The Oxidation of Water: Mechanisms and Implications
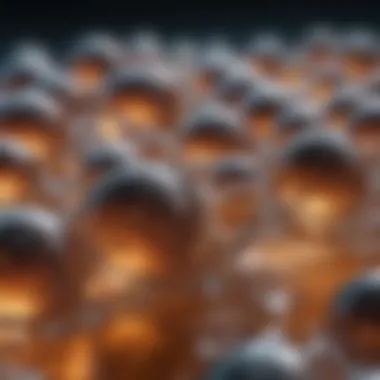
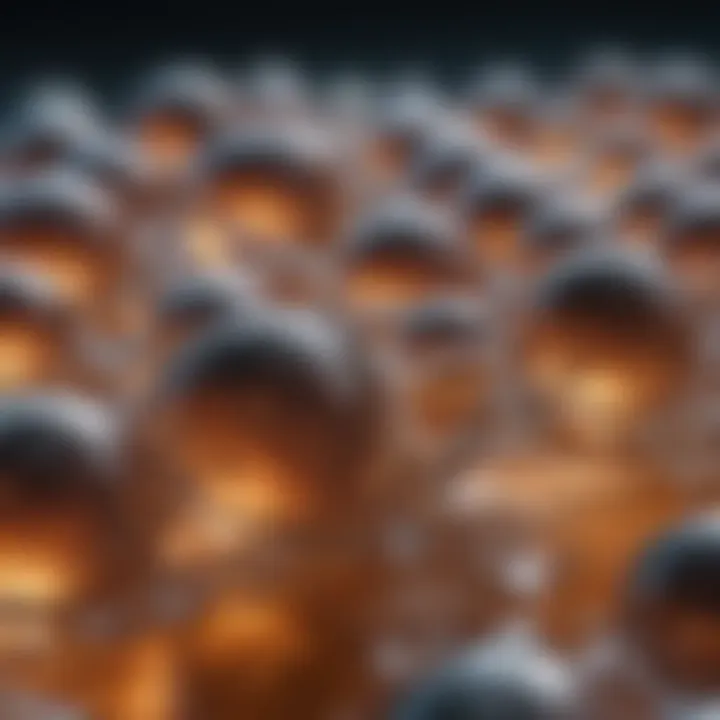
Intro
Water is often considered the essence of life, a fundamental component that plays various roles in both biological and industrial processes. At the heart of many biochemical reactions lies the phenomenon of oxidation—an intricate interplay of atoms and environmental conditions. This article seeks to examine the mechanisms of water oxidation, unraveling the processes behind it and its consequential implications.
The narrative begins by delving into the chemical interactions that define the oxidation of water. Understanding these reactions sheds light on their role in nature, particularly in processes like photosynthesis, where water serves as a crucial substrate. Through exploring the oxidation of water, one gains insight into broader environmental concerns and technological advancements.
The subsequent sections will synthesize key factors influencing these reactions, such as temperature, pH, and catalysis, while comparing these findings with past research to underscore theoretical implications and pave the way for future inquiries.
Methodologies
Accurate examination of the oxidation of water necessitates a utilization of diverse methodologies and tools that enable researchers to dissect and analyze various aspects of this complex process.
Description of Research Techniques
Multiple research techniques have been employed to investigate the oxidation of water effectively. These methods typically include:
- Electrochemical Techniques: These methods help measure the oxidation potential of water by using voltammetry or amperometry. This could assist researchers in understanding the reactivity of water under various conditions.
- Spectroscopic Analysis: Techniques such as UV-Vis, IR, and NMR spectroscopy allow the identification of oxidation products and the observation of real-time changes in molecular structures during reactions.
Tools and Technologies Used
To achieve reliable results, several tools and technologies prove indispensable:
- pH Meters and Temperature Sensors: Essential for monitoring changes in reaction conditions during experiments.
- Chromatography: Used to separate and analyze the various products that result from the oxidation of water.
- Computational Modeling Software: By simulating reactions, researchers can predict outcomes based on varying conditions such as temperature and catalyst presence.
Each of these methodologies contributes to a comprehensive understanding of water's oxidation mechanisms, ensuring a solid foundation for developing insightful implications.
