. Each has its significance and plays a vital role in advancing the discipline.
Experimental Techniques
Experimental techniques encompass a wide range of approaches used to gather data and test hypotheses in physical chemistry. These methods are essential for conducting experiments, allowing researchers to observe chemical phenomena in real-time.
Some key techniques include:
- Calorimetry: Measures the heat changes during chemical reactions, providing essential data on thermodynamic properties.
- Chromatography: Separates mixtures to analyze individual components, which is crucial in both research and practical applications.
- X-ray Diffraction: A key tool for determining the structure of crystalline materials, allowing researchers to study material properties at the atomic level.
- Nuclear Magnetic Resonance (NMR): Provides insights into molecular structure and dynamics, useful in various chemical fields.
These techniques are pivotal in obtaining accurate and reliable data. They enable scientists to verify theories and models, thus contributing to the broader understanding of chemical interactions and behaviors.
Computational Methods
Computational methods are increasingly becoming integral to physical chemistry research. They involve using computer simulations to model and predict the behavior of chemical systems. This allows scientists to probe areas that may be difficult or impossible to explore experimentally.
Key aspects of computational methods include:
- Density Functional Theory (DFT): Used for investigating the electronic structure of many-body systems. It is widely employed in material science, aiding in the design of new materials.
- Molecular Dynamics (MD): Simulates the physical movements of atoms and molecules over time, providing insights into dynamic processes.
- Quantum Monte Carlo (QMC): A stochastic method used for calculating properties of quantum systems. This technique can yield highly accurate results for various chemical and physical properties.
The integration of computational methods allows researchers to complement experimental findings and explore theoretical landscapes. It offers a means to test hypotheses and predict behavior, streamlining the research process.
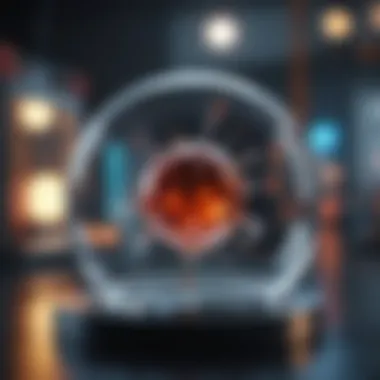
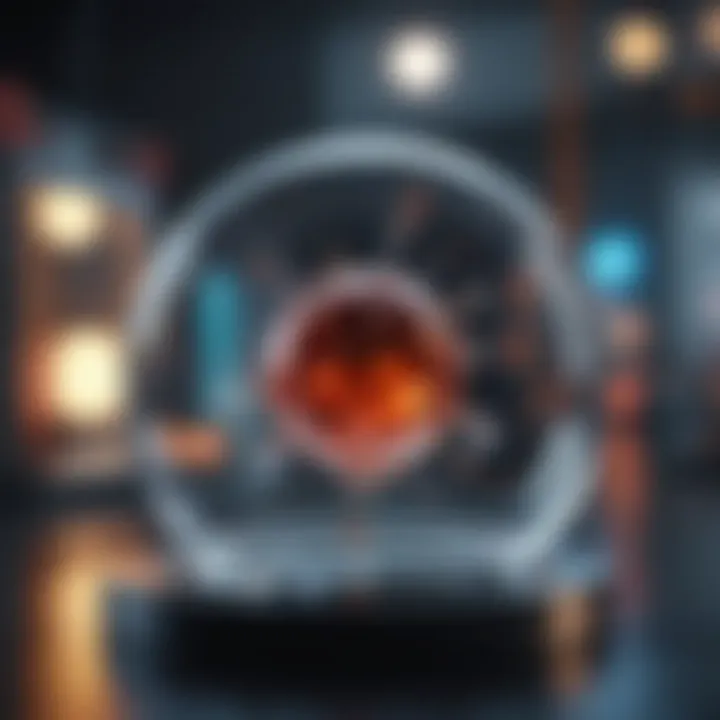
The synergy between experimental techniques and computational methods enhances the scope of physical chemistry research, leading to richer insights and discoveries.
In summary, methodologies in physical chemistry research form a critical part of the study. Experimental techniques provide hands-on data collection, while computational methods offer predictive power. Together, they advance the field, enabling deeper understanding and innovation.
Applications of Physical Chemistry
Physical chemistry occupies a pivotal role in the intersection of chemistry and physics, offering essential insights into numerous scientific domains. The application of principles derived from physical chemistry enhances our understanding of various systems, affecting industries and research sectors significantly. The benefits of applying physical chemistry in practice are manifold, with implications stretching across material science, pharmaceutical sectors, and environmental sciences. This section discusses three prominent areas where physical chemistry plays a crucial role, shedding light on the advantages and considerations pertinent to each field.
