Protein Modification: Mechanisms and Applications
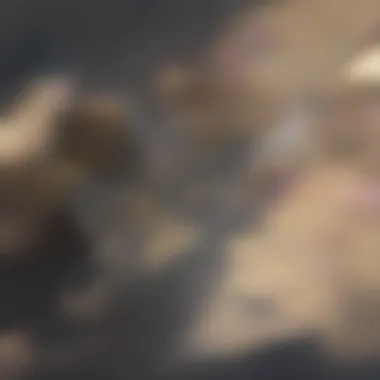
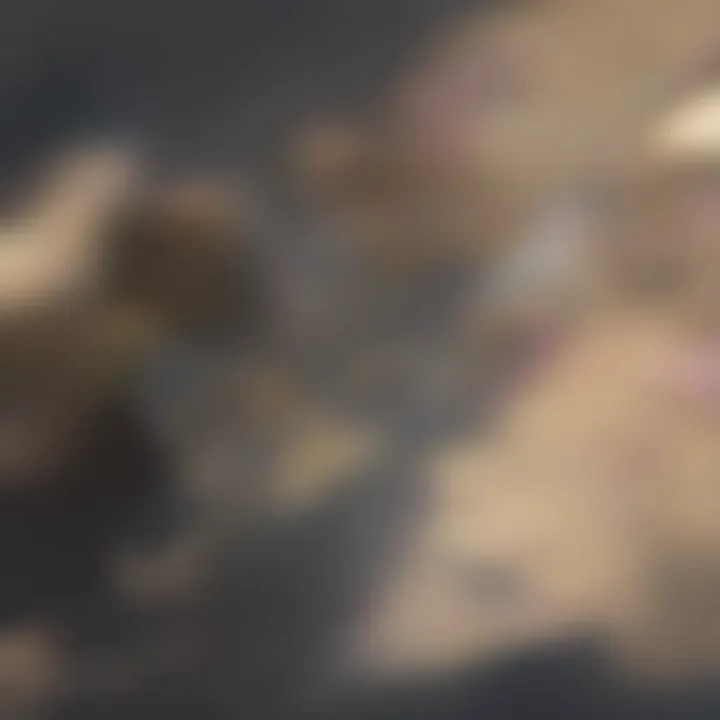
Intro
Protein modification is a crucial bio-chemical process that influences various physiological functions in living organisms. Proteins are not static entities; instead, they undergo several modifications that significantly impact their structure and function. Understanding these modifications is essential for insights into cellular processes, pathology, and potential therapeutic applications.
Post-translational modifications serve as a significant area of interest for researchers as they determine the fate and function of proteins. These modifications can enhance or inhibit activity, alter localization, or dictate protein stability. The mechanisms of protein modification vary greatly, and comprehending these can reveal much about biological systems.
In this article, we explore various aspects of protein modification, emphasizing its mechanisms, importance, and applications in both health and disease. We aim to provide a comprehensive guide that enables students, researchers, and professionals to engage thoughtfully with the topic.
Methodologies
Description of Research Techniques
In researching protein modification, various methods are employed to identify and characterize modifications. Techniques such as mass spectrometry allow for precise analysis of molecular weight, which can reveal modifications. Additionally, techniques like Western blotting are instrumental in detecting specific modifications on proteins, while immunoprecipitation can help purify proteins with certain modified states.
The advent of experimental methodologies like CRISPR and proteomics enables deeper exploration of how these modifications impact biological processes. These advancements highlight an era of precision in understanding protein chemistry.
Tools and Technologies Used
Modern labs utilize a range of tools to study protein modifications. Key technologies include:
- Mass Spectrometry: For identifying and quantifying modified proteins.
- X-ray Crystallography: To visualize three-dimensional structures of proteins and discern modification effects.
- Nuclear Magnetic Resonance (NMR): Enables observation of protein dynamics in solution, which can elucidate how modifications affect functionality.
- Bioinformatics Software: Assists in analyzing large datasets and predicting potential modification sites on proteins.
These tools are vital for advancing research in protein chemistry and have substantial implications for both health-related studies and industrial applications.
Discussion
Comparison with Previous Research
In recent years, there has been a shift in focus towards post-translational modifications as a significant factor affecting protein behavior. Early research predominantly centered on genetic coding of proteins, neglecting the impact of modifications. Recent findings have demonstrated how alterations like phosphorylation, glycosylation, and ubiquitination can dramatically affect protein functionality. These revelations have crucial implications for drug design and therapeutic approaches across various diseases, including cancer and neurodegenerative conditions.
