Understanding Photo Cross-Linking: Mechanisms and Uses
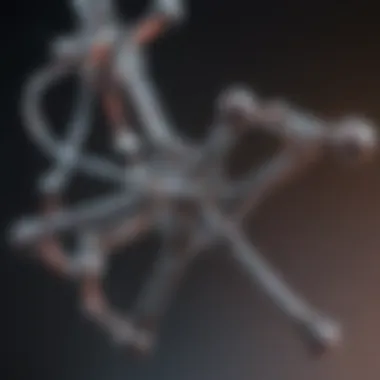
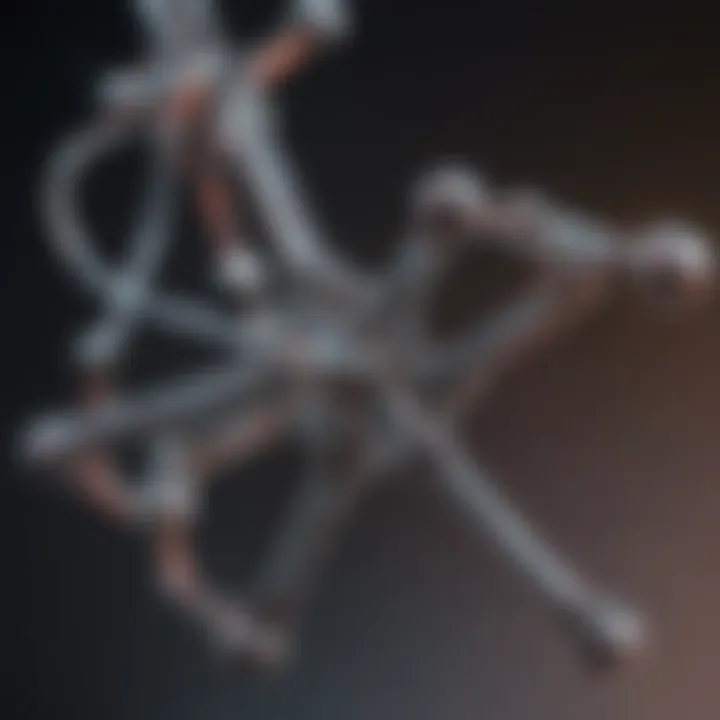
Intro
Photo cross-linking has carved a niche for itself across various fields, particularly in molecular biology and materials science. This technique, which stitches together molecules using light, plays a critical role in shaping the frontiers of research and innovation. Picture this: when certain chemical compounds are subjected to specific wavelengths of light, they undergo transformations that allow them to bond, forming stable structures that were previously unattainable.
The underlying mechanisms of photo cross-linking hinge largely on the reactivity of chromophores—molecules capable of absorbing light. Upon exposure, these chromophores enter an excited state, leading to an array of reactions that result in the formation of covalent bonds. It's a bit like setting off a chain reaction with a single spark, where light acts as the catalyst.
This article will explore the depths of photo cross-linking, spotlighting its applications in drug delivery, tissue engineering, and polymer science. Through various methodologies and recent advancements, the significance of this technique will be laid bare, providing a comprehensive understanding for students, researchers, and professionals in the field.
In the following sections, we will dive deeper into the methodologies employed in photo cross-linking, examine the tools that facilitate this process, and discuss how these insights compare to existing research. By the end, one should gain not only a clearer picture of photo cross-linking itself but also its implications for the future of scientific inquiry and technological advancement.
Preamble to Photo Cross-Linking
Photo cross-linking stands as a linchpin in the realms of molecular biology and materials science, merging the foundational principles of chemistry with cutting-edge technology. This technique enables the formation of robust covalent bonds between molecules through the exposure to light. It embodies a confluence of innovative research that propels advancements in diverse fields, making it a topic of immense relevance.
When examining the significance of photo cross-linking, it is vital to appreciate its profound applications. Not only does it play a central role in drug delivery systems, but it also proves essential in tissue engineering and polymer manufacturing. These applications highlight its versatility and ability to impact real-world challenges.
Another critical consideration is the efficiency and precision that photo cross-linking offers. Unlike traditional methods, where long processing times and extensive chemical reagents might pose hurdles, the systematic use of light for cross-linking provides an elegant solution. This not only enhances operational efficiency but also minimizes waste, making it a more sustainable choice in many scenarios.
