Nanoparticle Fabrication Techniques and Applications
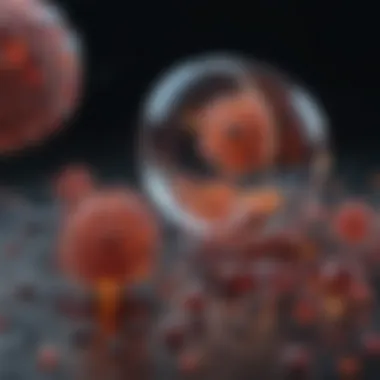
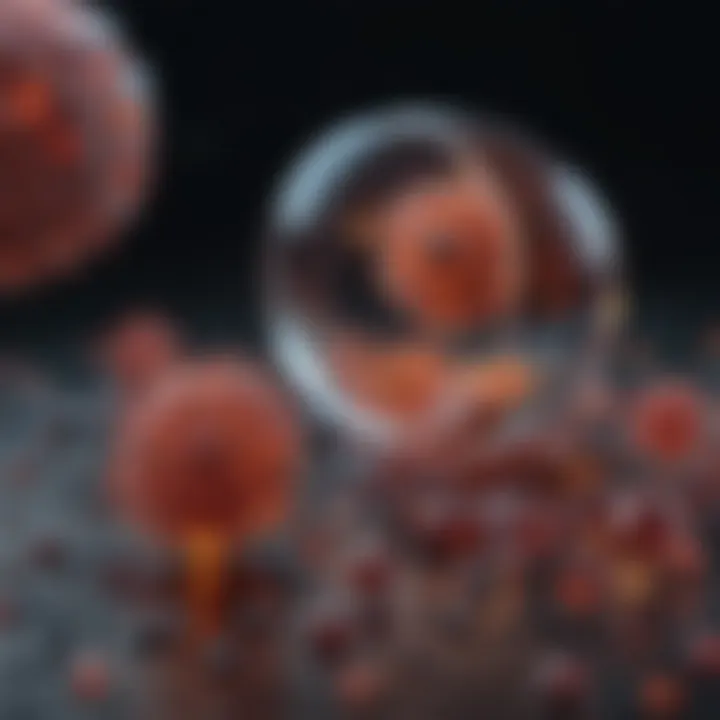
Intro
Nanoparticles have gained prominence in recent years due to their unique properties and applications. These tiny particles, typically in the size range of 1 to 100 nanometers, exhibit different physical and chemical behaviors compared to their bulk counterparts. Their size allows for enhanced reactivity and increased surface area, which can lead to market innovations across various industries, particularly in medicine, electronics, and materials science. Understanding how nanoparticles are fabricated is crucial to harnessing their potential. This article explores the sophisticated methodologies involved in nanoparticle fabrication, shedding light on both top-down and bottom-up approaches.
Methodologies
Description of Research Techniques
Nanoparticle fabrication techniques can primarily be categorized into two overarching strategies: top-down and bottom-up approaches. The top-down process involves breaking down bulk materials into nanoparticles. This can be achieved through methods like milling, lithography, and etching. These methods allow for precise control over the particle shape and size but may also introduce imperfections and defects.
Conversely, the bottom-up approach focuses on constructing nanoparticles from atomic or molecular builds. This includes chemical vapor deposition and sol-gel processes. The bottom-up methods generally yield particles with fewer defects and allow for the design of novel materials with tailored properties. However, they can be more complex and resource-intensive.
Tools and Technologies Used
The tools and technologies employed in nanoparticle fabrication are varied and sophisticated. Some critical tools include:
- Scanning Electron Microscope (SEM): This allows for high-resolution images, aiding in the characterization of nanoparticle morphology.
- Transmission Electron Microscope (TEM): TEM offers insights into the internal structure of nanoparticles, providing information on size and crystallinity.
- Dynamic Light Scattering (DLS): This technique is used to measure the size distribution of nanoparticles in suspension.
Research continues to evolve, leading to the development of innovative tools that improve both the fabrication process and characterization of nanoparticles.