Theoretical Implications
The implications of protein modification extend beyond mere functional adjustments. They challenge the previously held notion that proteins function only based on their genetic sequences. Instead, modifications contribute to a dynamic system where proteins can rapidly adapt to changes in cellular environment or stimuli. This understanding revolutionizes our perception of biological processes and opens new avenues for research and application.
"Protein modification can provide a real-time response mechanism for cell adaptation, crucial for understanding health and disease scenarios." - Insight from leading researchers in protein chemistry
By examining these modifications, researchers can piece together complex biological puzzles, leading to enhanced understanding and innovation in medical science and biotechnology.
Prelude to Protein Modification
Protein modification is a critical theme that underpins numerous biological processes and functions. Understanding this concept allows students, researchers, and professionals to appreciate the dynamic nature of proteins within living organisms. The complexity of protein modification deals with how proteins undergo changes after their initial synthesis, impacting their structure and functions significantly.
The relevance of protein modification extends well beyond basic science. It influences research and applications in biotechnology, drug development, and disease treatment. The mechanisms through which proteins are modified can dictate their roles in cellular activities, making this understanding imperative in various fields, from medicine to environmental biology.
Additionally, protein modification can be crucial for the regulation of metabolic pathways, signaling mechanisms, and the immune response. With the increase in sophisticated techniques for studying these modifications, the insights gathered can help in addressing critical health issues and developing innovative therapies.
Definition and Overview
Protein modification refers to the chemical alteration of proteins post-translationally. It encompasses various processes that change the properties of a protein, thus affecting its function, stability, and interactions. Common types of modifications include phosphorylation, glycosylation, ubiquitination, and acetylation. Each of these processes introduces specific chemical groups to the protein, which can serve distinct roles in signaling pathways and cellular functionality.
Defining protein modification rigorously helps delineate its pathways and effects within biological systems. Each modification type can be classified based on the nature of the change—either permanent or reversible, mild or drastic. Recognizing these variations is essential for understanding protein behavior in different contexts.
Significance in Biological Systems
The significance of protein modification cannot be overstated. It serves as a fundamental mechanism for regulating protein activity and cellular processes. In biological systems, these modifications allow proteins to adapt to changing internal and external environments. For instance:
- Regulatory Functions: Modifications can activate or deactivate enzymes, making them central to metabolic regulation.
- Cell Signaling: Changes in protein structure facilitated by modifications impact cell signaling pathways, influencing responses to hormones and other signals.
- Stability and Localization: Protein modifications can determine the stability of proteins, as well as their localization within cellular compartments.
Moreover, certain modifications play roles in disease states. Aberrant protein modifications can lead to malfunctioning proteins, which may contribute to conditions such as cancer or neurodegenerative diseases.
"Understanding protein modification is key to unlocking potential therapeutic avenues and enhancing biotechnological applications."
Types of Protein Modifications
Understanding the various types of protein modifications is essential in the study of proteomics and molecular biology. These modifications can influence protein function, stability, and interaction with other cellular components. In essence, they provide a layer of regulation beyond genetic coding. Through modifications, proteins can adapt to physiological conditions and play versatile roles in biological systems.
Post-Translational Modifications
Post-translational modifications (PTMs) are crucial processes that occur after the synthesis of proteins. They can alter the chemical structure of proteins, impacting their function and their interactions with other biomolecules. There are several types of PTMs, including phosphorylation, glycosylation, ubiquitination, and acetylation.
Each of these changes can have different consequences. For instance, phosphorylation typically occurs on serine, threonine, or tyrosine residues and plays a significant role in signal transduction pathways. Glycosylation influences protein folding and stability, and it is often essential for proper cellular recognition. The ubiquitin-proteasome system highlights another key type of PTM, where proteins marked for degradation are regulated. This way, PTMs are fundamental in dictating how a protein behaves within a cell and in maintaining homeostasis.