Material Science
Material science is one of the foremost sectors benefitting from physical chemistry. The development of new materials requires a deep understanding of their physical and chemical properties. Researchers in this domain study the link between molecular behavior and macroscopic properties, optimizing materials for specific applications. Physical chemistry aids in identifying the structural characteristics that can influence durability, conductivity, and reactivity.
For instance, when developing polymers, physical chemists examine how different processing conditions affect the properties of the final product. They apply thermodynamics and kinetics principles to optimize material performance under varied conditions. This knowledge can lead to significant advancements in electronics, construction, and nanotechnology.
- Application: Energy-efficient materials, semiconductors, and nanocomposites.
- Benefit: Enhanced performance and sustainability of materials used in various products.
Pharmaceutical Chemistry
In pharmaceutical chemistry, physical chemistry is indispensable for drug design and development. Understanding the interactions between drugs and biological systems is vital for creating effective medications. Physical chemistry provides the tools for studying molecular structures and dynamics, essential for predicting how substances behave in the body.
The principles of spectroscopic techniques play a crucial role in drug characterization and quality control. For example, understanding the solubility and stability of drug formulations aids in developing therapies with greater efficacy. By applying physical chemistry methodologies, researchers can design drugs that are not only effective but also safe for therapeutic use.
- Application: Development of targeted drug delivery systems.
- Benefit: Improved patient outcomes through tailored therapies and better drug formulations.
Environmental Chemistry
The realm of environmental chemistry greatly benefits from physical chemistry, particularly in addressing pollution and understanding chemical transformations in ecosystems. Researchers utilize physical chemistry’s analytical techniques to monitor contaminants and assess their behavior in various environmental matrices.
Studying reactions in atmospheric chemistry through physical chemistry principles helps in understanding climate change and air quality. For instance, determining the kinetics of pollutants' reactions can inform strategies for minimizing environmental impact. Techniques such as spectroscopy are employed to identify and quantify pollutants in soil, water, and air.
- Application: Treatment of wastewater and air quality monitoring.
- Benefit: Enhanced methods for managing environmental pollutants and safeguarding ecosystems.
The integration of physical chemistry into these fields not only drives innovation but also contributes to solving critical challenges facing society today.
By engaging with physical chemistry, these applications not only advance scientific understanding but also pave the way for practical solutions to real-world problems.
Recent Advances in Physical Chemistry
Recent advances in physical chemistry illustrate the field's dynamic nature and its essential role in scientific innovation. The importance of recent developments is multi-faceted, influencing not just academic research but also technological applications that benefit society. As our understanding of chemical processes deepens, researchers can better harness these principles for practical use. These advances open pathways for new materials, improved pharmaceuticals, and effective environmental solutions. By staying abreast of these trends, professionals in the field can navigate challenges and seize opportunities that arise from evolving scientific landscapes.
Emerging Trends
Emerging trends in physical chemistry signify the rising focus on sustainable practices and innovative materials. One notable trend is the development of green chemistry approaches. This includes designing chemical processes that minimize waste and conserve energy. Furthermore, nanotechnology is gaining traction, enabling scientists to manipulate materials at atomic and molecular levels for enhanced properties. For example, nanomaterials are being incorporated into catalysts to improve reaction efficiency.
Another trend is the integration of artificial intelligence with chemical research. Machine learning algorithms analyze data rapidly, predicting molecular behavior more efficiently than traditional methods. Such computational advancements allow researchers to simulate reactions and properties, accelerating discovery processes.
In summary, these trends reflect a shift towards efficiency, sustainability, and greater computational integration in research, driving the future of chemical science.
Innovations in Research Techniques
Innovations in research techniques within the realm of physical chemistry have significantly transformed data gathering and analysis. Modern spectroscopic methods, such as 2D NMR (Nuclear Magnetic Resonance) and X-ray diffraction, are providing finer resolutions and insights into molecular structures and interactions. These techniques have shown to offer deeper understanding in various applications, including drug development and material science.
Another important innovation involves the adoption of advanced computational models and simulations. Quantum mechanical calculations have become more accessible through powerful software platforms, allowing researchers to explore complex chemical systems with greater precision.
"The integration of advanced instrumentation and computational techniques is revolutionizing how we conduct research in chemistry, enabling unprecedented levels of accuracy and detail."