Discussion
Comparison with Previous Research
Research in nanoparticle fabrication is extensive. Traditionally, the focus was primarily on one-dimensional and two-dimensional materials. Recent advancements highlight the shift toward three-dimensional structures leveraging advanced methodologies. Comparing current techniques with historical approaches illustrates the rapid evolution in this field, showcasing improvements in efficiency and effectiveness.
Theoretical Implications
The understanding of nanoparticle synthesis holds significant theoretical implications. As researchers delve deeper into the material properties at the nanoscale, insights gained could influence various scientific disciplines, fostering a multi-faceted approach to problem-solving. By elucidating the underlying principles governing nanoparticle behavior, the scientific community can innovate further, paving the way for groundbreaking applications across many sectors.
"The future of nanotechnology holds promise, as researchers unlock new methodologies for fabricating nanoparticles and understanding their controlled behaviors."
Prelude to Nanoparticles
Nanoparticles have emerged as pivotal components within the realm of modern science and technology. Their unique characteristics, which differ significantly from bulk materials, are a result of their small size, typically ranging from 1 to 100 nanometers. This small scale offers a vast surface area to volume ratio, leading to enhanced reactivity and altered physical and chemical properties. Improved understanding of these properties enhances their utilization in various fields, making them a subject of extensive research.
Understanding nanoparticles is foundational to grasping their vast implications across disciplines. From medicine to electronics, the integration of nanoparticles can drive innovation and efficiency. This section provides two key aspects:
- Definition of Nanoparticles: To grasp what nanoparticles are, it is essential to delve into their specific attributes and classifications.
- Importance in Modern Science: This aspect underscores not just their functional roles, but also the way they are shaping future technologies.
Each element we will discuss in this article will serve to elucidate the intricate processes involved in nanoparticle fabrication. Through studying their definition and significance, we lay a groundwork for exploring fabrication techniques, characterization methods, and diverse applications that highlight their versatility and impact in contemporary scientific dialogues.
Fabrication Techniques
Fabrication techniques are fundamental in the field of nanoparticle research. These techniques determine how particles are created and manipulated, influencing the physical and chemical properties of nanoparticles. Understanding these methods helps in tailoring the nanoparticles for specific applications across various domains, such as medicine and electronics. Both top-down and bottom-up approaches offer unique benefits and considerations, impacting scalability, cost, and material properties.
Top-Down Approaches
Top-down approaches involve breaking down bulk materials to create nanoparticles. One common method is mechanical milling, where solids are subjected to high-energy impacts, leading to particle size reduction. This approach can produce a wide size range of nanoparticles, but it often results in irregular shapes and may introduce defects.
Another technique is lithography, which employs light or electron beams to pattern materials. This method allows for precise control over the size and arrangement of nanoparticles, making it ideal for applications in electronics. However, lithography can be cost-prohibitive due to complex equipment and processes.
Lastly, plasma etching is used to remove layers from a material using plasma. It is a technique that is highly controllable and suitable for creating nanoscale features. Despite its effectiveness, the process can be slow and may require specialized facilities.
Bottom-Up Approaches
In contrast, bottom-up approaches build nanoparticles from smaller units, atoms, or molecules. A prevalent technique is Chemical Vapor Deposition (CVD), which allows for the growth of high-purity films and nanoparticles through chemical reactions in gas phase. This method yields nanoparticles with uniform size and shape but may require expensive precursors and equipment.
Sol-gel techniques are another method, where a solution transforms into a gel-like material. This process is often used to produce metal oxides and can be advantageous for its simplicity and adaptability. However, achieving uniformity in particle size can be challenging.
Additionally, biological synthesis of nanoparticles utilizes living organisms. For example, plants or microorganisms can produce nanoparticles with specific shapes and sizes. This eco-friendly method is notable for its low toxicity and sustainability, although scalability can be an obstacle.
"The choice of fabrication technique plays a crucial role in determining the properties and applications of nanoparticles. Understanding these methods is essential for advancing research and practical applications."