Covalent Modifications
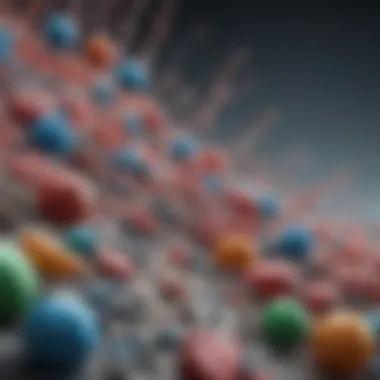
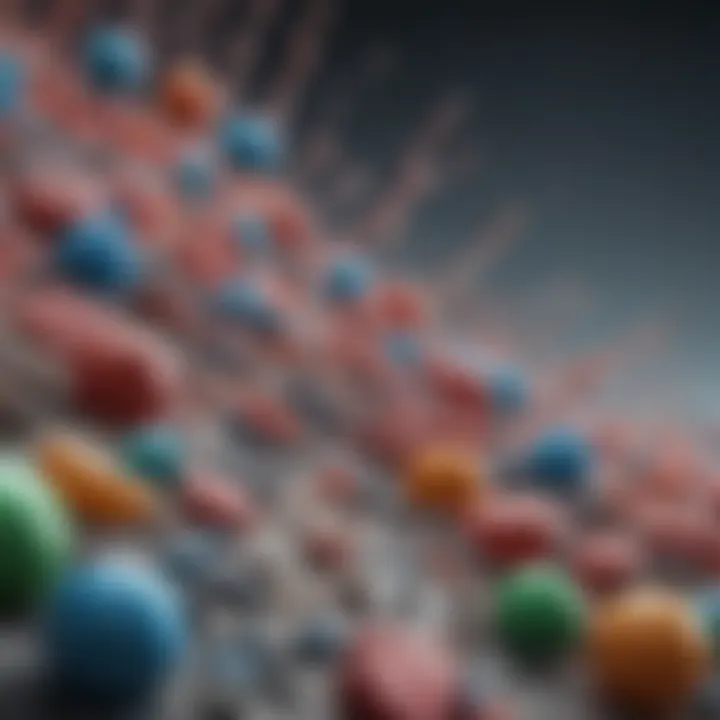
Covalent modifications involve the formation of stable bonds between the protein and modifying groups. Unlike some modifications, which can be reversible, covalent modifications tend to be permanent under physiological conditions. The most recognized covalent modifications include methylation, phosphorylation and acetylation. These modifications can introduce specific functional groups or chemical changes directly onto the protein, which alters its chemical identity.
Covalent modifications have significant implications in regulating enzyme activity and protein interactions. For example, the addition of a methyl group can mask or expose certain interaction motifs, altering how proteins engage with one another. Therefore, understanding these modifications aids in the elucidation of protein functionality and network regulation.
Non-Covalent Modifications
Non-covalent modifications, while lacking the permanence of covalent changes, play an equally important role in protein functionality. These modifications typically include interactions such as ionic bonds, hydrogen bonds, and van der Waals forces. Non-covalent interactions are crucial for the structural integrity of protein complexes and affect protein folding, stability, and dynamics.
These modifications can also facilitate transient interactions between proteins and other biomolecules, allowing for rapid signaling and regulatory pathways. For instance, protein-protein interactions often depend on non-covalent forces, enabling cells to react to signals swiftly. Understanding non-covalent modifications reveals how cells can fine-tune responses to external stimuli, highlighting their critical role in cellular communication.
Mechanisms of Protein Modification
Protein modification is critical in understanding how proteins function in biological systems. The mechanisms by which proteins are modified can have significant implications for their activity, stability, and interactions with other molecules. The complexity of these processes represents a key area of study for researchers interested in protein chemistry and functional biology. By detailing the mechanisms of protein modification, we can appreciate how proteins adapt to various cellular environments and how these modifications can be manipulated for therapeutic and biotechnological applications.
Enzymatic Modification
Enzymatic modifications are largely responsible for the dynamic nature of proteins. Enzymes catalyze reactions that can add or remove functional groups from proteins, effectively altering their structure and function. This includes processes such as phosphorylation, methylation, and ubiquitination among others. Each of these processes is mediated by specific enzymes that typically function under particular biological conditions.
Key Points on Enzymatic Modification:
- Specificity: Enzymes exhibit high specificity for their substrate, ensuring that modifications occur only on target proteins.
- Reversibility: Many enzymatic modifications are reversible. For example, phosphatases can remove phosphate groups added by kinases, allowing for a regulatory mechanism in protein activity.
- Regulatory Role: Modifications can either activate or inhibit protein function, thus influencing metabolic pathways and signaling networks within the cell.
Understanding enzymatic modifications provides insights into cellular regulation and potential therapeutic targets. The role of enzymes in modifying protein behavior cannot be overstated, as these modifications often dictate the fate of the protein within diverse cellular contexts.
Chemical Modification
Chemical modification of proteins can occur through spontaneous reactions or via the action of specific reagents. These modifications often involve covalent changes to the protein backbone or functional groups and can significantly impact protein behavior.
Types of Chemical Modifications Include:
- Oxidation: Changes in the oxidative state of amino acids can alter protein function and lead to loss of activity.
- Cross-linking: The formation of covalent bonds between proteins can influence structural integrity and protein-protein interactions.
- Derivatization: Chemical reagents can attach new chemical groups to proteins, facilitating studies in proteomics or altering therapeutic properties.
Chemical modifications can be more stable than enzymatic ones and might lead to permanent changes in protein function. Understanding these modifications is vital, particularly in developing drugs that can effectively target specific protein forms associated with diseases.