Discussion
Comparison with Previous Research
In the past, studies predominantly focused on water's roles in biochemical pathways, often overlooking the true magnitude of its oxidation reactions. However, recent advances have shifted attention to the intricacies of these dynamics, revealing beyond initial expectations the profound atmospheric and ecological impacts of water oxidation.
Prominent works have indicated a correlation between varying environmental conditions and the efficiency of water oxidation, suggesting that minor alterations can lead to significant shifts in biological and chemical outcomes. For example, researchers have shown that changes in pH levels could dramatically influence the rate of oxidation, highlighting areas for further investigation.
Theoretical Implications
The theoretical implications of water oxidation extend into many areas of scientific inquiry. A deeper comprehension of these processes can facilitate advancements in energy production and ecological restoration strategies. The application of findings from this research could influence technologies, such as fuel cells, which rely on efficient oxidation reactions based on water. Understanding how to enhance water's oxidizing properties may illuminate new pathways for sustainable energy solutions.
"Clean and efficient water oxidation could hold the key to a greener future, providing a strategic avenue for energy production and environmental management."
Prolusion to Water Oxidation
Understanding the oxidation of water is vital for grasping many scientific and industrial processes that define our interaction with the environment. Water, often viewed merely as a life-giving force, plays a more complex role in oxidation reactions that are foundational to both natural and human-made systems. This section sets the stage for a deeper exploration into not only how water undergoes oxidation but also its significant implications for varied disciplines, from biochemistry to environmental science.
Oxidation processes involving water are pertinent in areas such as energy production, photosynthesis, and environmental remediation. The implications reach far beyond the chemistry lab—a solid grasp of these principles can guide effective practices in sustainability and industry standards. Recognizing the significance of how water behaves under different conditions prepares the ground for sophisticated applications in technology and ecology.
Definition and Overview
Water oxidation refers to the process where water molecules lose electrons, which is often coupled with reduction reactions involving other substances. In the grand scheme, this fundamental reaction is crucial in various chemical processes, including photosynthesis in plants, where water is oxidized to produce oxygen—an essential component for life.
Typically, the core reaction can be simplified to:
[ 2 H_2O \rightarrow 4 H^+ + 4 e^- + O_2 ]
In this equation, water (O) is split into protons (H+), electrons (e-), and molecular oxygen (O2). This reaction has broad implications not only in nature but also in various industrial applications, such as in the production of hydrogen fuel or in catalytic processes. Understanding the oxidation of water opens a window into how energy transitions occur and supports life on Earth, as well as underpins crucial industrial reactions.
Historical Context
The exploration of water oxidation is deeply rooted in scientific history. It can be traced back to the early studies of chemists like Antoine Lavoisier, who established foundational concepts of oxidation and reduction in the late 18th century. During this time, the understanding of chemical reactions was rudimentary, yet it laid the groundwork for modern chemistry.
As the 20th century approached, the significance of water oxidation was further highlighted through the advent of the field of electrochemistry. Researchers began to hone in on the role of electrons and their transfer, establishing what we now know as the principles of electrochemical cells. Scientists like Michael Faraday and later on, in the mid-1900s, the work of chemists in the nascent field of biochemistry, particularly about photosynthesis, illuminated the intricate details of how water oxidation is integral to life.