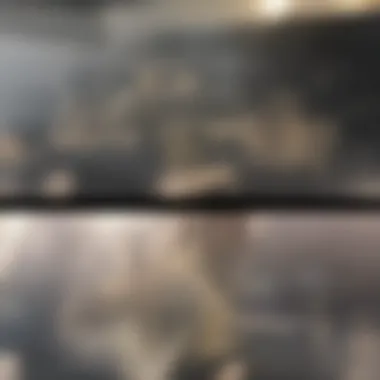
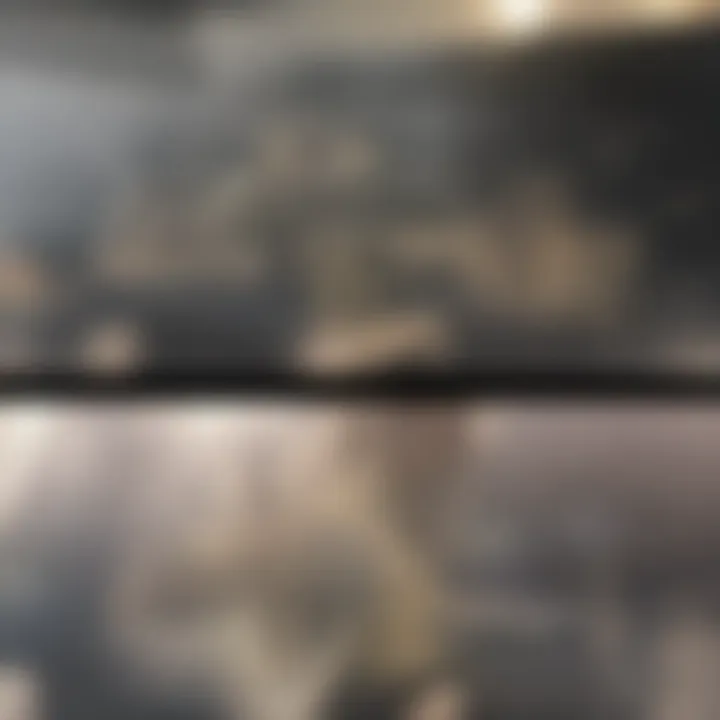
In summary, both top-down and bottom-up approaches present distinct advantages and challenges. A comprehensive grasp of these fabrication techniques enables researchers to optimize nanoparticle development for diverse applications.
Top-Down Approaches Explained
Top-down approaches play a crucial role in nanoparticle fabrication. They involve the reduction of bulk materials into nanoscale particles. This method is advantageous because it can utilize existing materials, making it resource-efficient. By understanding the various techniques within this category, researchers can explore the potential of nanoparticles utilized across different fields.
Mechanical Milling
Mechanical milling is a common technique used to create nanoparticles. This method involves the grinding of bulk materials to achieve nanoscale size. The process can lead to significant refinement of particle size. With the help of a ball mill, for example, hard materials are crushed repetitively until they reach desired dimensions. An advantage of mechanical milling is that it allows for a wide range of materials to be processed, including metals, ceramics, and polymers. However, a notable consideration is that this process often leads to the generation of unwanted heat. Excessive heat can alter the properties of nanoparticles, potentially affecting their performance.
Lithography Techniques
Lithography encompasses a series of processes used to pattern materials at the nanoscale. In nanoparticle fabrication, this technique is vital for precision. Photolithography relies on light to transfer a geometric pattern onto a substrate. This method is effective for creating uniformly sized and positioned nanoparticles. On the other hand, electron beam lithography uses focused beams of electrons to draw custom shapes. These lithography techniques allow scientists to control the particle size and distribution effectively.
*** "Lithography provides unprecedented control over the fabrication process. This precision is essential for applications requiring exact specifications." ***
Nonetheless, lithographic techniques present challenges as well. They can be expensive and time-consuming compared to other fabrication methods. Additionally, aligning patterns on a substrate can be complex, necessitating careful calibration.
Plasma Etching
Plasma etching is another significant top-down technique used in nanoparticle fabrication. This method employs plasma to remove material from the surface of a substrate, creating nanostructures. It is particularly effective for achieving high precision and sharp edges on nanoparticles. Plasma etching involves ions which bombard the surface, effectively sculpting the material. The primary benefit of this technique is that it can produce intricate designs that are difficult to achieve through other methods.
In addition, it allows for the control of etching depth and rates, which is essential for optimizing particle characteristics. However, challenges include potential damage to the underlying substrate from the aggressive nature of plasma bombardment.