The use of high-throughput screening techniques in material discovery presents another noteworthy advancement. This approach allows researchers to evaluate numerous compounds simultaneously, identifying promising candidates for applications quickly. The blend of these innovations fosters creativity and drives exploration in the field.
Challenges in Physical Chemistry Research
Physical chemistry is an intricate field that straddles several scientific domains. It not only addresses fundamental principles of chemistry but also extends into applied chemistry, material science, and other interdisciplinary areas. Despite its importance, researchers face numerous challenges that can significantly affect the pace and quality of discoveries. Understanding these challenges is essential for students, educators, and professionals alike, as they highlight not only barriers but also potential areas for innovation.
Technical Limitations
One major challenge in physical chemistry research is the technical limitations of experimental and computational methods. Researchers often rely on complex instrumentation and simulation software, which can be costly and require significant expertise to operate effectively. The precision of measuring instruments, such as spectrometers, calorimeters, and chromatography devices, can be a bottleneck. Even minor discrepancies in measurements can lead to vastly different interpretations of data.
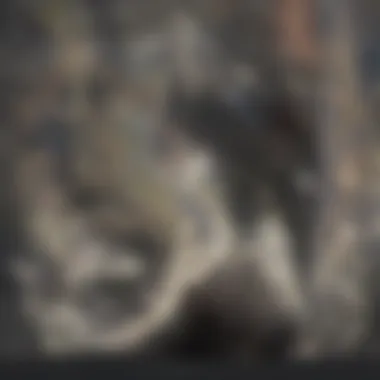
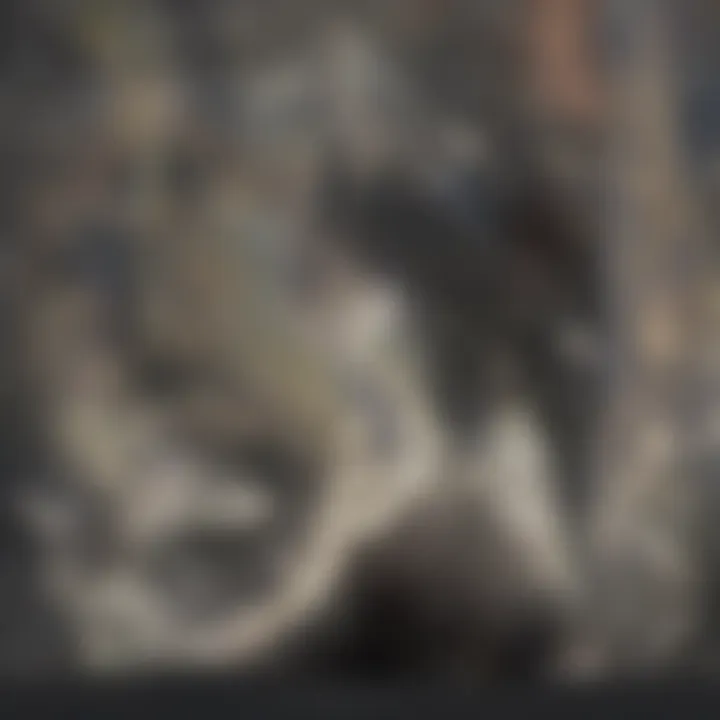
Further complicating this issue are the computational methods that some researchers employ to forecast reactions and processes. For example, density functional theory (DFT) and molecular dynamics simulations necessitate powerful computing resources. They can be time-consuming and may yield results that are sensitive to the quality of the input parameters. Thus, the limitations in both experimental and computational techniques impede the exploration of more complex systems in physical chemistry.
"Technical barriers can stifle innovations in understanding chemical reactions, highlighting the need for constant advancements in instrumentation and computational techniques."
Funding and Resource Allocation
The second challenge lies in funding and resource allocation. Research in physical chemistry often requires substantial financial resources for the acquisition of modern equipment and funding for skilled personnel. Institutions and universities may struggle to secure sufficient funds due to competing priorities, leading to limited research opportunities.
Moreover, funding bodies tend to favor specific projects deemed high-impact or high-visibility. As a result, niche research areas may struggle to obtain the necessary support, which can hinder the advancement of knowledge in critical but less popular fields. This leads to a disparity in research achievements among various areas of physical chemistry. Having a balanced funding strategy is essential to optimize development across the discipline.
Future Directions
The importance of future directions in the realm of physical chemistry is paramount for advancing the field. As scientific challenges evolve, researchers must not only address existing problems but also anticipate new ones that may arise due to technological and societal changes. This section focuses on potential research areas and the critical role of interdisciplinary collaboration in driving innovation in physical chemistry.