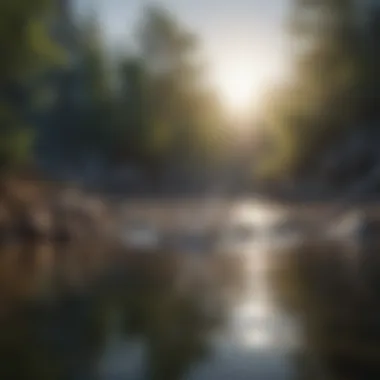
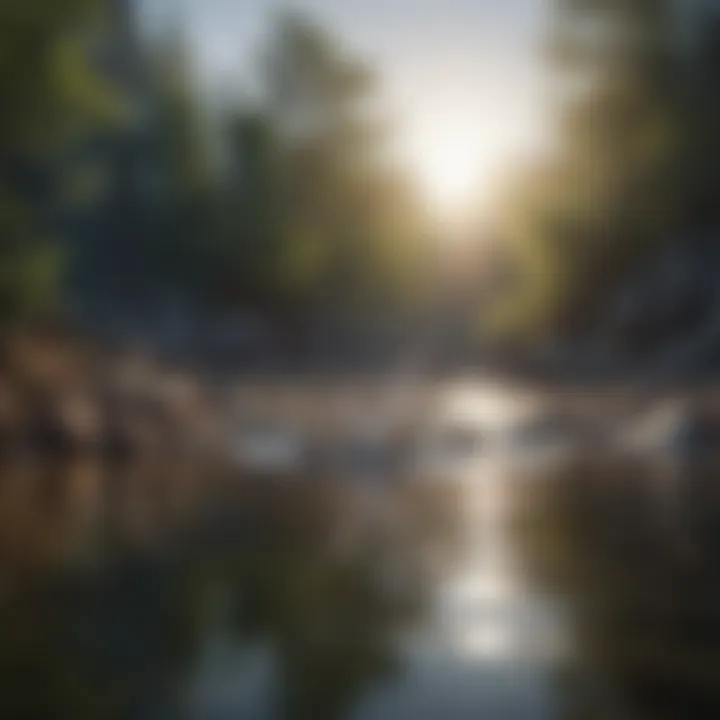
In contemporary times, water oxidation has gained renewed interest, driven by the urgent need for sustainable energy solutions and cleaner industrial processes. The recent developments in catalyst research and technological innovations signal a turning point in how we utilize water in various chemical and environmental contexts. Thus, understanding the historical context not only enriches our comprehension but also situates current research and applications within a broader narrative of scientific discovery.
Chemical Fundamentals
Understanding the chemical fundamentals behind the oxidation of water lays the groundwork for grasping the broader implications and applications of this process. Knowing how oxidation-reduction reactions occur, along with the mechanisms of electron transfer, helps in deciphering both the intricate science and practical relevance of water oxidation in various settings.
Oxidation-Reduction Reactions
Oxidation-reduction, or redox reactions, play a pivotal role in the oxidation of water. At its core, oxidation involves the loss of electrons from a substance, whereas reduction entails the gain of electrons by another. These two processes are inherently linked; when one substance is oxidized, another is simultaneously reduced. This interconnected nature is of immense importance, particularly in environments such as aquatic ecosystems where water serves not just as a reactant, but also as a transport medium for these reactions.
In the context of water oxidation, the relevant reaction can be generally summarized as:
2 O → O2 + 4 H+ + 4 e−
Here, water molecules lose electrons and protons, resulting in the formation of oxygen gas. This reaction is vital not only in natural processes, like photosynthesis, but also in various industrial applications. Understanding the framework of these reactions enables us to fully appreciate the energetic aspects, efficiency, and sustainability of water oxidation processes.
Electron Transfer Mechanisms
Diving deeper into the electronic interplay, we can classify electron transfer mechanisms that facilitate water oxidation into two main categories: direct electron transfer and mediated electron transfer.
Direct Electron Transfer
Direct electron transfer represents a fundamental method where electrons move from the oxidizing agent to the water molecules without intermediates. The key characteristic of direct electron transfer is its simplicity and often higher efficiency when conditions are optimal. This mechanism establishes a more straightforward pathway for the oxidation reactions to happen, which can be particularly advantageous in biological systems where rapid reactions are crucial.
One unique feature of direct electron transfer is its reliance on suitable electrode materials in electrochemical cells. Materials like platinum or gold can provide the necessary conductive environment, allowing for increased rates of reaction. However, while this method showcases several advantages, such as reduced reaction time and energy efficiency, it can also suffer from limitations like over-potential and electrode degradation over time.
Mediated Electron Transfer
On the other hand, mediated electron transfer involves the use of an intermediate species to facilitate the transfer of electrons between the oxidant and water. This approach can be particularly useful when direct transfer is inefficient or impractical. A defining characteristic of mediated electron transfer is the ability to harness various catalysts to improve reaction conditions, making it a popular choice in both biochemistry and industrial applications.
The unique feature here is the versatility provided by catalytic mediators, which can vary widely depending on the environment and requirements of the reaction. Though this method also offers distinct advantages, such as enhanced reaction control, it might come with downsides, such as the dependency on these additional species which can complicate the system.
Through this exploration, one sees how critical these chemical fundamentals are in not only elucidating the oxidation of water but also in paving the way for broader applications, from energy generation to ecological sustainability.