In summary, top-down approaches offer several techniques for creating nanoparticles with specific properties. Understanding these methods is beneficial for researchers aiming to optimize the fabrication of nanoparticles, ensuring they meet the requirements of various applications.
Bottom-Up Approaches Explained
Bottom-up approaches to nanoparticle fabrication are significant because they allow the construction of materials from the atomic or molecular level upward. This strategy offers a unique way to create structures with controlled properties, leading to enhanced functionality in various applications. One of the key benefits of bottom-up methods is their ability to produce nanoparticles with uniform sizes and shapes, which are critical factors in areas such as catalysis, drug delivery, and electronics. However, it is crucial to acknowledge the considerations involved, including time and cost efficiency, environmental impact, and scalability.
Chemical Vapor Deposition
Chemical Vapor Deposition (CVD) is a widely used technique in the production of nanoparticles. In this method, precursors are vaporized and transported to a substrate, where they react to form a solid material. This process allows for precise control over composition and thickness, making CVD ideal for applications like semiconductor manufacturing and coatings.
Key advantages of CVD include:
- High purity of materials
- Ability to produce conformal coatings on complex geometries
- Scalability for industrial applications
However, challenges exist in the form of precursor stability and waste management. Optimizing these factors is essential for advancing the CVD process in practical settings.
Sol-Gel Techniques
Sol-gel techniques represent another effective bottom-up method for fabricating nanoparticles. This process begins with the creation of a colloidal solution (or sol) that transitions into a gel phase as the nanoparticles form. Sol-gel techniques are known for their versatility and can be used to create diverse materials, such as oxides and ceramics.
Some notable points about sol-gel processes include:
- Simple processing at relatively low temperatures
- Capability to incorporate various dopants for tailored properties
- Potential for coating applications on different substrates
The drawbacks often highlight challenges regarding homogeneity and the need for efficient drying processes to avoid crack formation in the final product.
Biological Synthesis of Nanoparticles
Biological synthesis of nanoparticles involves the use of biological organisms to produce nanomaterials. This method, often referred to as green synthesis, leverages plants, bacteria, or fungi to generate nanoparticles in an eco-friendly manner. The biological approach is gaining popularity due to its sustainability, reduced toxicity, and potential for large-scale production.
Benefits of this method include:
- Lower production costs
- Minimal environmental impact
- Intrinsic functional capabilities imparted by biological agents
Despite these advantages, challenges in controlling the size and shape of the nanoparticles remain. Future research will likely focus on optimizing these variables to harness the full potential of biological synthesis.
"The exploration of bottom-up approaches not only advances the capabilities of nanoparticle fabrication but also aligns with sustainable practices in materials science."
In summary, bottom-up approaches in nanoparticle fabrication offer profound possibilities for innovation across many fields. As these techniques evolve, they hold the potential to reshape how materials are created and utilized, highlighting their significance in both research and applied sciences.
Nanoparticle Characterization Techniques
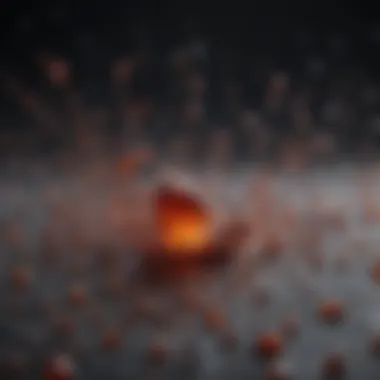
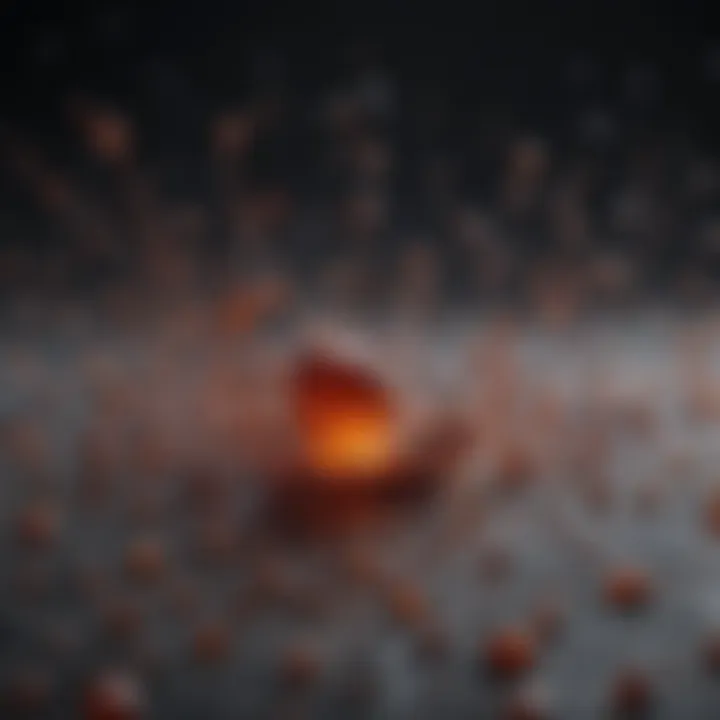
