PCR in DNA: Techniques and Applications Explained
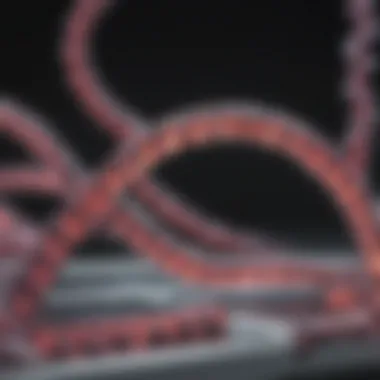
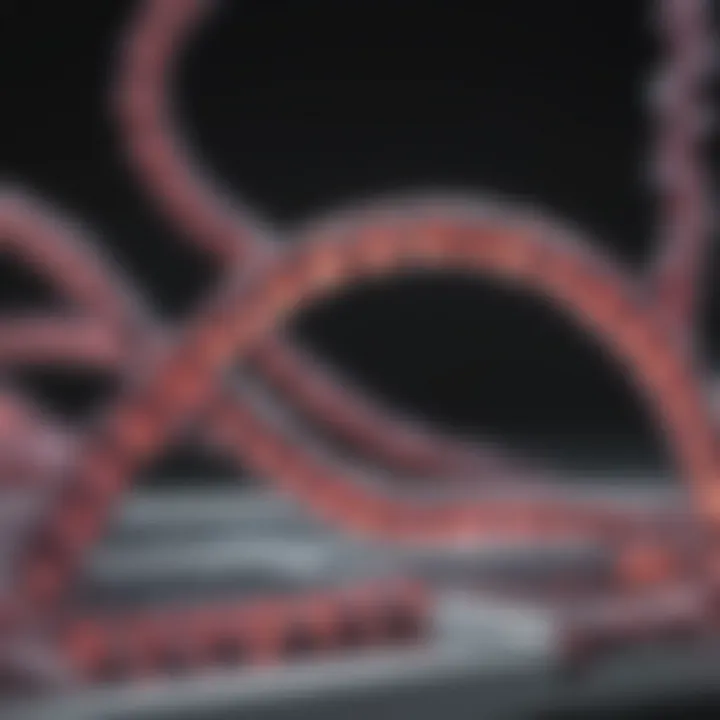
Intro
The emergence of Polymerase Chain Reaction (PCR) has revolutionized molecular biology, allowing scientists to amplify specific DNA sequences with remarkable accuracy. Originally developed in the 1980s by Kary Mullis, PCR has evolved into a cornerstone methodology for genetic analysis across various domains including diagnostics, forensics, and basic research. This introduction sets the stage for a detailed exploration of the techniques, methodologies, and implications associated with PCR technology.
As we delve into the subsequent sections, we will break down the fundamental principles at play, investigate the tools and technologies that facilitate this process, and evaluate the broader impact PCR has had on genetics and ethics.
Methodologies
This section will cover the core methods used in PCR, detailing the step-by-step processes that enable the amplification of DNA.
Description of Research Techniques
PCR operates on a simple yet powerful principle: repeated cycles of heating and cooling. The process generally involves three key steps—denaturation, annealing, and extension.
- Denaturation: This initial step requires heating the reaction mixture to around 94-98 degrees Celsius, resulting in the separation of the double-stranded DNA into single strands.
- Annealing: The temperature is then lowered, typically to 50-65 degrees Celsius. During this phase, short DNA primers bind to the specific target sequence on the single-stranded DNA.
- Extension: Finally, the temperature is raised to about 72 degrees Celsius, allowing the DNA polymerase enzyme to synthesize new DNA strands from the primers.
This cycle is repeated for 20 to 40 times, producing millions of copies of the target DNA sequence. Researchers can utilize different variations of PCR, such as Real-Time PCR, Reverse Transcription PCR, and others, each tailored for specific applications.
Tools and Technologies Used
A successful PCR requires several key components and tools that work in tandem.
- DNA Polymerase: The enzyme responsible for synthesizing new DNA strands. Taq polymerase is one of the most common enzymes used due to its heat stability.