Factors Influencing Water Oxidation
Understanding the factors that influence the oxidation of water is crucial, as it impacts not only chemical processes but also biological and industrial applications. This section highlights essential elements that govern how water undergoes oxidation, with attention to their significance and consequences. Each factor plays a unique role, creating a complex interplay that determines the efficiency and outcomes of oxidation reactions.
Thermodynamics of Oxidation
Gibbs Free Energy
Gibbs Free Energy (G) serves as a fundamental concept in assessing the spontaneity of oxidation reactions. This thermodynamic parameter helps predict whether a given reaction can occur under constant temperature and pressure conditions. In the context of water oxidation, a negative change in Gibbs Free Energy indicates that the process is thermodynamically favorable.
One key characteristic of Gibbs Free Energy is that it encapsulates both enthalpy and entropy contributions, making it essential for a holistic view of reaction feasibility. Its clear and straightforward interpretation makes it a valuable tool in this article. The unique aspect of Gibbs Free Energy lies in its capacity to link energy changes with reaction progress, giving insight into the efficiency of water oxidation reactions.
However, while it is beneficial to gauge the spontaneity of reactions, it does not provide information about the rate of the reaction. Therefore, it is important to integrate Gibbs Free Energy analysis with kinetic studies to get a full picture of water oxidation processes.
Enthalpy and Entropy
Enthalpy (H) and entropy (S) are integral to understanding the thermodynamic landscape of oxidation. Enthalpy measures the heat content of a system, while entropy quantifies the level of disorder or randomness. In oxidation reactions, changes in enthalpy reflect energy shifts associated with bond breaking and formation, whereas entropy changes indicate how molecular arrangements evolve throughout the process.
The relationship between these two quantities is encapsulated in the Gibbs Free Energy equation:
[ G = H - TS ]
Here, T represents the absolute temperature. This relationship underscores why these thermodynamic aspects are popular choices when examining water oxidation. Enthalpy is often scrutinized for its differences in initial and final states of the chemical system. On the other hand, entropy allows us to account for disorder changes that tend to drive spontaneous reactions.
However, a drawback in using enthalpy and entropy independently is their interdependence, which can complicate the interpretation of changes in oxidation states. Without a careful approach, one might overemphasize one over the other, potentially leading to misunderstandings about the reaction behaviors.
Role of pH
The role of pH cannot be overstated when discussing water oxidation. In biochemical systems, the acidity or basicity of the environment can drastically affect the oxidation reactions. For instance, under acidic conditions, protons are more abundant, which can facilitate electron transfer mechanisms. Conversely, under alkaline conditions, the availability of hydroxide ions can also play a pivotal role in stimulating or inhibiting reactions.
Moreover, certain enzymes involved in biological water oxidation are sensitive to pH levels. For example, photosystem II, part of the photosynthesis process in plants, operates optimally at a specific pH range. Understanding how pH influences these biological systems is essential for grasping the larger implications of water oxidation.
Temperature Effects
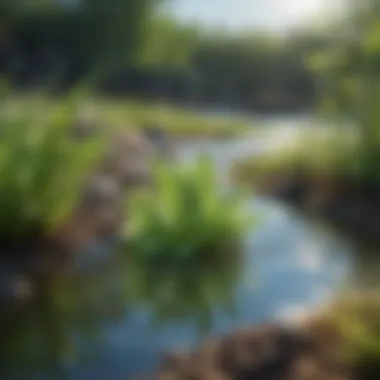
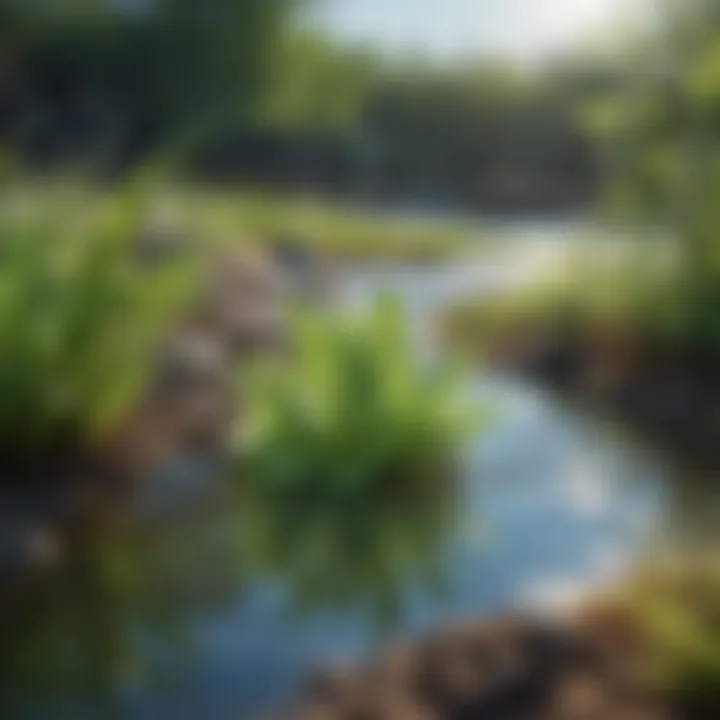
Temperature is another critical player in water oxidation. Generally, as temperature increases, the kinetic energy of molecules also rises, which may lead to a speeding up of oxidation reactions. However, it's not just about faster reactions; higher temperatures can also affect the equilibrium states of the reactions involved.