"The ability to chemically modify proteins opens up avenues in therapeutic development and industrial applications, enhancing our approach towards protein science."
Specific Types of Post-Translational Modifications
Post-translational modifications (PTMs) are crucial for the functionality of proteins in a cell. PTMs enhance the diversity of the proteome and allow proteins to perform various roles in biological systems. Understanding the specific types of PTMs helps in delineating their impact on protein function, structure, and interaction networks. This section focuses on the four main types: phosphorylation, glycosylation, ubiquitination, and acetylation. Each modification not only alters the protein's behavior but also plays significant roles in cellular signaling and regulatory pathways.
Phosphorylation
Phosphorylation is a process where a phosphate group is added to a protein. This often occurs on serine, threonine, and tyrosine residues. Phosphorylation is essential in regulating a broad range of cellular activities. It can activate or deactivate enzymatic activity, influence protein-protein interactions, and modulate protein localization within the cell. Phosphorylation is critical for signaling pathways. For example, in response to various stimuli, kinases add phosphate groups to specific proteins, effectively turning them on or off. This process is reversible, as phosphatases remove phosphate groups, allowing for rapid cellular responses.
Glycosylation
Glycosylation is the addition of sugar molecules to proteins, which can enhance stability and affect protein folding. It is one of the most common PTMs. Both N-linked and O-linked glycosylation can occur. N-linked glycosylation happens when sugars are added to the nitrogen atom of asparagine residues, while O-linked glycosylation involves the attachment to the oxygen atom of serine or threonine residues. Glycosylation modulates protein solubility, facilitates cell-cell recognition, and plays a role in immune response. Proper glycosylation is essential; improper glycosylation can lead to diseases, including cancer and congenital disorders.
Ubiquitination
Ubiquitination is the attachment of ubiquitin, a small protein, to a target protein. This modification typically marks proteins for degradation by the proteasome, a cellular structure responsible for degrading unneeded or damaged proteins. Ubiquitination can also regulate cellular processes such as DNA repair and cell cycle progression. The machinery responsible for ubiquitination involves a cascade of enzymes, including E1 (activating), E2 (conjugating), and E3 (ligating) enzymes. Understanding this modification has implications in cancer biology, as alterations in ubiquitin pathways can lead to uncontrolled cell growth.
Acetylation
Acetylation generally occurs on lysine residues of proteins. By adding an acetyl group, this modification can neutralize the positive charge on lysine, which can alter the protein’s interaction with DNA and other proteins. Acetylation plays a significant role in gene expression regulation and chromatin structure. It is also involved in metabolic processes and cellular signaling. Both acetylation and deacetylation are reversible, indicating a highly dynamic system that responds to environmental changes. Its importance is highlighted in various diseases, including neurodegenerative disorders, emphasizing the relevance of studying this modification.
"The extensive range of post-translational modifications provides proteins with the adaptability needed to respond to cellular changes effectively, ensuring proper function across various conditions."
Impact of Protein Modifications on Function
Protein modifications play a crucial role in determining how proteins behave in biological systems. Understanding this impact is vital for anyone engaged in research or applications involving proteins. These modifications can dramatically influence various aspects of protein functionality, including enzymatic activity, protein stability, and interactions between proteins. This knowledge can assist in the development of novel therapies and biotechnological applications, making it a significant topic in protein chemistry.
Alteration of Enzymatic Activity
Protein modifications can lead to a substantial change in enzymatic activity. Enzymes are vital for facilitating biochemical reactions, and their activity is often regulated through post-translational modifications. Phosphorylation, for example, is a common method of enzyme regulation. Through the addition of a phosphate group, enzymes can switch between active and inactive forms.
Furthermore, acetylation can also impact enzymatic properties by affecting the structure and, consequently, the activity of enzymes. An enzyme's ability to catalyze a reaction can be enhanced or diminished based on its modified state. Understanding these processes is crucial as it provides insights into metabolic regulation and the development of pharmaceutical agents targeting specific enzymatic pathways.
Effects on Protein Stability
Protein stability is another important area affected by modifications. Proteins undergo various environmental stresses, and their modifications can dictate their stability under these conditions. For instance, glycosylation enhances protein stability by shielding them from proteolytic enzymes. This modification plays a role in cell-signaling pathways and contributes to the longevity of certain proteins in circulation.
Conversely, certain modifications may render proteins more susceptible to degradation. Ubiquitination, a well-known post-translational modification, marks proteins for degradation. Thus, the balance of protein modifications can dictate the overall stability and lifespan of proteins in cells, influencing cellular functions and responses.