- Primers: Short sequences of nucleotides designed to bind to specific regions of DNA.
- Thermal Cycler: A machine that precisely controls the temperature for each step of the PCR process.
- Nucleotides: The building blocks of DNA that are added to the reaction mixture for synthesis of new strands.
PCR exemplifies a blend of simplicity and sophistication, making it an indispensable tool in modern molecular biology.
Discussion
In examining PCR, it is useful to consider its development in relation to prior genetic analysis techniques and its theoretical implications beyond the lab.
Comparison with Previous Research
Before the advent of PCR, methods for DNA analysis were time-consuming and required larger amounts of sample DNA. Techniques such as gel electrophoresis and Southern blotting were widely used but were less efficient when it came to amplifying small DNA quantities. PCR has addressed these limitations, drastically reducing both the time and material needed for genetic analysis.
Theoretical Implications
The implications of PCR extend into philosophical discussions about genetic manipulation and bioethics. As researchers gain the ability to identify, replicate, and alter genetic material, ethical considerations regarding gene editing and synthetic biology become increasingly relevant. Such capabilities prompt debate about the boundaries of genetic research and the responsibilities of scientists in this evolving landscape.
Prelims to PCR
Polymerase Chain Reaction, commonly known as PCR, has revolutionized molecular biology. This technique, which amplifies specific DNA sequences, underpins many scientific advancements that span various disciplines. By increasing the quantity of DNA, PCR allows for more detailed analyses, ultimately leading to significant breakthroughs in diagnostics, forensics, and genetic research.
Definition and Significance
PCR refers to a technique used to generate thousands to millions of copies of a specific DNA sequence, enabling scientists to study genetic material more effectively. The significance of this amplification lies in its ability to facilitate various applications. For instance, in clinical diagnostics, these amplified DNA fragments can help in identifying pathogens or genetic disorders. Moreover, in forensic science, PCR assists in analyzing minute DNA samples found at crime scenes. This comparison of targeted sequences has made PCR an indispensable tool in modern laboratories.
The technique's crucial role extends beyond just amplification. It has made complex genetic analysis accessible and straightforward, transforming research methodologies. Therefore, an understanding of PCR is vital for students, researchers, and professionals engaged in genetics and cellular biology.
Historical Background
The inception of PCR dates back to 1983 when Kary Mullis first outlined the concept. Mullis aimed to create a method that could replicate DNA segments rapidly without the need for bacterial cloning, which was time-consuming and labor-intensive. Following its introduction, early practitioners recognized the technique's enormous potential, leading to remarkable advancements in genetic research and diagnostics.
Notably, the first practical demonstration of PCR occurred in 1985, utilizing a thermostable DNA polymerase extracted from Thermus aquaticus, known as Taq polymerase. This enzyme was pivotal in making PCR more feasible since it could withstand the high temperatures required during the denaturation stage of the process. The ability to reuse this enzyme in multiple cycles significantly enhanced the reaction's efficiency and reproducibility.
The recognition of PCR's impact culminated in Mullis receiving the Nobel Prize in Chemistry in 1993. The method has since evolved, with numerous adaptations being developed to enhance its accuracy, speed, and applicability in various fields including environmental studies and synthetic biology. Today, PCR stands as a cornerstone of modern molecular biology, illustrating the importance of a technique that emerged from a simple idea.
Principles of PCR
The principles of Polymerase Chain Reaction (PCR) are fundamental to understanding its applications and implications in modern molecular biology. PCR is not just a technique; it embodies several critical concepts that ensure successful amplification of specific DNA sequences. Knowledge of these principles enables researchers and practitioners to harness the power of PCR effectively.
Basic Mechanism
The basic mechanism of PCR operates in a cyclical manner involving three key steps: denaturation, annealing, and extension. In denaturation, the double-stranded DNA unwinds into single strands due to exposure to high temperatures. This step is critical as it prepares the DNA template for the next phase. During annealing, primers bind to the complementary sequences on the single-stranded DNA. This is followed by the extension phase, where DNA polymerase synthesizes new strands by adding nucleotides. This cycle can be repeated multiple times, leading to exponential amplification of the target DNA sequence.