Nanoparticle characterization techniques are essential in understanding the properties and behaviors of nanoparticles. This section delves into different methods that scientists use to determine the size, shape, distribution, and structural characteristics of nanoparticles. Accurate characterization is crucial for both applying nanoparticles effectively in various fields and ensuring the reproducibility of synthesis methods. Key benefits of these techniques include providing insights for optimizing synthesis processes and confirming the suitability of nanoparticles for specific applications.
Electron Microscopy
Electron microscopy is a powerful tool that allows for high-resolution imaging of nanoparticles. This technique uses a beam of electrons instead of light, enabling visualization at the atomic level. It is especially valuable for determining the morphology and size of nanoparticles.
One common type is Transmission Electron Microscopy (TEM), which can resolve details below 1 nanometer. Scanning Electron Microscopy (SEM) is another variant, providing surface texture information. Both techniques are critical in confirming the dimensions of nanoparticles and their aggregates.
Dynamic Light Scattering
Dynamic Light Scattering (DLS) is a method used to measure the size distribution of small particles suspended in a fluid. When a laser beam passes through a sample, the light scatters off the nanoparticles. The Doppler shift that occurs provides information about the size based on how fast the particles are moving due to Brownian motion.
DLS is advantageous due to its ease of use and speed, allowing for rapid characterization. However, it may not accurately measure polydisperse samples, as it averages data from all particle sizes present.
X-ray Diffraction
X-ray diffraction (XRD) is used to examine the crystallographic structure of nanoparticles. When X-rays are directed at a crystalline sample, they are diffracted at specific angles. By measuring these angles and the intensity of scattered rays, scientists can determine the crystal structure, phase composition, and size of the nanoparticles.
XRD is vital for ensuring that the synthesized nanoparticles maintain the desired crystallinity, which often influences their reactivity and mechanical properties.
Spectroscopic Methods
Spectroscopic methods encompass a range of techniques that analyze the interaction between nanoparticles and electromagnetic radiation. These methods provide information about the chemical composition and electronic structure of nanoparticles.
Common techniques include UV-Vis spectroscopy, which monitors absorption spectra, and Fourier Transform Infrared spectroscopy (FTIR), which helps identify chemical bonds. These methods are crucial for understanding the functional properties of nanoparticles, particularly when designing them for specific applications.
"Characterization techniques not only reveal nanoparticle properties but also guide future research direction through empirical data."
Effective characterization is a multi-faceted process that requires the integration of various methods. Through these techniques, researchers can ensure that the right properties are achieved for successful applications in fields like medicine, electronics, and environmental science.
Applications of Nanoparticles
Nanoparticles represent a paradigm shift in numerous scientific fields. Their unique properties, stemming from their small size, enable new innovations and solutions across varied applications. Understanding the applications of nanoparticles is essential, as it highlights their relevance in advancing technology and improving human lives.
In Medicine
Nanoparticles play a crucial role in medicine, particularly in drug delivery and diagnostics. The small size allows them to navigate biological barriers, enhancing the effectiveness of treatments. Here are some key points to consider:
- Targeted Drug Delivery: Nanoparticles can deliver drugs directly to specific cells, reducing side effects and maximizing therapeutic effects. This is particularly beneficial in cancer therapy, where targeted nanoparticles can attack tumor cells while leaving healthy cells untouched.