The journey through this article will delve into the mechanisms that underlie photo cross-linking, including the types of agents employed and the reactions involved. We will also explore its evolution through history, offering insights into both its foundational principles and key advancements that have marked significant progress in this field.
The significance of photo cross-linking cannot be overstated; it continually shapes how researchers and industry professionals approach challenges, driving innovation in materials and biological applications. As we unfold the layers of this intricate process, we take a closer look at its definition and historical development, laying a solid groundwork for understanding its chemical principles and broad spectrum of applications.
Chemical Principles of Photo Cross-Linking
Photo cross-linking, at its core, involves intricate chemical principles that enable the effective bonding of molecules when illuminated by light. This process is not only critical to advancing various scientific fields but also highlights the adaptability of chemical interactions under specific conditions. Understanding these principles sheds light on the choices researchers and industries make when selecting methods for creating durable and functional materials, making it an essential reading for students and professionals alike.
Mechanism of Action
The mechanism of action in photo cross-linking is where the magic really happens. Essentially, when molecules undergo photo-excitation, the incident light causes specific bonds to break and reform, leading to a rearrangement in the molecular structure. This rearrangement typically results in the formation of new covalent bonds between nearby molecules. A fascinating example of this can be found in proteins; certain amino acids can react when exposed to ultraviolet (UV) light, leading to cross-linking that enhances the stability and functionality of the proteins. It's like a dance where light serves as the conductor, prompting the molecules to come together to create something new.
"The interactions formed through the mechanism of photo cross-linking can significantly improve the properties of biological materials, creating a landscape of possibilities in tissue engineering and drug delivery systems."
This action, while highly beneficial, does not come without its own set of challenges. For one, the efficiency of this mechanism often relies on the specificity of the light source and the surrounding environment. Adjusting factors like wavelength, intensity, and duration of light exposure can dramatically alter the outcome of the cross-linking process.
Types of Photo Cross-Linking Agents
Numerous agents can initiate photo-cross-linking, and each brings its own set of advantages and applications. Common types include:
- Acrylates: Favored for their versatile properties, these agents react swiftly under UV light, making them ideal for rapid curing in industrial applications.
- Benzoin ethers: Known for their ability to form strong bonds, they are often used in creating durable coatings and adhesives.
- Photoinitiators: These compounds are vital, as they absorb light and generate reactive species that facilitate the cross-linking process. Their selection can greatly impact the efficiency of the reaction and the final properties of the material.
Choosing the right agent is crucial. For instance, acrylates might be preferred in applications requiring quick setting times, while other agents may be more suited for environments that demand precision and control.
Photochemical Reactions
Photochemical reactions underpin the entire process of photo cross-linking, dictating how light interacts with the materials at a molecular level. These reactions often involve the formation of reactive intermediates that can readily bond with other molecules. The most common photochemical reactions include:
- Energy transfer: Here, the energy absorbed by one molecule can be transferred to another, exciting it and potentially leading to cross-linking.
- Radical reactions: This type involves the generation of free radicals, which can initiate chain reactions that result in cross-linking.
- Electron transfer: This can result in oxidation or reduction processes, creating reactive species.
The outcomes of these reactions are largely influenced by light wavelength, exposure time, and environmental factors. Optimizing these conditions can enhance the effectiveness of the photochemical reactions, ultimately improving the properties of the synthesized materials.
Understanding the chemical principles behind photo cross-linking provides a window into the sophisticated processes that support advancements in multiple disciplines. It is fundamental to improve material properties and develop systems that can meet specific challenges in various fields.