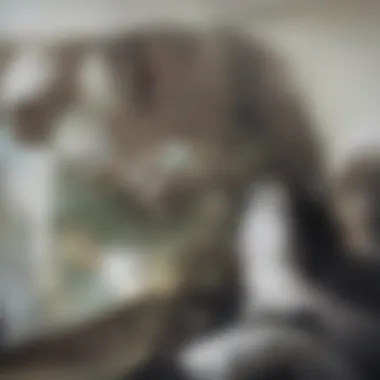
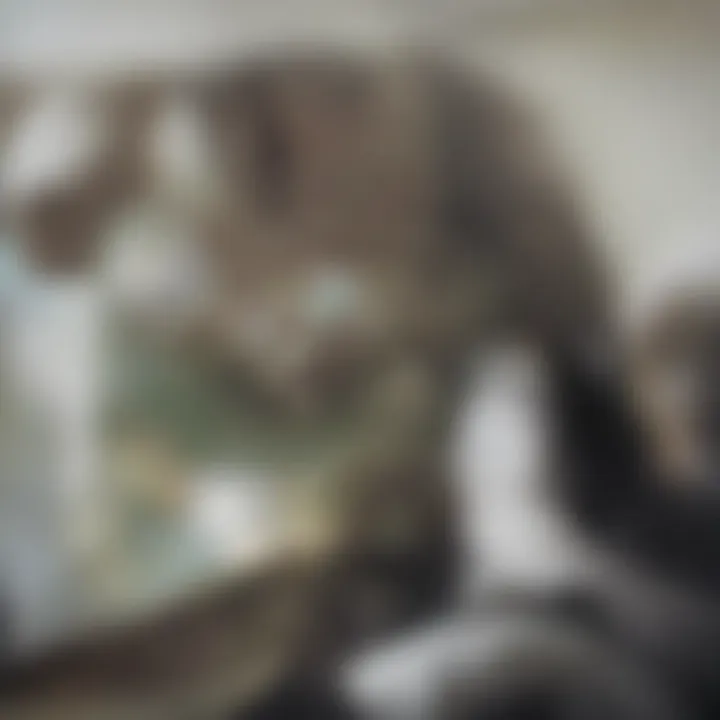
Key Components
Understanding the key components of PCR is essential for optimizing the technique. Each component plays a distinctive role in the success of amplifying DNA sequences.
DNA Template
The DNA template serves as the source of the sequence that needs amplification. Its key characteristic is that it contains the specific region of interest. Using a high-quality template yields better results. One unique feature of DNA templates is their source, which can be from plasmids, genomic DNA, or other sources. The source can impact the efficiency of the PCR, as some templates may be more prone to degradation during the process. Thus, choosing an appropriate template is crucial to achieving reliable results.
Primers
Primers are short strands of nucleotides that initiate DNA synthesis. A primary characteristic of primers is their designed specificity for the target sequence. Primers must be carefully selected to bind effectively under the conditions used in PCR. A unique aspect is that using too many or too few primers can lead to non-specific amplification or failure to amplify the target. Properly designed primers enhance the success of PCR by ensuring high specificity and yield, making them a valuable tool in genetic analysis.
DNA Polymerase
DNA polymerase is an enzyme that synthesizes new DNA strands by adding nucleotides. A key feature of DNA polymerase is its processivity and fidelity; high-fidelity enzymes reduce errors during DNA synthesis. Popular choices include Taq polymerase, which is stable at high temperatures, and Pfu polymerase, known for its proofreading abilities. The role of polymerase is significant; an inefficient enzyme can result in low yields and imprecise amplification. Thus, selecting the right polymerase is fundamental in various applications of PCR.