- Imaging and Diagnostics: Certain nanoparticles are used as contrast agents in imaging techniques such as MRI and CT scans. They improve the visibility of tissues and organs, enabling early detection of diseases.
- Theranostics: This combines therapy and diagnostics. Nanoparticles can be designed to deliver therapeutic agents while also allowing monitoring of the treatment progress through imaging.
"The integration of nanoparticles in medicine signifies a bold step forward, redefining treatment methodologies and improving patient outcomes."
In Electronics
The electronics industry benefits significantly from nanoparticle applications. Their unique electrical, optical, and magnetic properties are exploited in various technologies. Consider the following:
- Conductivity Enhancements: Nanoparticles like silver and copper are used to create better conductive materials. These enhance the efficiency of electronic components, improving overall performance.
- Transparent Conductors: Indium tin oxide nanoparticles serve as transparent conductors in touch screens and displays, allowing device functionality without sacrificing visibility.
- Memory Storage: Nanoparticles are being researched for use in high-density memory storage devices. Their small size can lead to more compact storage solutions, addressing the growing demand for data retention.
In Environmental Science
Environmental science is another field where nanoparticles have transformative potential. They aid in addressing ecological challenges through innovative solutions:
- Pollution Remediation: Nanoparticles can degrade pollutants in water and soil. They target contaminants at a molecular level, which can lead to more effective cleanup efforts.
- Sensors for Monitoring: Nanosensors detect environmental pollutants with high sensitivity, enabling timely responses to contamination issues.
- Sustainable Energy Solutions: In solar cells, nanoparticles improve light absorption and energy conversion efficiency. This can lead to more sustainable energy sources.
Overall, the applications of nanoparticles are varied and impactful. Their integration into countless sectors not only enhances functionality but also promotes innovative approaches to pressing global challenges. Each application showcases the potential of nanotechnology to revolutionize our interaction with the world.
Advantages of Nanoparticles
Nanoparticles offer remarkable benefits that contribute significantly to various fields of science and technology. The unique properties of nanoparticles arise from their small size and high surface-area-to-volume ratio. This section discusses two key advantages: enhanced properties and versatility in applications.
Enhanced Properties
Nanoparticles exhibit enhanced properties that often deviate from their bulk counterparts. These alterations in physical, chemical, and biological properties derive from the sheer scale at which nanoparticles operate. For instance, gold nanoparticles show different optical characteristics compared to bulk gold. Their color and light absorption change due to quantum effects. Moreover, the larger surface area allows for a higher reactivity, making them suitable for catalytic applications.
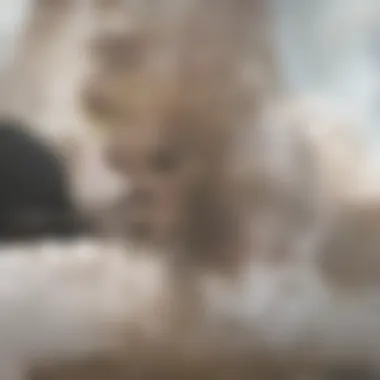
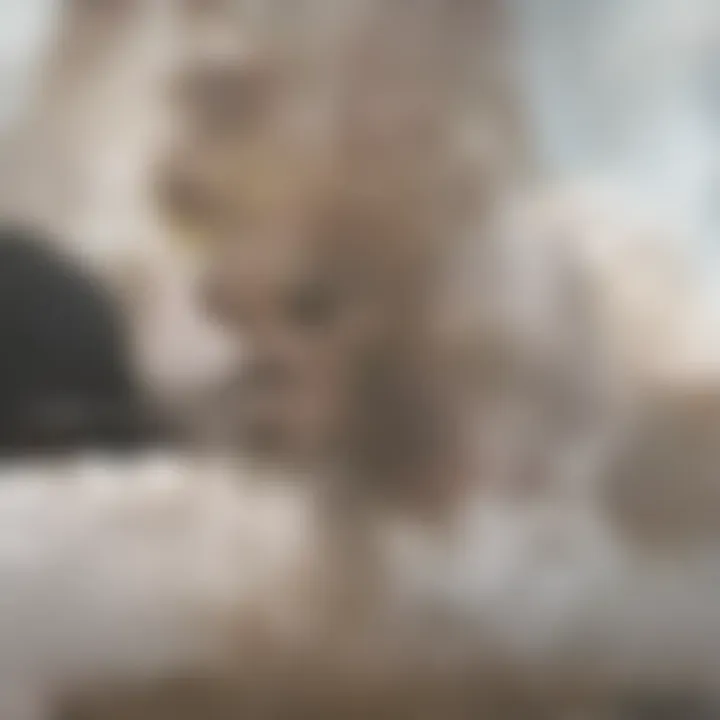
Other enhanced characteristics include:
- Strength: Nanoparticles can increase the tensile strength of materials, improving durability.
