Exploring Mouse Bone Marrow Cells: Insights and Innovations
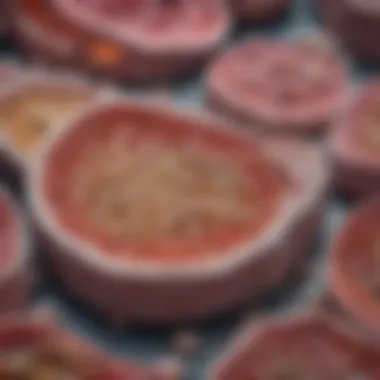
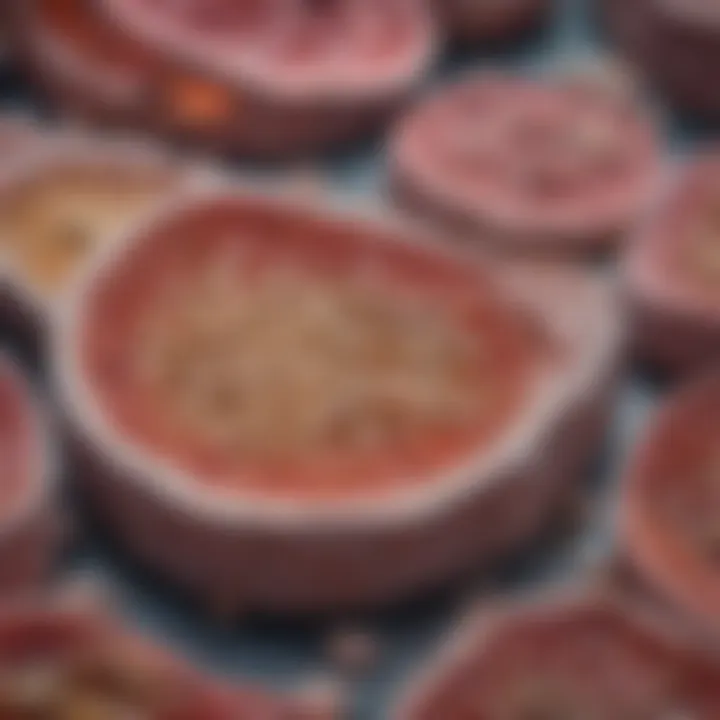
Intro
Mouse bone marrow cells play a pivotal role in the study of hematopoiesis, the process by which blood cells are formed. Understanding the structure and function of these cells is crucial for advancing biomedical research, particularly in areas such as regenerative medicine and immunotherapy. The utility of mouse models offers a unique advantage due to their genetic similarity to humans, which allows researchers to explore human diseases and therapeutic approaches more effectively.
The nuances of different cell types within the bone marrow, their developmental stages, and various isolation techniques contribute to a deeper understanding of their functions. By examining these factors, researchers can identify potential applications in treating blood disorders and enhancing immune responses. This article will delve into these complexities, shedding light on the methodologies and implications for future research.
Methodologies
Description of Research Techniques
Studying mouse bone marrow cells involves an array of sophisticated techniques designed to isolate and characterize various cell types. Common methodologies include flow cytometry, which enables researchers to analyze cell surface markers. This technique allows for differentiation between hematopoietic stem cells and differentiated progenitor cells. Another significant method is magnetic-activated cell sorting (MACS), which utilizes specific antibodies to isolate target cells from heterogeneous populations.
Isolating the bone marrow itself typically follows standardized protocols, which often include the use of collagenase or other enzymes to facilitate the separation of cells. After obtaining a single-cell suspension, researchers can apply multiple assays to assess cell viability, proliferation, and function.
Tools and Technologies Used
Researchers employ a variety of tools and technologies in their studies of mouse bone marrow cells. Essential instruments include:
- Flow Cytometers: For quantifying and analyzing the characteristics of single cells.
- Confocal Microscopes: Allow for detailed imaging of cell features and interactions.
- PCR Machines: Used for amplifying DNA, crucial for genetic studies of isolated cells.
The integration of these technologies enhances the accuracy of research, thereby contributing to more reliable outcomes. In addition, advancements in single-cell RNA sequencing are transforming our understanding of gene expression profiles within these cells, leading to more personalized approaches in treatment strategies.
Discussion
Comparison with Previous Research
Previous studies on mouse bone marrow have laid a foundation for our current understanding. Current research often corroborates findings from earlier works but also identifies new dimensions in cell behavior and interaction. For example, the role of the microenvironment in influencing hematopoietic stem cell function has been extensively explored in the past. Recent discoveries have added more complexity by uncovering novel signal pathways involved in cell maintenance and differentiation.
Theoretical Implications
Examining mouse bone marrow cells has broader implications for theoretical models in hematology and oncology. Insights gained from these studies could lead to refined models of disease progression and treatment responses. Understanding the molecular mechanisms that govern stem cell dynamics offers potential for breakthroughs in targeting therapies, particularly in diseases like leukemia, where bone marrow function is critically altered.
As research evolves, it is critical to continually evaluate and integrate new findings to ensure that methodologies remain relevant and efficacious in addressing complex biological questions.
In summary, ongoing investigations into mouse bone marrow cells are essential for the advancement of medical science. The methodologies and insights derived from these studies not only enhance our understanding but also underscore the significance of these cells in developing innovative therapies.
Preface to Mouse Bone Marrow Cells
Mouse bone marrow cells play a pivotal role in understanding both fundamental biological processes and various diseases. Their significance lies in their involvement in hematopoiesis, the process by which all blood cells are produced. As a model organism, mice share many genetic and physiological traits with humans, making them ideal subjects for research. This relevance allows for translational discoveries that can inform clinical practices and therapies.