Thermal effects can cause different species of water to become more reactive, enhancing the overall rate of oxidation. But temperature can also destabilize certain catalysts and intermediates, which could negate the benefits of increased kinetic energy. Thus, any analysis of oxidation should consider the temperature as a dual-edged sword that can optimize or hinder the process.
Catalysts and Inhibitors
Catalysts can dramatically change the landscape of water oxidation. These substances lower the activation energy of reactions, increasing the rate at which oxidation occurs without being consumed in the reaction itself. In industrial applications, the use of specific catalysts can make water oxidation processes more efficient, leading to cost savings and reduced environmental impact.
On the flip side, inhibitors can slow down or prevent oxidation processes. Understanding which substances act as inhibitors is just as vital as knowing the catalysts. Some compounds may introduce competitiveness in binding to active sites, thus hindering the overall reaction rate. This dynamic between catalysts and inhibitors creates a complex ecosystem that is critical for managing reactions that involve water oxidation.
"The intricate balance between catalysts and inhibitors exemplifies that not only the presence of a substance matters but also its nature and concentration."
So, a careful examination of these factors gives insight into the mechanisms driving the oxidation of water, which has substantial implications in biological, environmental, and industrial fields.
Biological Relevance
Understanding the biological relevance of water oxidation sheds light on a fundamental aspect of life on Earth. Water oxidation is not just a chemical transformation; it's a pivotal process tied to energy conversion and biological sustainability. In essence, it forms the backbone of how energy flows through ecosystems and supports diverse life forms. It’s akin to the pulse of living systems, connecting everything from microscopic cells to expansive biomes.
Photosynthetic Water Oxidation
In the realm of photosynthesis, water oxidation takes on a crucial role. The process occurs in the chloroplasts of plant cells, where sunlight catalyzes the transformation of water into oxygen. When chlorophyll absorbs light, it triggers a series of reactions that lead to the splitting of water molecules. Here’s a closer breakdown of the process:
- Photolysis of Water: Light energy breaks down water into hydrogen ions, electrons, and oxygen gas. The released oxygen, a byproduct, is vital for the survival of aerobic organisms, including humans.
- Energy Transfer: The electrons liberated from water travel through the electron transport chain, producing ATP and NADPH. These compounds serve as energy currency for plants to synthesize glucose, which forms the basis of the food web.
- Ecological Impact: The oxygen generated not only enriches the atmosphere but also supports life in aquatic systems. The surplus amounts contribute to maintaining balanced ecosystems, underscoring the significance of water oxidation.
The interplay between water oxidation and photosynthesis illustrates a remarkable cycle of energy and matter, critical for life’s continuity.
Role in Cellular Respiration
Interestingly, water oxidation also finds its importance in cellular respiration, particularly as it relates to the metabolism of living organisms. The connection here is equally profound. During cellular respiration, glucose is oxidized to release stored energy, and water is formed as a product. Below are key points about its role:
- Oxidative Phosphorylation: This process takes place in the mitochondria, where the reduced coenzymes from glycolysis and the Krebs cycle donate electrons to the electron transport chain. As these electrons move through the chain, they ultimately reduce oxygen to form water.
- Energy Production: The energy released during these reactions is harnessed to convert ADP into ATP, providing the energy required for various cellular activities.
- Water Balance: In cellular metabolism, the generation of water is essential for maintaining cellular hydration and balance of fluids, which impacts numerous physiological functions.