Biological Applications of Photo Cross-Linking
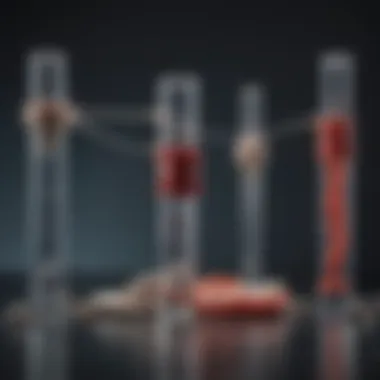
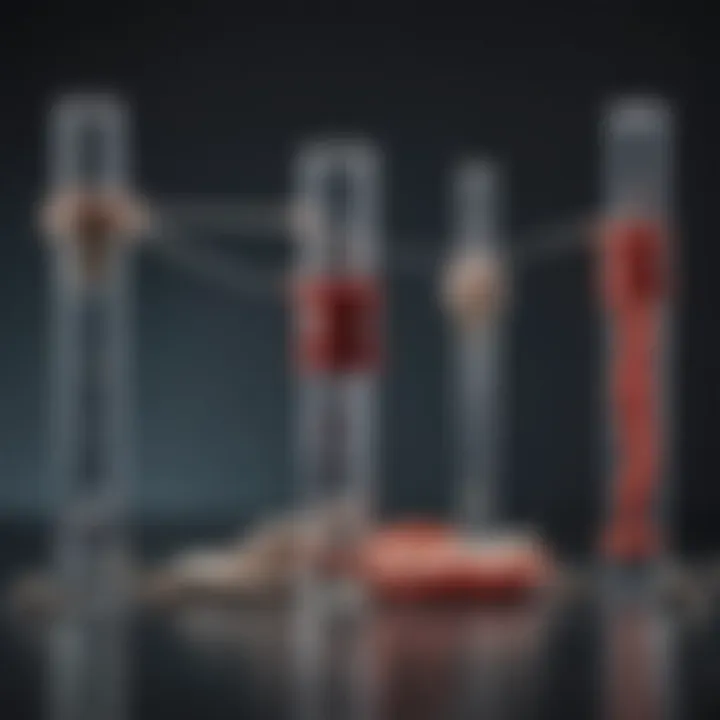
Photo cross-linking serves as a cornerstone in biological research and its applications range from creating artificial tissues to enhancing drug delivery systems. The ability to form stable covalent bonds through light activation offers significant advantages. This section will delve into its relevance in a variety of biological fields, emphasizing specific benefits and considerations that scientists and researchers must keep in mind.
Tissue Engineering
Tissue engineering has come a long way, and photo cross-linking integrates seamlessly into this realm. By allowing for precise control over the spatial arrangement of biomaterials, researchers can mimic natural tissue structures. This method facilitates the repair or replacement of damaged tissues. Unlike traditional methods, which often result in uneven distributions of materials, photo cross-linking enhances uniformity and control.
Key aspects to consider include:
- Biocompatibility: Ensuring that the cross-linking agents used do not elicit adverse responses from surrounding biological tissues.
- Customization: Photo cross-linking allows for tailored constructs. This means that engineers can modify mechanical and biological properties to meet specific requirements for different tissues, such as cartilage or bone.
- Degradation Rates: Optimizing the rate at which the cross-linked material degrades is critical for effective integration into host tissues. Balancing durability and degradability can determine the success of tissue engineering applications.
Photo cross-linking not only enhances tissue compatibility; it also opens doors to cell-specific interactions, prompting cell adhesion and proliferation in engineered tissues.
Drug Delivery Systems
When it comes to drug delivery, photo cross-linking takes center stage. It permits the creation of sophisticated carriers that can release drugs at controlled rates. This level of control is vital, especially in treatments where precision is key, such as cancer therapy.
Consider the following benefits:
- Targeted Release: Photo cross-linking allows for the grafting of light-sensitive moieties that respond to specific wavelengths, maximizing localized targeting of drug delivery.
- Formulating Hydrogels: Hydrogels produced via photo cross-linking can encapsulate drugs, providing a protective vehicle that can dissolve or degrade upon light exposure. This can help in achieving sustained release profiles.
- Bioavailability Improvement: By using this technique, researchers can improve the solubility and stability of compounds that typically struggle in biological environments.
These aspects elevate the potential effectiveness of drug therapies, driving forward improvements in pharmacology and treatment strategies.
Cell Studies and Protein Interactions
Cell studies benefit tremendously from photo cross-linking, particularly when examining intricate cellular interactions and protein dynamics. By utilizing this technique, researchers can study how drugs interact at the cellular level or how proteins engage with one another within cellular structures.
Important elements include:
- Real-time Monitoring: By cross-linking proteins in situ, scientists can observe interactions as they happen, shedding light on processes that were previously elusive.
- Enhanced Resolution: Techniques utilizing photo cross-linking can achieve higher-resolution imaging of cellular components and protein complexes. This is crucial in understanding signaling pathways.
- Functional Studies: The technique allows for functional investigations into how specific proteins affect cellular behavior, contributing to the understanding of diseases and potential therapeutic targets.