Nucleotides
Nucleotides are the building blocks of DNA and are essential for the PCR process. Their key characteristic is that they provide the necessary components for new DNA strand synthesis. The use of high-quality nucleotides ensures optimal performance of the PCR. Nucleotides are unique in that their availability can affect the overall amplification. An excess or shortage can lead to imbalanced reactions, impacting the quality and quantity of the amplified product. Ensuring the right concentration is an important consideration when planning for PCR experiments.
Understanding the principles and components of PCR helps researchers navigate the intricacies of this technique, paving the way for innovative applications in various fields.
PCR Process and Techniques
The PCR process and its associated techniques are essential in understanding how this powerful tool enables scientific discoveries across diverse fields. This section elucidates the stages of PCR and the different types of PCR techniques that have emerged, highlighting their unique characteristics and contributions.
Stages of PCR
Denaturation
Denaturation is the first critical step in the PCR process. During this stage, the temperature is increased to separate the two strands of the DNA double helix. This separation is essential for allowing the primers to access the single-stranded DNA template. The high temperature typically ranges from 94 to 98 degrees Celsius, effectively breaking the hydrogen bonds between base pairs.
The key characteristic of denaturation is its role in unwinding the DNA. By providing access to the genetic material, this stage is a vital part of the PCR process. Without it, the subsequent steps cannot occur properly. While denaturation is a beneficial choice due to its effectiveness, it requires precise temperature control to avoid damaging the DNA or affecting the efficiency of the overall reaction.
Annealing
Following denaturation, the annealing stage occurs when the temperature is lowered to allow primers to attach to the complementary sequences on the single-stranded DNA. This step typically occurs at temperatures ranging from 50 to 65 degrees Celsius, depending on the melting temperature of the primers used.
The key characteristic of annealing is its specificity. Primers are designed to be complementary to the target DNA sequences. This ensures that only the desired segments are amplified in the subsequent cycles. While annealing is critical for achieving accurate results, the temperature must be optimized carefully. If it's too low, primers may bind non-specifically; if too high, they may not bind at all, affecting PCR efficiency.
Extension
Extension is the final stage of the PCR cycle, where the temperature is slightly raised again to around 72 degrees Celsius. During this time, the DNA polymerase synthesizes new DNA strands by adding nucleotides to the primers, effectively creating copies of the target region.
The key characteristic of extension is its reliance on the DNA polymerase's efficiency. This enzyme functions optimally at this temperature and can significantly enhance the replication speed. Additionally, the unique feature of extension is that it generates new DNA strands. As a result, the number of target DNA copies doubles with each cycle. However, the extension time must be calculated based on the length of the DNA to be amplified. Longer sequences may require extended reaction times, which can affect overall throughput.
Types of PCR
Standard PCR
Standard PCR is the most basic form of PCR, serving as the foundation for many other types. It involves amplifying DNA through repeated cycles of denaturation, annealing, and extension. This method is widely appreciated for its simplicity and effectiveness.
The key characteristic of Standard PCR is its straightforward nature, allowing researchers to amplify specific DNA sequences without advanced equipment. Its popularity is rooted in its versatility and ability to generate sufficient DNA quantities for various applications. However, the limitation of Standard PCR is that it does not provide real-time data or measurements, which can be a disadvantage in time-sensitive situations.
Real-time PCR
Real-time PCR, also known as quantitative PCR (qPCR), allows for the monitoring of DNA amplification in real time. By using fluorescent dyes or probes, the amount of amplified DNA can be measured during each PCR cycle. This technique is particularly advantageous in various fields, including clinical diagnostics.
The key characteristic of Real-time PCR is its ability to quantify DNA. This feature aids researchers in determining the initial amounts of DNA in the sample. Because of its accurate measurement capabilities, this technique is seen as a critical innovation in modern molecular biology. However, the cost and complexity of the required equipment can be seen as disadvantages, making it less accessible for some laboratories.
Reverse Transcription PCR
Reverse Transcription PCR (RT-PCR) is a variant of PCR designed to amplify RNA sequences. The process begins with the conversion of RNA to complementary DNA (cDNA) via reverse transcriptase. This is followed by traditional PCR steps to amplify the cDNA.