By examining these processes, it becomes clear that water oxidation cannot be underestimated. It weaves through the very fabric of life, from the energy acquisition in plants to sustaining complex metabolic pathways in animals.
In summary, the oxidation of water is not merely a reaction; it is a fundamental process that underpins the biological machinery of life.
Through photosynthesis and cellular respiration, it serves as a catalyst for energy flow, impacting everything from individual cellular function to global ecological dynamics.
Environmental Implications
Understanding the environmental implications of water oxidation offers insights into its role in sustaining ecological integrity and promoting a balanced ecosystem. The processes underlying this phenomenon influence not just natural habitats but also human activities that rely on water. By examining water oxidation's effects on aquatic life and water quality, we can grasp the broader ramifications of this critical chemical reaction.
Impact on Aquatic Ecosystems
Water oxidation is pivotal in shaping the health of aquatic ecosystems. The oxidation process helps regulate the amounts of dissolved oxygen, which is essential for fish and other aquatic organisms. Without adequate oxygen levels, these ecosystems face severe challenges; fish and invertebrates struggle to thrive in hypoxic conditions.
Moreover, the oxidation of organic matter contributes to the development of microbial life that governs nutrient cycling within water bodies. Through these interactions, a dynamic equilibrium is maintained, supporting a plethora of biodiversity. For instance, the breakdown of pollutants via oxidation leads to a healthier habitat, ultimately benefiting both flora and fauna.
Its relevance stretches beyond mere biology. A well-oxidized aquatic system can become a buffer against unwanted algal blooms, commonly triggered by excessive nutrients. By fostering oxidation, we reduce the risk of water bodies turning into green mats of algae that choke out light and kill marine life.
Water Quality Concerns
Water quality is an essential factor tied to water oxidation. Considerable research highlights how oxidation reactions can mitigate the effects of contaminants, ensuring safe water for consumption and recreation. Two significant aspects come into play under this section: pollutants and natural purification processes.
Pollutants and Oxidation
The interplay between pollutants and oxidation processes is critical. Pollutants in water, ranging from industrial discharges to agricultural runoff, present considerable risks to ecosystems and human health. Water oxidation acts as a remedy to this chaos.
- Key Characteristic: One defining feature of oxidation of pollutants is its ability to break down complex chemical structures into simpler, less harmful compounds. This transformation drastically reduces toxicity.
- Beneficial Choice: Integrating oxidation processes into water treatment presents a popular strategy for municipalities aiming to meet regulations and improve public health.
- Unique Feature & Advantages/Disadvantages:
- On one hand, oxidation efficiently reduces key contaminants like hydrocarbons and heavy metals.
- On the other hand, it can produce intermediate by-products that may pose their challenges, necessitating comprehensive treatment planning.
Natural Water Purification
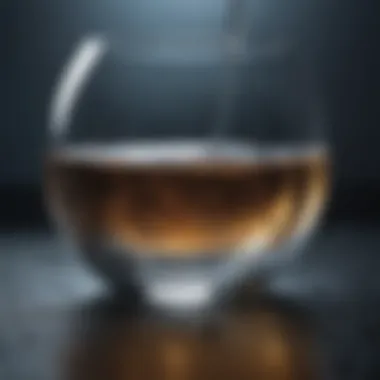
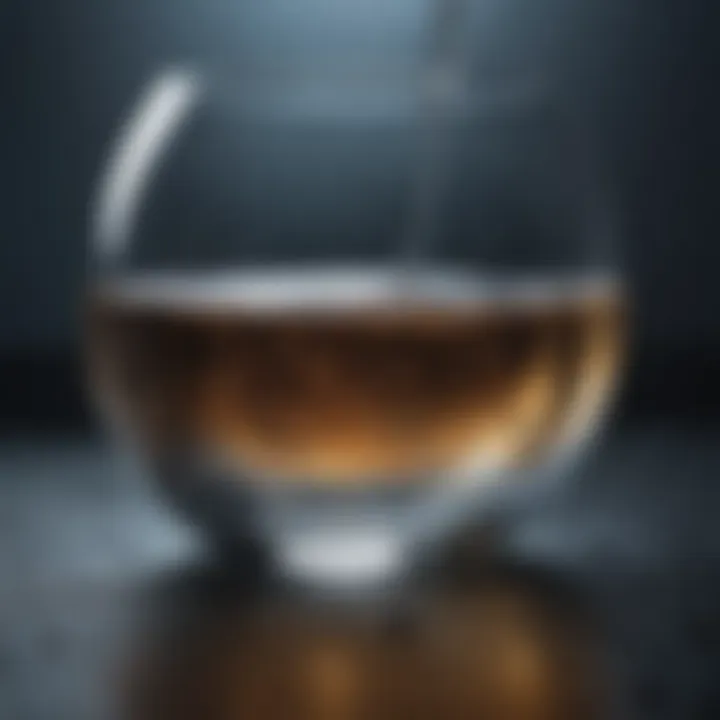
Natural water purification through oxidation processes is often an underestimated mechanism. Water bodies have evolved systems that employ bacteria and various microorganisms to degrade pollutants through oxidation.