Through these applications, photo cross-linking emerges as a transformative tool, not only advancing our comprehension of biological processes but also paving the way for innovative solutions in health and medicine.
Industrial Applications of Photo Cross-Linking
The realm of industrial applications of photo cross-linking is vast and intricate, representing a bridge between scientific innovation and practical use. The strength of this technique lies in its ability to create durable bonds using light exposure, which can fundamentally change the properties of materials used in various industries. This section will explore the importance of photo cross-linking in industrial sectors, particularly focusing on its benefits in polymer manufacturing, coatings and adhesives, and textiles and material development.
Polymer Manufacturing
In the polymer industry, photo cross-linking plays a critical role in the synthesis of advanced materials. This method enhances the structural integrity and performance of polymers, allowing for the creation of products that exhibit superior resistance to heat, chemicals, and physical stress. When polymers are subject to ultraviolet (UV) light, certain molecules can be activated to form interconnected networks, transforming a liquid polymer into a solid state almost instantaneously. This process not only saves time but also boosts productivity significantly.
Key benefits of utilizing photo cross-linking in polymer manufacturing include:
- Shorter production cycles: Rapid curing times mean that products can be processed and set faster.
- Versatility: A wide variety of monomers can be used, catering to specific needs in different industries.
- Improved mechanical properties: Enhanced strength and resistance properties are crucial for many applications.
This method is particularly evident in the production of coatings for tools and machinery, where durability is paramount. The innovations in photo-cross-linked polymers have further encouraged sustainable practices by minimizing waste and optimizing resources.
Coatings and Adhesives
Another significant application of photo cross-linking is in the development of coatings and adhesives. This sector benefits greatly from the ability to create rapid-setting solutions that provide robust adhesion and protective barriers. The use of photoinitiators enables coatings to harden quickly under light, allowing for improved workflow in manufacturing and construction.
The advantages here are substantial:
- Enhanced durability: Coatings created using this method can withstand harsh environmental conditions better than traditional ones.
- Fast turnaround: As the curing process is light-activated, it drastically reduces downtime, enabling quicker project completion.
- Low VOC emissions: Many photo cross-linking processes are designed to be eco-friendly, producing fewer volatile organic compounds compared to traditional methods, which aligns well with contemporary sustainability goals.
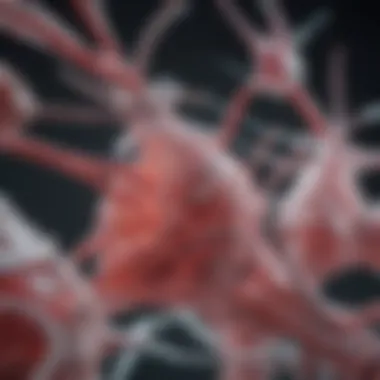
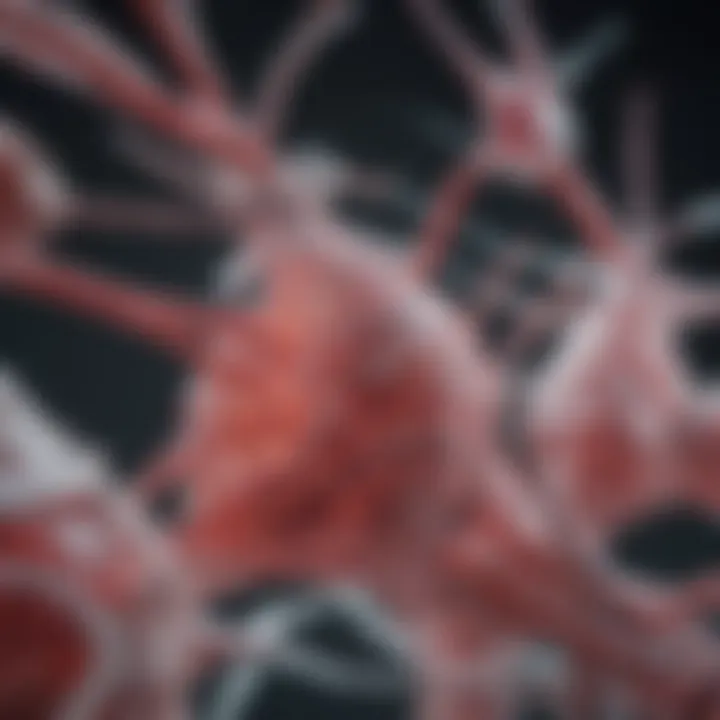
Additionally, these coatings can be applied to a range of surfaces including wood, metal, and plastics, making them particularly valuable across multiple sectors such as automotive and consumer goods.