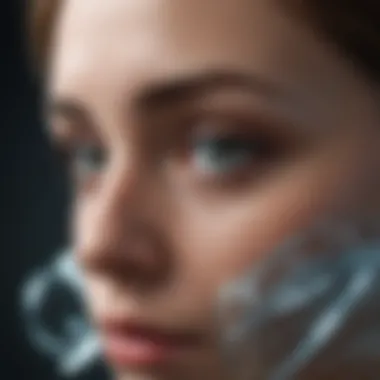
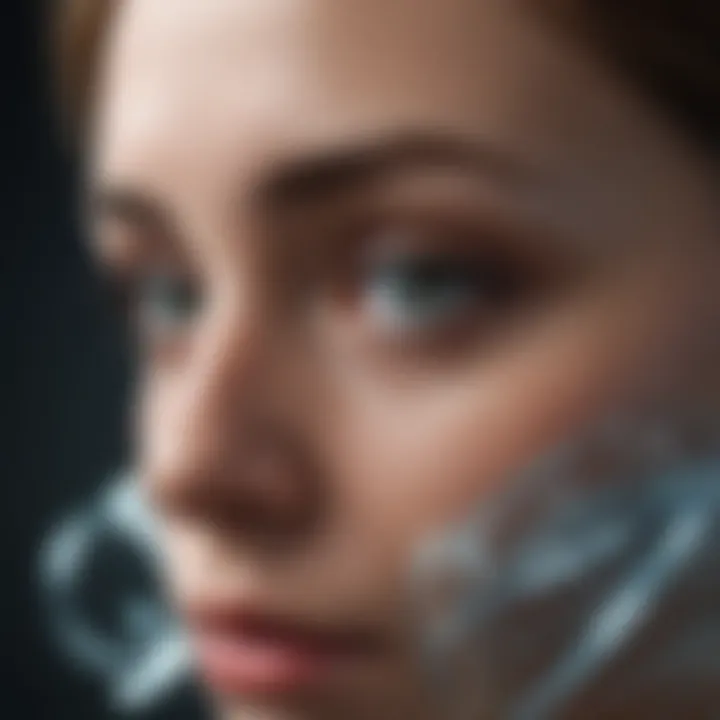
The key characteristic of RT-PCR is its ability to detect and quantify RNA levels in a sample. This is especially useful for studying gene expression and detecting viral RNA. While RT-PCR opens doors for studying RNA, it can be more complex and labor-intensive than standard PCR, as it requires additional steps and enzymes.
Quantitative PCR
Quantitative PCR is similar to Real-time PCR, focusing on quantifying DNA during the amplification process. This technique is crucial for analyzing the amount of specific DNA in a sample. Researchers often use it to assess gene expression levels or detect pathogens.
The key characteristic of Quantitative PCR is its precision. It allows scientists to not only amplify DNA but also to gather quantitative data for analysis. Despite its advantages, this method requires careful optimization to achieve reliable results.
The understanding of PCR processes and techniques is crucial for utilizing this method in research, diagnostics, and various applications in molecular biology.
Applications of PCR
The applications of Polymerase Chain Reaction (PCR) extend across diverse fields, fundamentally transforming how we approach diagnostics, forensics, and research. PCR’s ability to amplify specific DNA sequences with precision serves critical roles in various domains. With its rapid and reliable results, the technique has implications that impact public health, criminal investigations, and various scientific research sectors.
Clinical Diagnostics
Clinical diagnostics leverages PCR in numerous ways, primarily focusing on the detection of infectious diseases and genetic disorders.
Infectious Diseases
PCR is instrumental in diagnosing infectious diseases. It allows for the rapid identification of pathogens like viruses and bacteria. One of the hallmark traits of this application is its sensitivity; PCR can detect minute quantities of DNA and RNA, making it a valuable tool for early diagnosis.
This ability is especially beneficial during outbreak situations, where timely identification is crucial. Furthermore, the speed of PCR can lead to faster treatment decisions, thus improving patient outcomes. The unique feature of this application is its capacity to detect a wide range of pathogens in a single test. However, the downside can include the requirement for highly skilled personnel to conduct and interpret the tests accurately, which can be a resource strain in some medical setups.