- Key Characteristic: This process hinges on natural elements and biological activity, offering a sustainable way to maintain water quality without significant human intervention.
- Beneficial Choice: This method is favored because it often operates cost-effectively and aligns well with ecosystems’ natural rhythms.
- Unique Feature & Advantages/Disadvantages:
- A profound advantage of natural purification is its sustainability; it fosters resilience within ecosystems.
- However, its efficiency can be hampered by human-induced factors, such as chemical imbalances, which limit the natural processes. Thus, it’s a balancing act.
In summation, the environmental implications of water oxidation stretch far and wide, influencing aquatic ecosystems and the quality of water. By advancing our understanding of these processes, we can strive toward better stewardship of our water resources. Such efforts are crucial for both ecological and human well-being.
Industrial Applications
The significance of industrial applications in the context of water oxidation cannot be overstated. Water acts as a universal solvent and medium for many chemical reactions indispensable to various industries. In particular, understanding the mechanisms of water oxidation opens doors to innovative processing techniques, enhancing both environmental sustainability and efficiency in manufacturing. With industries constantly searching for cost-effective and environmentally friendly solutions, water oxidation presents itself as a key player in a multitude of processes.
Chemical Reactions in Industry
Water oxidation plays a crucial role in a myriad of chemical reactions within industrial settings. From the production of hydrogen gas through electrolysis to the synthesis of valuable chemicals, water acts as both a reactant and a solvent.
- Electrochemical Processes: In the context of energy production, water oxidation is pivotal for generating hydrogen fuel. By breaking down water molecules into hydrogen and oxygen, industries can tap into a renewable energy source that adheres to a low-carbon agenda.
- Chemical Synthesis: Numerous chemical synthesis processes rely on oxidation reactions with water. For instance, the production of various organic compounds often necessitates an oxidizing agent, and water serves as a key component in these reactions.
- Analytical Chemistry: The use of water in sample preparation and analysis cannot be overlooked. Understanding how water participates in redox chemistry helps refine analytical methods, making them more accurate and efficient.
The flexibility of water oxidation in facilitating essential chemical reactions showcases its vital role in achieving industrial objectives.
Water Treatment Technologies
Industrial applications of water oxidation extend to the realm of water treatment technologies, where oxidizing agents can transform contaminated water into safe consumable resources. This application is crucial as industries grapple with the challenge of wastewater management and environmental sustainability.
- Advanced Oxidation Processes (AOPs): These processes employ water oxidation to break down organic pollutants into less harmful substances. Techniques such as ozonation and hydrogen peroxide-based oxidation have gained traction, with their ability to tackle micro-pollutants effectively.
- Wastewater Treatment: Industries are leveraging water oxidation to enhance wastewater treatment processes, using methods like the Fenton process which utilizes ferrous ions and hydrogen peroxide to produce hydroxyl radicals for oxidation of hazardous compounds.
- Recycling and Reuse: Water treatment technologies employing oxidation allow for the recycling and safe reuse of water in industrial applications. By effectively removing contaminants, industries can minimize their water footprint, aligning with sustainable practices.
This commitment to harnessing water oxidation for treatment technologies not only addresses regulatory concerns but also paves the way for innovative approaches to water management.
Future Perspectives
The exploration of water oxidation is still very much a field alive with possibilities, pushing the boundaries of our scientific comprehension. Researchers and industries alike regard future perspectives as not just a pathway for advancement but as a crucial frame of reference for addressing pressing global challenges. The significance of this topic cannot be overstated, as it shapes our understanding of environmental sustainability and energy efficiency.