Modulation of Protein-Protein Interactions
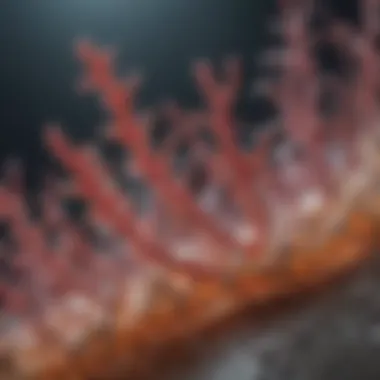
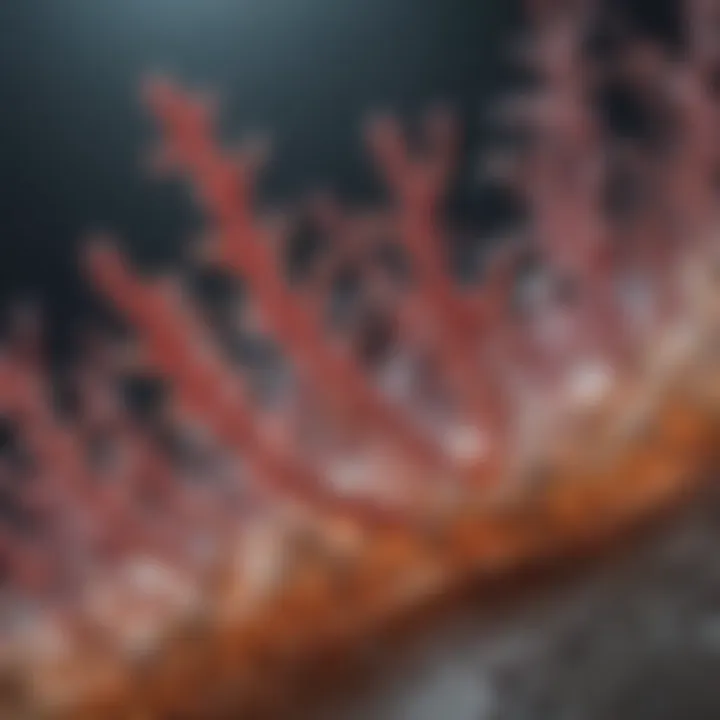
Protein modifications can also significantly affect how proteins interact with one another. These interactions are fundamental for cellular processes, such as signaling and structural integrity. For example, phosphorylation can create binding sites for other proteins, thereby modulating their interaction dynamics. This can have downstream effects on signaling pathways and regulatory networks.
Moreover, modifications like ubiquitination can alter protein conformation, affecting their capability to bind other partners. Through these interactions, the functional outcome of a protein can be drastically altered. Thus, investigating how protein modifications influence these interactions can yield important insights into cellular mechanisms, potentially exposing targets for therapeutic intervention.
Understanding the impact of protein modifications helps in demystifying biological processes that are vital for life.
In summary, the effects of protein modifications stretch beyond mere structural changes. They offer a dynamic regulatory framework that influences enzymatic activities, stability, and interactions. As research progresses, it reveals the underlying complexities of protein chemistry, which carries significant implications for health, disease, and biotechnology.
Experimental Methods for Studying Protein Modifications
Understanding protein modifications is critical for grasping how proteins function biologically. Various experimental methods allow scientists to analyze these modifications accurately. These techniques provide insights into the types of modifications present, their functional implications, and how they interact in complex biological systems. Emphasizing these methods helps bridge the gap between theoretical knowledge and practical application, allowing researchers to derive meaningful conclusions from their experiments.
Mass Spectrometry
Mass spectrometry is a cornerstone technique widely used to analyze protein modifications. It works by measuring the mass-to-charge ratio of ionized particles, enabling scientists to identify various molecular structures. In the context of protein modification, mass spectrometry allows for precise determination of the molecular weight of modified peptides, facilitating the identification of specific post-translational modifications like phosphorylation or glycosylation.
The benefits of mass spectrometry include its high sensitivity and resolution. This means even low-abundance modifications can be detected with ease. Additionally, coupling mass spectrometry with liquid chromatography improves separation, allowing better analysis of complex protein mixtures. However, it requires skilled personnel and significant instrumentation, which can be a limitation for some laboratories.
Western Blotting
Western blotting remains a powerful method for studying specific protein modifications. The technique involves separating proteins by gel electrophoresis and transferring them to a membrane for further analysis. Using antibodies specific to the modified forms of proteins, researchers can visualize the presence and amount of specific modifications.
One key advantage of Western blotting is its specificity, as it allows detection of particular proteins in a sample. It's relatively simple and cost-effective compared to other techniques. However, quantification can be challenging and may not reflect the actual levels of modifications accurately. Therefore, it is often used in conjunction with other methods for comprehensive understanding.