Genetic Disorders
In genetic disorders, PCR plays a critical role in the identification of mutations and hereditary conditions. The key characteristic here is precision; PCR allows for the amplification of targeted regions where known mutations may exist. This specificity supports more accurate diagnoses, which is vital for patient management and treatment.
The unique feature is the ability to identify specific genetic markers linked to disorders, enabling predictive testing. However, limitations exist, such as the potential for false positives or negatives, which can impact patient decisions and family planning.
Forensic Science
Forensic science extensively utilizes PCR, focusing primarily on DNA profiling and crime scene investigations.
DNA Profiling
PCR has revolutionized DNA profiling, critical for identifying individuals in criminal cases. This technique amplifies specific regions of DNA known for variability among individuals. The primary benefit of DNA profiling is its high degree of accuracy. The probability of two individuals sharing the same DNA profile is exceedingly low, making it a powerful tool in criminal justice.
The unique feature of this application lies in its ability to work with degraded DNA samples, often found at crime scenes. A challenge is the ethical implications surrounding privacy, especially when DNA is stored in databases without consent.
Crime Scene Investigations
In crime scene investigations, PCR aids in analyzing biological samples. It allows forensic teams to extract and amplify DNA from evidence, such as hair or bodily fluids. The key characteristic of this process is its adaptability; PCR can be applied to very small or degraded samples, which is common at crime scenes. This adaptability enhances the chances of obtaining usable evidence for investigations.
However, the major disadvantage includes the potential for contamination, which can lead to erroneous conclusions about the evidence's source. Strict protocols must be in place to maintain the integrity of samples collected.
Research and Development
PCR also plays an essential role in research and development, particularly in gene cloning and the creation of transgenic organisms.
Gene Cloning
In gene cloning, PCR facilitates the amplification of specific genes for further analysis or modification. The critical characteristic of this process is efficiency; PCR enables researchers to replicate genes quickly and in large quantities. This efficiency is crucial for experiments that require substantial amounts of DNA, such as sequencing or functional assays.
Additionally, the unique features include the ability to modify genetic material for various purposes, including research and therapeutic applications. Limitations can exist, such as the difficulty in amplifying certain sequences, especially those that are repetitive or rich in secondary structures.
Transgenic Organisms
Transgenic organisms are developed through the integration of genes from one species into another. PCR is vital in ensuring precise gene insertion. The key attribute of using PCR in this context is its ability to verify the successful incorporation of transgenes into the host genome. This verification is essential for assuring that the genetic modification has achieved the desired traits in the organisms.
Unique features include enhancing crop yields or introducing desirable traits into plants or animals. However, ethical concerns about genetic modifications and their long-term impacts remain contentious issues.
PCR has touched many aspects of modern science, evolving processes in medicine, forensics, and biological research, highlighting its importance across various fields.