Research Trends
New research trends in water oxidation are paving the way for breakthroughs that could impact energy production and environmental conservation. The current trajectory shows a keen interest in the following elements:
- Nanotechnology: Utilizing nanoparticles to enhance the efficiency of catalytic processes in water oxidation. These materials increase the surface area for reactions, leading to more effective results.
- Bio-inspired methods: Emulating natural processes, particularly those seen in photosynthetic organisms. This aspect of research focuses on how we can harness nature's strategies to improve water oxidation efficiency.
- Sustainable energy: Investigating methods of integrating water oxidation into renewable energy systems. As the world pivots toward sustainable solutions, the relation between oxidation processes and solar energy conversion has come to the forefront.
Researchers are not just observing these trends; they are actively trying to transform them into actionable insights. For instance, recent studies have illustrated how hybrid systems—essentially combining biological and synthetic approaches—can significantly enhance the yield from water oxidation reactions.
Technological Innovations
At the crux of future perspectives lies technological innovation. Here are some noteworthy advancements to keep an eye on:
- Electrochemical Cells: Continuous improvements in electrochemical cell design can enhance water oxidation processes. Innovations allow for increased energy efficiency and reduced costs associated with water-splitting reactions, which are vital for producing hydrogen fuel.
- Artificial Photosynthesis: Merging biological elements with technological systems to create devices that mimic photosynthesis. This could lead to breakthroughs in energy conversion efficiencies, where water oxidation plays a pivotal role.
- Smart Sensors: The integration of smart sensor technology is enhancing our ability to monitor reactions in real-time, providing crystal clear data that leads to better control and optimization of the oxidation process.
"The future of water oxidation research is not just limited to understanding the mechanisms but involves applying this knowledge in innovative ways to solve real-world problems."
The future is a complex tapestry woven from various threads—research trends, technological innovations, and practical applications. By prioritizing water oxidation in these areas, we could unlock new pathways that make significant contributions toward a more sustainable future, addressing energy needs and environmental preservation.
End
The conclusion serves as a capstone, tying together the multitude of elements explored throughout this article. By summarizing the multifaceted nature of water oxidation, we recognize not only its fundamental role in chemical processes but also its broader implications across environmental, biological, and industrial spheres. This synthesis is vital, as it provides the audience with a clear understanding of how intertwined these aspects are in the realm of scientific inquiry.
Summary of Findings
In writing this piece, we delved into several key findings that highlight the prominence of water oxidation:
- Chemical Mechanisms: We discussed the oxidation-reduction reactions, providing insight into both direct and mediated electron transfers that underline water's role in various reactions. This framework is essential for grasping the nuances of how energy moves within chemical systems.
- Influential Factors: Elements such as temperature, pH levels, and the presence of substances that act as catalysts have shown to significantly affect the rate and efficiency of oxidation. These factors were examined to highlight their importance in both natural and engineered contexts.
- Biological Mechanisms: The significance of water oxidation in photosynthesis ignites discussions on ecological balance and energy flow within terrestrial and aquatic ecosystems. Understanding these connections underscores the importance of water oxidation beyond mere chemistry, linking it to life's sustainability.
- Environmental and Industrial Relevance: Water oxidation’s pivotal role in ecological health and its application in water treatment technologies frame its relevance in addressing environmental challenges. As pollutants are oxidized, the restoration of water quality becomes an obtainable goal, adding a layer of urgency to our understanding of these reactions.
In essence, the findings suggest that water oxidation represents a vital intersection of chemistry, biology, and environmental science, making the comprehension of its mechanisms all the more pertinent for researchers, students, and industry professionals alike.
Final Thoughts
Reflecting on the article as a whole, it becomes vivid that the oxidation of water encompasses far more than laboratory reactions. It is an intricate dance of electrons, vital not only for sustaining life through photosynthesis but also for maintaining ecological harmony. As we uncover pathways for technological innovations and research advancements, the importance of this topic comes into sharper focus.
Future studies may well build upon the foundations laid here, exploring uncharted territories in catalysis or finding new applications in sustainable practices. The depth of our understanding can shape not just scientific discourse but actionable strategies toward an environmentally resilient future.
Ultimately, comprehending water oxidation invites us to reassess the relationship between humanity and the natural world—paving the way for smarter, more integrated solutions in tackling the pressing challenges of our time.