Textiles and Materials Development
Textiles are no stranger to the transformative effects of photo cross-linking. This technology allows for tailored properties in fabrics, providing them qualities such as increased water resistance, stain repellency, and durability against wear and tear. By integrating photo cross-linking agents within textile manufacturing processes, manufacturers can enhance the performance of fabrics significantly.
Noteworthy points worth mentioning include:
- Customizable properties: By altering the cross-linking agents and conditions, various fabric characteristics can be achieved, tailoring them to specific applications.
- Enhanced lifespan: Fabrics treated with photo cross-linking demonstrate higher resistance to degradation from UV light and environmental pollutants.
- Innovation in smart textiles: Recent advances are paving the way for responsive materials that change properties when exposed to specific light frequencies, opening avenues in healthcare, sports, and fashion.
The infusion of photo cross-linking into textiles has reshaped expectations of what fabrics can do, pushing boundaries of function alongside creativity.
Challenges in Photo Cross-Linking
Photo cross-linking, while a revolutionary approach in various fields, does not come without its fair share of hurdles. Understanding these challenges is critical for refining methodologies and improving outcomes throughout research and industrial practices. This section explores the significant challenges in photo cross-linking, particularly focusing on light absorption inefficiencies, selectivity and control issues, and stability concerns that could affect the long-term viability of products and processes.
Inefficiencies in Light Absorption
One major challenge faced in photo cross-linking is the inefficiency of light absorption during the reaction process. Light is the primary driver for creating covalent bonds, and if the material doesn’t absorb the light effectively, the entire process can be compromised. Some materials may require specific wavelengths of light to initiate the reaction, and when the matching light source isn't available or if there is misalignment in the light characteristics, the desired cross-linking may not occur.
This inefficiency can lead to incomplete reactions, resulting in poor mechanical properties or inadequate functionalization of materials. Moreover, when considering practical applications, such as in tissue engineering, insufficient light absorption can hinder the process of creating biologically compatible scaffolds. Thus, addressing this issue not only enhances the efficiency of the cross-linking process but also ensures the quality and reliability of the end products.
Selectivity and Control Issues
Controlling selectivity during the photo cross-linking process poses another significant challenge. Achieving the desired bond formation without unintended reactions can feel like trying to catch smoke with your bare hands. Many cross-linking agents do not distinguish between different functional groups, which can lead to complex mixtures and unpredictable reactions.
The consequences of lack of selectivity are particularly apparent in biological applications where precise modification of biomolecules is crucial. For instance, when engineering drug delivery systems, it becomes essential to selectively bind to the target sites, avoiding unwanted interactions that could compromise the therapeutic efficacy. Therefore, improving selectivity is essential for the success of applications ranging from nanomedicine to the development of advanced materials.
Stability and Shelf-Life Concerns
Lastly, stability and shelf-life concerns are paramount in the field of photo cross-linking. The materials involved in cross-linking can sometimes degrade under certain environmental conditions, which affects their performance over time. For example, if a cross-linked polymer loses structural integrity due to humidity or temperature fluctuations, the entire product’s utility is at risk. This challenge becomes even more pronounced in the pharmaceutical industry, where active ingredients must maintain their effectiveness throughout their shelf life.
The successful integration of photo cross-linking technologies into widespread applications hinges on addressing these stability concerns. Improved formulations and protective strategies can mitigate these issues, ultimately leading to more robust and reliable photo-cross-linked materials.
Effective strategies are needed to tackle the inefficiencies, selectivity issues, and stability challenges in photo cross-linking, driving innovation and enhancing application viability.
In summary, while photo cross-linking holds immense potential, understanding and overcoming these challenges is essential for advancing its practical applications. With continued research, possible solutions can emerge, leading to enhanced methodologies that ensure greater reliability and performance.
Recent Advancements in Photo Cross-Linking Technologies
The field of photo cross-linking has seen remarkable growth and innovation in recent years. These advancements not only enhance the efficiency of the cross-linking process but also broaden the horizons for applications in various sectors such as medicine and materials science. Understanding these developments is crucial because they reflect the ongoing evolution of this technology in response to current scientific challenges.