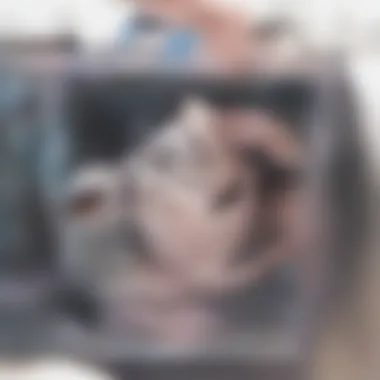
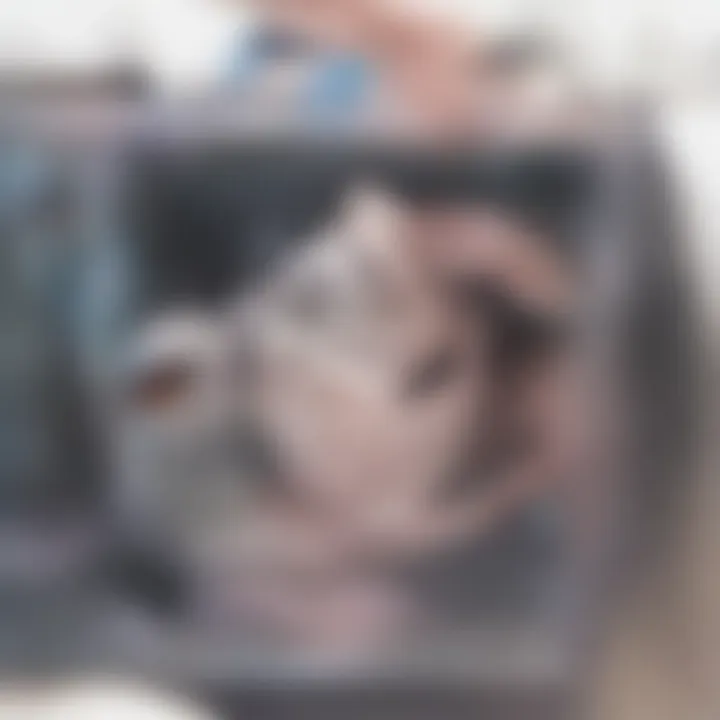
In summary, the applications of PCR range from aiding clinical diagnostics to transforming forensic analysis and even advancing research. The flexibility and precision of this technology continue to drive significant innovations, albeit with challenges in ethics and accuracy that need ongoing discussion.
Limitations and Controversies
Understanding the limitations and controversies surrounding PCR is essential for a holistic view of its role in science. Despite the widespread use of PCR, there are notable sensitivity and specificity concerns that can affect its reliability. Moreover, ethical issues related to genetic privacy and biotechnology ethics arise as the technology advances, prompting deeper discussions in both scientific and social spheres.
Sensitivity and Specificity Challenges
Sensitivity in PCR refers to its ability to detect low quantities of target DNA. Conversely, specificity relates to the technique’s ability to distinguish between the intended target sequence and non-specific sequences. High sensitivity is crucial in clinical diagnostics, where detecting low levels of a pathogen can be critical for timely treatment. However, an overly sensitive test may lead to false positives, which can mislead medical professionals and patients.
In practice, achieving a balance between sensitivity and specificity is challenging. Many factors impact these parameters, including primer design, reaction conditions, and the quality of the reagents used. If primers are not specific to the target sequence, they can bind to non-target sequences, leading to amplification that does not relate to the desired DNA. This phenomenon can skew test results, producing inconclusive or erroneous data.
Ethical Considerations
Ethics intertwined with PCR technology cannot be overlooked. Two significant aspects merit attention: genetic privacy and biotechnology ethics.
Genetic Privacy
Genetic privacy involves the protection of an individual's genetic information from unauthorized access and disclosure. This aspect is particularly pertinent with PCR's capability to analyze and amplify specific genetic sequences. As PCR can reveal sensitive information about an individual's predisposition to diseases or inherited traits, ensuring genetic privacy is paramount.
A key characteristic of genetic privacy is that it is an important safeguard in the age of genetic research and biotechnology. The potential misuse of genetic data is a pressing concern, fueling debates within scientific, medical, and legal fields. The unique feature of genetic privacy rests in its ability to prevent discrimination based on genetic information, which can have significant social implications.
Nonetheless, achieving genetic privacy poses challenges. Data storage systems must be robust, and ethical frameworks must be developed to regulate access to genetic information. Therefore, discussions on genetic privacy are fundamental to advancing PCR applications responsibly.
Biotechnology Ethics
Biotechnology ethics delves into the moral implications of biotechnological advancements, such as those provided by PCR. This field questions the appropriateness of how technology can manipulate biological systems. A prominent characteristic of biotechnology ethics is its focus on the consequences of biotechnological research and applications on society and the environment.
Biotechnology ethics is crucial as it promotes accountability in research and application practices. It leads to policies that safeguard human rights and environmental integrity. However, it also brings unique challenges, as rapid advancements in genetic technology often outpace existing ethical guidelines.