- Electrical Conductivity: Some nanoparticles enhance conductivity, valuable in electronic devices.
- Thermal Stability: Nanoparticles can improve heat resistance in various applications.
- Magnetic Properties: Magnetic nanoparticles find use in data storage and biomedical applications.
In medicine, for example, iron oxide nanoparticles possess superparamagnetic properties that are exploited in magnetic resonance imaging and targeted drug delivery methods. Through such enhancement of properties, nanoparticles play a crucial role in advancing technology and improving efficiency in numerous applications.
Versatility in Applications
Another key advantage of nanoparticles is their versatility in applications. Due to their unique properties, nanoparticles can be utilized across a wide range of fields. The following applications highlight their flexibility:
- Medicine: They are used in drug delivery systems, imaging, and therapeutic agents. Nanoparticles can target specific cells, minimizing drug side effects.
- Electronics: In this sector, nanoparticles enhance the performance of devices such as thin-film transistors and solar cells. They increase efficiency while reducing material costs.
- Environmental Science: Nanoparticles serve in water treatment processes. They can effectively remove contaminants and pollutants.
- Energy Storage: Nanotechnology improves battery technology, leading to more efficient energy storage solutions.
"The flexibility in applying nanoparticles to various problems demonstrates their growing significance in scientific innovation and practical applications."
The ability to engineer nanoparticles for specific purposes results in creative solutions for complex issues. As research continues, the versatility of nanoparticles will likely expand, leading to unexpected breakthroughs in science and technology.
Challenges in Nanoparticle Production
The journey of nanoparticle production is not without its hurdles. While advancements in fabrication techniques have driven innovation, challenges persist that can impact the efficacy and applicability of nanoparticles. Understanding these challenges is crucial for researchers and practitioners alike, particularly when considering scalability, quality, and standardization in this evolving field.
Scale-Up Issues
Transitioning from the laboratory to industrial-scale production of nanoparticles poses unique challenges. In a controlled research environment, conditions can be finely tuned, allowing for precise synthesis. However, when scaling up, maintaining uniformity and quality becomes difficult. Factors such as reactor design, temperature fluctuations, and material costs all contribute to inconsistencies.
In essence, if the nanoparticle production process is not properly optimized for larger scales, issues may arise. This can include variations in particle morphology, size distribution, and surface characteristics. Accordingly, companies or labs attempting to manufacture nanoparticles in bulk may find profitability compromised by excessive waste and rework required due to inconsistent quality.
Contamination and Standardization
Contamination during the production process can adversely affect the properties of nanoparticles. In environments where nanoparticles are synthesized, even minor contaminants can lead to significant changes in behavior and efficacy. Such contamination can stem from equipment, operator error, or even the materials themselves.
Furthermore, the lack of standardized procedures creates obstacles in the comparison of results across different studies. Without a consensus on best practices for nanoparticle fabrication, reproducibility diminishes, which is vital for advanced research and application in various fields such as medicine and electronics. Standardization efforts encompass establishing criteria for particle size, morphology, and purity, which can streamline research and development efforts.
"Standardized fabrication techniques ensure that the research community can build on previous work effectively."
Future Directions in Nanoparticle Research
The field of nanoparticle research is dynamic, constantly evolving with new technological advances and discoveries. Future directions in this area are crucial for not only improving fabrication processes but also for expanding the applications of these materials. Researchers are keen on addressing existing limitations in nanoparticle production and finding innovative solutions that enhance functionality. This section explores innovations in fabrication techniques and important regulatory considerations that will shape the future of nanoparticle applications.