Innovative Cross-Linking Agents
Recent years have introduced a variety of novel cross-linking agents that have improved the efficiency and specificity of photo cross-linking reactions. For example, these new agents frequently include tailored photosensitizers and functionalized polymers, which help to achieve controlled cross-linking rates. One such agent is the Diels-Alder click chemistry-based cross-linker that operates efficiently under mild conditions, making it particularly suitable for biological applications.
- Benefits of Innovative Agents:
- Enhanced Selectivity: More targeted binding can lead to better outcomes in drug delivery systems.
- Milder Reaction Conditions: These agents often require less extreme conditions, making them suitable for sensitive biological materials.
- Multifunctionality: Many of these agents offer the capability to introduce various functional groups into the cross-linked network.
These innovations make the application of photo cross-linking agents significantly more versatile and effective, addressing earlier limitations where control over the reaction was lacking and often resulted in unwanted by-products.
Advances in Photolithography
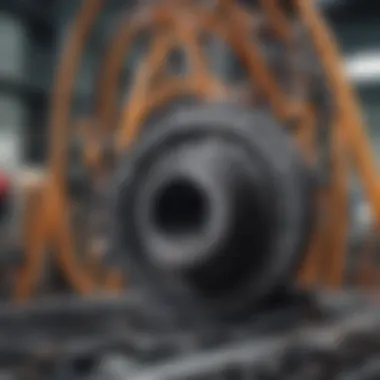
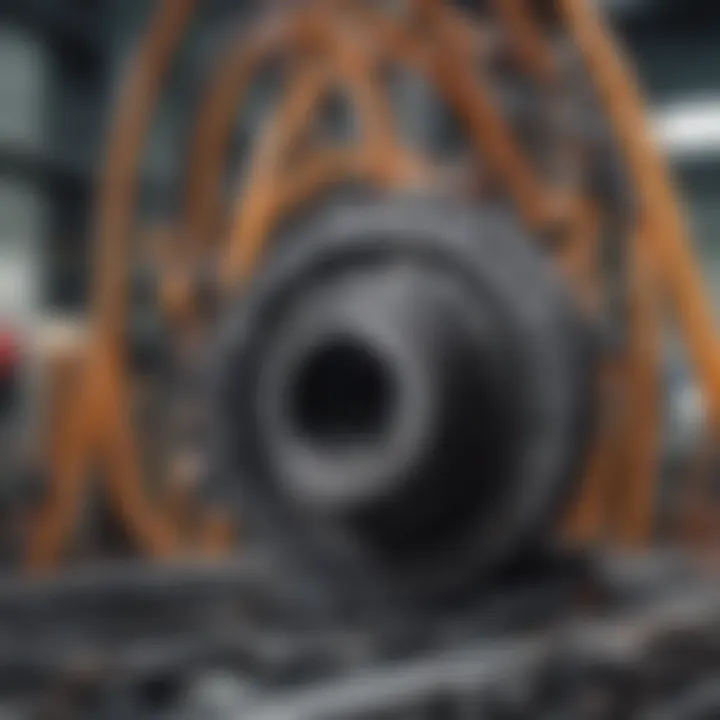
Moreover, photolithography techniques have also evolved, showing promise in the refinement of photo cross-linking processes. Traditional photolithography often struggled with resolution and precision, particularly in microfabricated structures. Recent innovations such as maskless lithography and digital light processing have increased throughput and precision by allowing for custom designs on demand.
- Notable Developments:
- Improved Resolution: New optical components enable finer detail in designs, which is crucial in applications like creating nanoscale scaffolds.
- Adaptive Techniques: Dynamic control systems can adjust parameters in real-time, further enhancing precision in cross-linking.
These advances not only facilitate more accurate structures but also enable the integration of complex geometries that were previously difficult to achieve, expanding the applicability of photo cross-linking in creating intricate tissue engineering scaffolds or specialized coatings.
Multi-Photon and Near-Infrared Techniques
Equally important are the multi-photon and near-infrared techniques that represent a significant leap in photo cross-linking methodologies. Utilizing longer wavelengths of light allows for deeper penetration into biological tissues while minimizing damage. This resilience is key when considering the sensitive nature of most biological systems.
- Key Advantages:
- Increased Penetration Depth: Enable the accessibility of cross-linking within thick tissues, which was previously nearly impossible with traditional methods.