In summary, navigating biotechnology ethics requires ongoing dialogue among scientists, ethicists, and the public to establish a framework that aligns scientific innovation with moral responsibility. The effective integration of ethical considerations with PCR applications will constitute a substantial benefit to the scientific community and society at large.
Future Perspectives
In the realm of molecular biology, the future of Polymerase Chain Reaction (PCR) technology holds immense potential. The progression in this field not only promises improvements in the efficiency and accuracy of DNA amplification but also heralds transformative changes across various applications. As the demand for rapid diagnostics and precise genetic analysis grows, innovations within PCR techniques are becoming increasingly crucial.
Innovations in PCR Technology
Recent developments in PCR technology focus on enhancing speed and reducing the complexity of the processes involved. One notable advancement is the emergence of digital PCR, which offers unparalleled sensitivity and quantification of nucleic acids. This method improves precision by partitioning samples into many individual reactions, allowing for more accurate detection of low-abundance targets.
Another innovative technique is the use of isothermal amplification methods, such as loop-mediated isothermal amplification (LAMP). Unlike traditional PCR, which requires thermal cycling, LAMP can operate at a constant temperature. This not only simplifies the process but also minimizes the risk of contamination, making it suitable for point-of-care testing.
"The array of innovations in PCR technology is reshaping diagnostic capabilities and research methodologies in profound ways."
Moreover, microfluidic technology is being integrated into PCR systems. These compact devices enable the manipulation of fluids at a microscale, allowing for rapid and precise amplification with smaller sample volumes. Such innovations can drastically enhance the accessibility of PCR-based tests, especially in resource-limited settings.
Integration with Next-Generation Sequencing
The integration of PCR with next-generation sequencing (NGS) stands as a significant development in the field. This synergy enhances the capabilities of both techniques, allowing for more comprehensive genomic analyses. PCR provides the necessary amplification needed for NGS, which can analyze millions of sequences simultaneously.
One of the key benefits of this integration is the ability to perform targeted sequencing, where specific regions of interest can be amplified before sequencing. This approach is particularly useful for studying genetic variations linked to diseases, as it ensures that the data generated is not only rich but also relevant.
Furthermore, the compatibility of PCR with NGS facilitates the tracking of variants in pathogen genomes. This has become increasingly relevant in the context of epidemic outbreaks, where real-time genomic surveillance can inform public health responses.
Epilogue
The conclusion of this comprehensive exploration into PCR in DNA delineates the profound role this technique plays in modern molecular biology. As seen throughout the article, PCR is not merely a laboratory method but a cornerstone that underpins various scientific disciplines.
Summary of Key Findings
In summary, PCR enables the amplification of DNA sequences with remarkable specificity and efficiency, which can be applied in multiple fields, including diagnostics and forensic sciences. The innovations in PCR technology have led to a plethora of methodologies, each tailored to meet the growing needs of genetic research and clinical diagnostics. Key findings include:
- Versatility: PCR's adaptability allows it to function in diverse applications, from identifying pathogens in clinical settings to aiding in the development of genetically modified organisms.
- Precision and Sensitivity: The ability to detect minute quantities of DNA makes PCR a powerful tool in forensic science and medical diagnostics, enhancing our understanding and management of diseases.
- Ethical Implications: The widespread use of PCR raises important ethical considerations, particularly in matters of genetic privacy and the moral use of biotechnology.
The Ongoing Impact of PCR in Science
The impact of PCR extends beyond its immediate applications. As a foundational technique, it facilitates numerous advancements in genetic research and biotechnology. Future innovations are likely to further integrate PCR with emerging technologies, like next-generation sequencing. As researchers continue to refine PCR methods, the potential for new applications in personalized medicine, disease prevention, and even environmental science remains expansive.
Importantly, the ethical dimensions surrounding PCR technology necessitate ongoing dialogue among scientists, ethicists, and policymakers. This ensures the benefits of PCR are harnessed responsibly and equitably.
"PCR has reshaped our understanding of DNA, paving the way for breakthroughs that were once considered science fiction."