Chromatographic Techniques
Chromatographic techniques, such as high-performance liquid chromatography (HPLC), are essential for separating different protein modifications prior to characterization. These techniques exploit differences in the chemical and physical properties of protein variants, enabling detailed analysis of their modifications.
The importance of chromatographic techniques lies in their ability to purify proteins from complex mixtures. This purification is crucial for subsequent analysis, especially when combined with mass spectrometry or other methods. However, they require careful optimization to achieve the best resolution, which can be time-consuming.
In summary, each experimental method for studying protein modifications offers unique advantages and challenges. Utilizing a combination of these techniques will provide a more comprehensive understanding of protein modifications, paving the way for advancements in both research and therapeutic applications.
"The choice of experimental method ultimately depends on the specific research question, resources available, and desired outcomes."
By embracing the strengths of each technique, researchers can ensure robust data supporting their findings in the complex field of protein modification.
Role of Protein Modifications in Disease
Protein modifications play a crucial role in the context of diseases. These modifications can influence the behavior of proteins in ways that lead to pathological conditions. Understanding how modifications affect protein function helps in identifying potential biomarkers and therapeutic targets.
The implications of protein modifications in disease involve various mechanisms. When protein functions are altered, they can contribute to disease progression or development. For instance, certain modifications can enhance oncogenic properties in cancer cells or disrupt normal cellular processes in neurodegenerative diseases. This section explores the relationship between protein modifications and two major health concerns: cancer biology and neurodegenerative disorders.
Cancer Biology
In cancer biology, protein modifications have significant roles. Cancer cells often undergo changes in the way proteins are modified post-translationally. Phosphorylation, for example, affects cellular signaling pathways that control growth, survival, and division. Aberrant phosphorylation patterns can lead to uncontrolled cell proliferation and resistance to apoptosis.
Key considerations include:
- Oncogene Activation: Some proteins, when modified, can promote oncogene activity. Elevated levels of phosphorylated proteins like HER2/neu may signal aggressive tumor behavior.
- Tumor Suppressor Inactivation: Modifications can also inhibit tumor suppressor proteins. For instance, the ubiquitination of p53 can result in its degradation, removing a vital check on cellular proliferation.
- Metastasis: Protein modifications influence cell adhesion and migration, promoting the metastatic spread of cancer cells.
Understanding these mechanisms carries potential for targeted therapies. Drug developers focus on reversing harmful modifications or enhancing protective ones.
"Accurate profiling of protein modifications in cancer can significantly aid in personalized medicine approaches."
Neurodegenerative Disorders
Neurodegenerative disorders such as Alzheimer's and Parkinson's diseases also illustrate the importance of protein modifications. Misfolded proteins often arise due to improper modifications. For example, tau protein hyperphosphorylation leads to the formation of neurofibrillary tangles, a hallmark of Alzheimer’s disease.
Relevant aspects include:
- Protein Aggregation: Aggregated proteins tend to lose function and exert toxic effects on neurons. Modifications like ubiquitination, for instance, facilitate the degradation processes that can go awry in neurodegenerative diseases.
- Cellular Stress Response: The ability of neurons to handle stress is crucial. Changes in protein modifications can affect stress response pathways, leading to cellular dysfunction and death.
- Inflammation: Some modifications may initiate inflammatory pathways, compounding the damage to neural tissues in diseases.
Research in this area emphasizes understanding how modifications contribute to disease development and progression. This can lead to new strategies in treatment that target specific protein modifications.
In summary, the role of protein modifications in disease highlights a complex interplay affecting cell behavior and state. Insights from cancer biology and neurodegenerative disorders illustrate how critical these changes are to maintaining health and combating disease.
Applications of Protein Modification Understanding
Understanding protein modification is crucial in multiple fields, influencing both basic biological research and applied scientific endeavors. With an in-depth knowledge of how these modifications occur, scientists can improve existing methodologies and develop innovative solutions for contemporary challenges. This is especially relevant for industries such as biotechnology and pharmaceuticals, where the manipulation of protein structures can lead to significant advancements.
Biotechnology and Drug Development
In biotechnology, protein modifications are leveraged to enhance product efficacy. Proteins can be engineered to improve their stability and activity. For instance, monoclonal antibodies often undergo glycosylation, affecting their therapeutic efficacy and serum half-life. Techniques like site-specific mutagenesis can create variants with desired properties, making treatments more effective.