- Reduced Photodamage: Lower energy light minimizes the risk of damaging surrounding cells during the cross-linking process.
These methods open the door for future applications in medical imaging, targeted therapy, and even advanced procedures in materials science where thick sections must be cross-linked without compromising structural integrity.
Recent strides in cross-linking technology pave the way for impressive breakthroughs in numerous fields from biomedicine to complex material formulations, showcasing the dynamic potential and importance of continued research in this area.
Future Directions in Photo Cross-Linking Research
Photo cross-linking is at a fascinating crossroads of research and application, with various avenues waiting to be explored. As we look to the future, understanding the potential directions this field can take becomes essential for scientists and industry experts alike. The importance of this topic resides not only in its implications for innovation but also in how it connects disparate fields ranging from medicine to materials science.
Exploring New Applications
One of the most exciting prospects in photo cross-linking research is the exploration of new applications. Scientists are actively looking beyond traditional uses, such as in drug delivery and tissue engineering, to see what other fields might benefit from this technology. For instance, in the realm of biodegradable plastics, photo cross-linking can facilitate the development of materials that break down safely in the environment, a priority as pollution becomes a global concern.
Applications in bioimaging have also gained traction, where photo cross-linking can enhance contrast and resolution in imaging techniques. This allows researchers to visualize molecular processes in real-time, significantly improving our understanding of cellular functions.
On another front, combining photo cross-linking with 3D printing presents vast opportunities. This integration can lead to the creation of custom designs and structures for implants or prostheses, tailored specifically to the needs of individual patients, thereby pushing the boundaries of personalized medicine.
Integration with Other Technologies
As technology evolves, the importance of integrating photo cross-linking with other techniques cannot be overstated. Combining it with nanotechnology, for example, allows for the development of nano-scale materials that could revolutionize drug delivery systems. This synergy can result in targeted therapy, where drugs are delivered precisely to affected cells, reducing side effects and improving treatment efficacy.
Moreover, merging photo cross-linking with optogenetics—a technique that uses light to control cells—can enable new levels of precision in manipulating cellular behavior. This integration could advance studies in neural networks, paving the way for breakthroughs in understanding diseases such as Parkinson's or Alzheimer's.
Sustainability Considerations
In an era where sustainability is paramount, it's crucial to factor in the environmental impact of photo cross-linking technologies. Research is ongoing into the development of eco-friendly photo cross-linking agents, which minimize toxic byproducts during production and application. Emphasis on biodegradable materials in photo cross-linking can lead to significant strides towards more sustainable practices.
- Renewable resources: Developing agents derived from renewable resources for photo cross-linking can reduce dependence on fossil fuels.
- Energy efficiency: Advancements in LED technologies could make photo cross-linking methods more energy-efficient, reducing the carbon footprint associated with their use.
In essence, as we forge ahead in photo cross-linking research, the topics of integration with emerging technologies and sustainability are not just optional considerations—they are becoming fundamental to the future of this field. Understanding these dimensions will surely enhance the applications of photo cross-linking, contributing to innovations that align with both societal needs and environmental responsibilities.
Closure
The conclusion encapsulates the profound importance of photo cross-linking in contemporary science and technology. It synthesizes the key insights drawn throughout the article, connecting various threads from the mechanisms and applications explored in prior sections. This technique has emerged as a cornerstone in molecular biology and materials science, proving indispensable in areas ranging from drug delivery systems to polymer manufacturing.
Summary of Key Points
- Fundamental Mechanisms: The intricate chemical processes involved in photo cross-linking lay the groundwork for its application in various sectors.
- Diverse Applications: Ranging from tissue engineering to industrial manufacturing, this method showcases versatility in enhancing product performance and functionality.
- Challenges Addressed: Understanding the hurdles, like inefficiencies in light absorption and control issues, helps pave the way for innovative solutions.
- Emerging Technologies: Recent advancements, including innovative cross-linking agents and near-infrared techniques, signal a push toward improving efficiency and broadening the range of applications.
Final Thoughts on the Impact of Photo Cross-Linking
"Photo cross-linking is not just a technique; it's a gateway to the future of material science and biomedicine."
Understanding the broad scope and implications of photo cross-linking encourages an interdisciplinary approach, stimulating deeper collaborations among researchers and industry professionals. This collective endeavor ensures that the knowledge and innovations surrounding photo cross-linking will have a lasting and transformative effect on science and society.