Potential Research Areas
Emerging disciplines within physical chemistry offer numerous avenues for exploration. Key potential research areas include:
- Nanotechnology: Investigation of nanoscale materials and their unique properties, leading to innovations in electronics and materials science.
- Green Chemistry: Development of sustainable chemical processes that minimize waste and reduce environmental harm.
- Biophysical Chemistry: Study of biological processes through the lens of physical chemistry, offering insights into drug design and biological mechanisms.
- Energy Storage and Conversion: Research on new materials and processes to improve energy efficiency.
- Computer Modeling: Enhanced modeling techniques to predict chemical behavior and interactions accurately in various conditions.
These potential research areas not only promise advancements in knowledge but also provide practical solutions to pressing global issues. Addressing these fields requires a multidisciplinary approach, fostering collaboration between physical chemists and researchers from related areas.
Interdisciplinary Collaboration
Interdisciplinary collaboration emerges as a fundamental element for future progress in physical chemistry. Integrating insights from biology, physics, engineering, and materials science can lead to comprehensive solutions for complex problems. Collaborative efforts can result in:
- Expanded Knowledge Base: Sharing expertise from multiple disciplines can generate new ideas and improve research quality.
- Innovative Solutions: Combining methodologies from different fields often unveils novel approaches to traditional challenges.
- Resource Optimization: Collaborative projects often have access to broader funding sources and shared resources, enhancing research capabilities.
"The combination of skills across disciplines will lead to breakthroughs in physical chemistry that were previously unimaginable."
The End
The concluding segment of this article encapsulates the essence of research in physical chemistry. This section is critical as it serves to synthesize the information presented throughout the document. By doing so, it enables readers to grasp the importance and implications of the discussed concepts and methodologies.
Summary of Key Points
In this article, we explored several fundamental aspects of physical chemistry, including:
- Thermodynamics, which provides insights into energy transformations.
- Kinetics, focusing on the rates of chemical reactions and how they are influenced by various factors.
- Quantum Chemistry, shedding light on atomic and molecular behavior under quantum mechanical principles.
- Spectroscopy, a powerful analytical tool that aids in studying the interactions between matter and electromagnetic radiation.
Each of these topics contributes to a comprehensive understanding of chemical processes, which is vital for advancements in multiple scientific domains.
Implications for the Field
The implications of research in physical chemistry are vast and significant. The insights gleaned from this field are critical for several areas such as:
- Material design and innovation, impacting engineering and manufacturing.
- Drug development, enhancing pharmaceutical efficacy and safety.
- Environmental monitoring, aiding in pollution control and sustainability.
Advances in methodologies and understanding from physical chemistry research also foster interdisciplinary collaborations, bridging gaps between chemistry, biology, physics, and engineering. This convergence not only enhances the research output but also contributes to the innovative approach to tackling contemporary challenges.
In summary, the discipline of physical chemistry not only enriches our understanding of the microscopic world but also lays the groundwork for innovative solutions in a rapidly evolving scientific landscape.
Citing Sources in Physical Chemistry
Citing sources in physical chemistry is essential for several reasons. First, it helps in acknowledging the work of other researchers and avoiding plagiarism. Every piece of research builds upon existing knowledge, and crediting original ideas is fundamental in scientific discourse.
Moreover, proper citations enhance the reliability of an article. When readers come across cited works, they can easily trace back to the original studies. This transparency fosters trust and allows for independent verification of the information presented.
Citations also facilitate the academic process. By referring to relevant literature, researchers can identify gaps in existing knowledge. This may inspire new hypotheses or experiments, thereby advancing the field of physical chemistry.
"Crediting sources is not merely a formality. It reflects the collaborative nature of scientific research and the importance of building upon foundational knowledge."
When considering how to cite sources, researchers must choose a suitable format, such as APA, MLA, or Chicago style. Each format has its own set of rules and requirements, so it is critical to follow the guidelines relevant to specific journals or institutions.
- Key Considerations for Citing Sources:
- Format adherence: Ensure consistency with citation style.
- Source reliability: Prefer peer-reviewed journals and established publishers.
- Relevance: Select sources that directly contribute to the topic.
Furthermore, using reference management tools, such as EndNote or Zotero, can streamline the citation process. These tools help organize references and format citations correctly, saving researchers valuable time.
In summary, citing sources in physical chemistry not only adds credibility and robustness to research work but also fosters collaboration and innovation in the scientific community. Accurate references improve the reader's understanding and engagement while laying a solid foundation for future research.