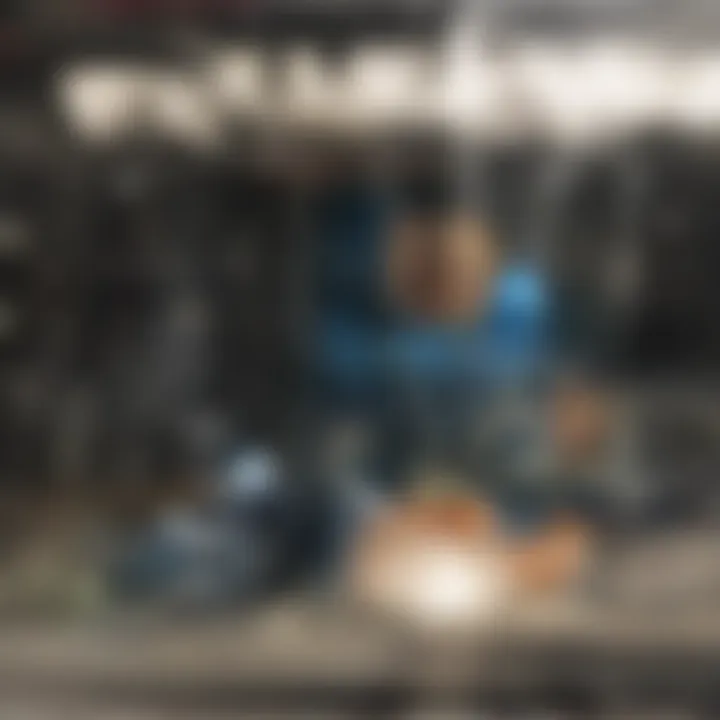
Furthermore, the pharmaceutical industry increasingly relies on understanding protein modifications in drug development.
- Target Selection: Identification of targets for new drugs often hinges on understanding the modified forms of proteins in disease states.
- Drug Design: Knowledge of modification sites allows for the creation of small molecules that can interact specifically with these regions.
- Personalized Medicine: In the sphere of personalized medicine, recognizing how an individual’s proteins are modified can guide treatments tailored to their unique metabolic profile.
Through these avenues, biotechnology and drug development benefit greatly from robust knowlege of protein modifications, resulting in more efficient therapeutic strategies.
Therapeutic Interventions
The implications of protein modification understanding extend to therapeutic interventions, especially in treating diseases characterized by dysregulated protein functions. For example, in cancer biology, aberrant protein modifications often lead to unchecked cell proliferation and survival. Targeting these modifications offers a pathway for novel therapeutic strategies.
Additionally, in neurodegenerative disorders, such as Alzheimer’s and Parkinson’s diseases, post-translational modifications play a pivotal role.
- Potential Targets: Modifications like phosphorylation or ubiquitination can serve as specific targets for intervention.
- Bioavailability: Enhanced understanding can lead to better formulations, ensuring that drugs maintain bioavailability in the presence of modified proteins.
- Predicting Outcomes: Monitoring protein modifications can be useful for predicting therapy outcomes, allowing for dynamic adjustments based on patient response.
In summary, the practical applications of understanding protein modifications are vast. They span the realms of biotechnology, drug development, and therapeutic interventions, ultimately driving innovative approaches to health and disease management.
"The ability to modify proteins offers promising avenues for both therapeutic and commercial advancements in diverse fields."
By applying the principles of protein modification, researchers and professionals can unlock substantial potential in their respective domains.
Recent Advances in Protein Modification Research
The field of protein modification research is dynamic and rapidly evolving. Understanding proteins is pivotal for many biological processes, thus, advances in this area have profound implications. New techniques are constantly emerging that enhance our ability to analyze and manipulate protein modifications. These advances not only improve existing methodologies but also open the door for innovative applications in various fields, including medicine and biotechnology.
Novel Modification Techniques
In recent years, several novel techniques have been developed that significantly enhance our ability to study protein modifications. One notable approach is the use of CRISPR-Cas9 technology for targeted modifications. This method allows researchers to edit protein sequences with high precision, enabling the investigation of specific modifications in native protein environments.
Another promising technique is the application of mass spectrometry paired with advanced bioinformatics. This synergy allows for the identification and characterization of post-translational modifications with unprecedented accuracy. Scientists can now detect a wider range of modifications, helping to elucidate their functional roles in cellular processes.
Furthermore, click chemistry has gained popularity for its ability to tag specific proteins in live cells. This method allows researchers to visualize and track protein modifications in real-time, providing insights into dynamic biological processes.
Advances in protein modification techniques not only enhance our capacity to manipulate proteins but also expand the horizons for therapeutic innovations.
Emerging Trends in Protein Engineering
With the rise of synthetic biology, protein engineering has taken on new dimensions. Specific trends are emerging that highlight the importance of protein modification in creating novel biomolecules. One trend is the design of proteins with enhanced therapeutic properties through selective modifications. This area is particularly relevant for drug development, where modified proteins can exhibit improved efficacy and reduced side effects.