Innovations in Fabrication Techniques
Recent innovations in fabrication techniques are paving the way for more efficient and scalable production of nanoparticles. These techniques seek to improve the properties and application potential of nanoparticles across various fields. Some notable approaches include:
- 3D Printing: This method enables precise control over the shape and size of nanoparticles. It offers opportunities for product customization, which can be crucial in medical applications.
- Electrospraying: This technique allows for the generation of nanoparticles with tunable sizes by applying an electrical potential in a fluid solution.
- Microwave-assisted synthesis: By using microwaves for heating, this method can significantly reduce synthesis time while improving particle uniformity.
- Green synthesis methods: Innovations in using biological organisms for nanoparticle production are garnering attention. Using microorganisms or plant extracts reduces environmental impact compared to traditional chemical methods.
These advancements are not just about increasing the efficiency of production; they also focus on tailoring the surface properties of nanoparticles to enhance their performance in specific applications, such as drug delivery or sensor technologies.
Regulatory Considerations
As the field of nanoparticle research advances, regulatory considerations become increasingly important. The unique properties of nanoparticles pose challenges not typically faced with bulk materials. Ensuring safety and efficacy in various applications is essential. Key regulatory considerations include:
- Safety Assessments: Agencies like the U.S. Environmental Protection Agency require comprehensive safety data assessing the impact of nanoparticles on human health and the environment.
- Standardization: Developing clear standards for nanoparticle characterization and testing is crucial. This will help unify research approaches and ensure consistency in results.
- Intellectual Property Rights: Researchers must navigate complex patent landscapes when developing new nanoparticles or fabrication methods. Understanding the implications of intellectual property laws is vital for innovation.
In summary, addressing regulatory challenges will facilitate the commercialization of nanoparticle technologies while ensuring safety and compliance with legal standards.
The future of nanoparticle research hinges on integrating innovative fabrication techniques with robust regulatory frameworks. By focusing on these areas, researchers will enhance nanoparticle utility in medicine, electronics, and environmental applications, driving forward the potential these materials hold.
Ending
In this article, we have explored the significant dimensions of nanoparticle fabrication, emphasizing its multifaceted nature in research and application. The discussion highlighted the critical techniques categorized into top-down and bottom-up approaches, each with unique benefits and limitations. These methods shape how we innovate in various fields, from medicine to environmental science. Understanding these methods helps stakeholders appreciate the precision and creativity needed to manipulate materials at the nanoscale.
Summary of Key Points
- Nanoparticle Fabrication Methods: Both top-down and bottom-up techniques play pivotal roles. Top-down approaches involve breaking down larger materials, which can introduce flaws, while bottom-up techniques allow building nanoparticles atom by atom, enabling greater control.
- Characterization Techniques: Several methods such as electron microscopy and dynamic light scattering are crucial for evaluating nanoparticle properties. This ensures that the fabricated nanoparticles meet the specifications required for their intended applications.
- Applications: The use of nanoparticles spans different sectors. In medicine, they are used for targeted drug delivery; in electronics, they enhance device performance; and in environmental science, they help in pollution remediation.
- Challenges: Despite advancements, challenges such as scale-up issues and contamination remain. Addressing these is vital for the successful commercialization of nanoparticle technologies.
Future Implications
The future of nanoparticle research lies in refining fabrication techniques and addressing regulatory considerations. Advancements in methods will likely lead to more economical and efficient production processes. As regulations evolve to keep pace with innovations, a proactive approach in compliance will empower researchers and manufacturers. Furthermore, as knowledge about nanoparticles grows, their potential applications will likely expand beyond current boundaries, possibly leading to breakthroughs in sustainable technologies and personalized medicine. Maintaining an interdisciplinary perspective will be essential, as collaborations across scientific fields can yield novel solutions to complex challenges.
"The landscape of nanoparticle research is rapidly evolving, presenting both challenges and vast opportunities for innovation."
In summary, our exploration of nanoparticle fabrication reveals a critical intersection of science and technology, embodying both the promise and complexity inherent in modern material science. By embracing a thorough understanding of these processes, the scientific community is better equipped to unlocking the full potential of nanoparticles in various applications.