Additionally, the advent of machine learning algorithms is revolutionizing protein engineering. These tools enable researchers to predict which modifications will lead to desired changes in protein function. As a result, researchers can design proteins more effectively, tailoring them for specific applications.
Lastly, efforts are being made to integrate interdisciplinary approaches that combine biology, chemistry, and computational science. This collaboration is leading to innovative modification techniques and a deeper understanding of protein behavior in complex biological systems. The convergence of these disciplines is likely to yield profound advancements in therapeutic strategies and biotechnological applications.
Overall, recent advances in protein modification research highlight a crucial phase in understanding protein functions and interactions. As techniques continue to evolve, they foster opportunities for groundbreaking research and applications, paving the way for enhanced health solutions and biotechnological innovations.
Future Directions in Protein Modification Studies
The exploration of protein modification is continually evolving, raising the need for robust future directions to fully understand its implications. In the increasingly complex landscape of biological research, these directions hold substantial importance. They enhance our comprehension of protein behavior and interaction. Moreover, advancements could also transform therapeutic strategies for diseases where protein function is disrupted.
Challenges and Opportunities
As research progresses, several challenges persist. One significant challenge is the intricate nature of protein interactions. Proteins are not isolated entities; they exist within networks that complicate our understanding of their behavior. This complexity can lead to difficulties when attempting to model or predict outcomes based on specific modifications. Additionally, the technical limitations in current methodologies can restrict the depth of analysis possible in studying these modifications.
However, opportunities abound. The refinement of analytical techniques presents a promising future. Enhanced methods like mass spectrometry and CRISPR technology allow a more detailed exploration of protein modifications. The development of better computational tools can improve our ability to analyze large datasets, leading to new insights in protein functions and modifications. Embracing multi-omics approaches will also uncover novel interactions and pathways that could be targeted for therapeutic purposes.
"Addressing the challenges in protein modification research requires a commitment to innovation and collaboration among disciplines."
Interdisciplinary Approaches
Future studies in protein modification must adopt interdisciplinary approaches. Collaboration between biology, chemistry, and computational sciences is essential. These convergences can facilitate a more comprehensive understanding of protein dynamics. For instance, integrating bioinformatics with experimental biology will enhance the predictive power of modifications on protein behavior.
Moreover, involving researchers from diverse fields can yield unique insights. For example, insights from structural biology can inform biochemical pathways, providing clearer context about modification impacts. Furthermore, input from pharmacology can guide the development of more effective drugs by highlighting how modifications influence interactions with therapeutic targets.
Culmination
In this concluding section, we summarize the critical insights gained from the exploration of protein modification mechanisms and their importance. Understanding protein modifications is paramount for grasping how biological systems operate at a molecular level. Each type of modification, whether it be phosphorylation, glycosylation, or ubiquitination, offers unique insights into cellular processes. These modifications serve not only to regulate protein function but also to dictate the pathways in which proteins participate.
Furthermore, the implications of our findings extend into numerous practical arenas. For instance, in biotechnology, a thorough knowledge of protein modifications facilitates innovations in drug development and therapeutic interventions. It also underscores the necessity of precision in experimental methods. Thus, the importance of accurate techniques such as mass spectrometry and chromatographic methods cannot be overstated; they are essential for elucidating these modifications accurately.
Summation of Findings
The investigation into protein modifications highlights several key findings that are essential to understanding their roles:
- Biological Significance: Protein modifications are vital for the regulation of enzymatic activities and cellular signaling pathways.
- Mechanistic Diversity: Various mechanisms, including enzymatic and chemical modifications, reveal the complexity underlying protein functionality.
- Impact on Health: There are profound implications of modifications in disease contexts, particularly in cancer and neurodegenerative disorders.
- Research Applications: Knowledge of these modifications aids in developing new biotechnological applications and therapeutic strategies.
This synthesis elucidates the multifaceted nature of protein modification and the necessity of continued research in this domain.
Implications for Future Research
The area of protein modifications presents numerous avenues for future research. As our understanding expands, several implications arise:
- Investigation of Novel Modifications: New post-translational modifications may be discovered, which could influence protein functionality in previously uncharted ways.
- Technological Advancements: Ongoing development of analytical techniques promises better quantification and characterization of protein modifications, thereby increasing research accuracy.
- Interdisciplinary Collaborations: Combining insights from fields like bioinformatics, systems biology, and structural biology can enrich understanding and foster innovative approaches.
- Therapeutic Discoveries: There is potential for identifying new therapeutic targets within the modification pathways, particularly associated with maladaptive modifications in disease processes.