Definition and Importance
Bone marrow is a specialized tissue found in the hollow interior of bones. It consists of a diverse population of cells, mainly hematopoietic and stromal cells. Hematopoietic cells generate the different blood cell types, including red blood cells, white blood cells, and platelets. Stromal cells provide structural support and play roles in cell signaling and maintenance of the hematopoietic environment. This duality in function highlights the complexity and necessity of studying bone marrow cells. Their importance extends beyond mere cellular functions, touching on regenerative medicine and the development of immunotherapeutic strategies.
Understanding the structure and function of these cells has profound implications for health. For instance, insights gained from mouse models have led to advancements in treatments for cancers and blood disorders. Moreover, bone marrow studies contribute significantly to inform research initiatives around aging, autoimmune diseases, and the effects of molecular manipulations on cellular behavior.
Historical Context of Bone Marrow Research
The exploration of bone marrow values its roots in the early discoveries of blood formation. The term hematopoiesis comes from the Greek words 'hema' (blood) and 'poiesis' (to make). Researchers first identified the bone marrow's role in blood production in the 19th century. Since then, numerous breakthroughs have transformed our knowledge.
The development of techniques like bone marrow aspiration and transplantation in the mid-20th century marked significant milestones. With advancements in genetic technologies over the following decades, the ability to manipulate mouse genetic makeup has enhanced understanding of bone marrow cell functions immensely. Today, the complexity of bone marrow is appreciated, with research focusing not only on its cellular composition but also on the intricate regulatory mechanisms governing hematopoiesis and immune responses.
"Bone marrow research has a rich history that has laid the groundwork for modern hematology and therapeutic innovations."
In summary, the investigation of mouse bone marrow cells is an essential endeavor in biomedical research. It opens avenues for understanding both normal biological functions and pathological conditions, fostering progress in health and medicine.
Composition of Mouse Bone Marrow
Understanding the composition of mouse bone marrow is crucial for comprehending its complex roles in health and disease. Bone marrow is not a homogeneous tissue; it consists of various cell types that collaborate to maintain fundamental physiological processes. The main components include hematopoietic cells, responsible for blood cell formation, and stromal cells, which provide support and regulatory functions. This composition influences not only the development and differentiation of blood cells but also the immune response and regenerative capabilities. Thus, a detailed exploration of these components sheds light on their significance in research and clinical practices.
Hematopoietic Cells
Lineage Classification
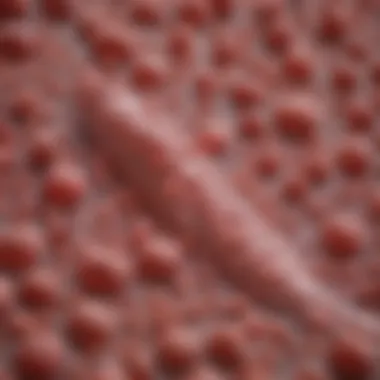
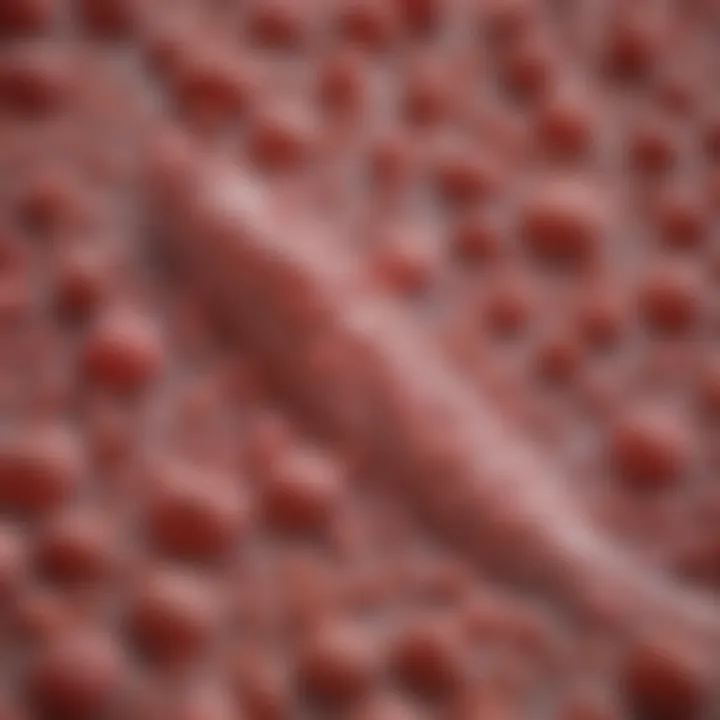
Lineage classification categorizes hematopoietic cells based on their origin and developmental pathways. This classification is essential because it helps scientists track the fate of specific cell types through various stages of maturation. Key characteristics of lineage classification include its systematic approach and ability to categorize cells into distinct lineages such as myeloid and lymphoid. This method is beneficial in research for identifying cellular function and lineage-specific responses. However, one unique feature of lineage classification is its potential to oversimplify the complex nature of cell signaling during hematopoiesis. This limitation should be recognized when interpreting results from lineage studies.
Development Stages
Development stages refer to the sequential phases hematopoietic cells undergo from pluripotent stem cells to differentiated blood cells. Each stage is marked by specific surface markers and functional capabilities. The key characteristic of these stages is their role in maintaining a balance in hematopoiesis, ensuring a steady supply of mature blood cells. This aspect is particularly relevant to this article as it underscores how disruptions in these stages can lead to hematological disorders. A unique feature of development stages is their temporal aspect, altering over time under varying physiological conditions. Hence, understanding these stages is crucial for developing therapies for blood disorders.
Functional Roles
Functional roles of hematopoietic cells highlight their contributions to sustaining hemostasis and immune functions. Different cell types such as erythrocytes, leukocytes, and platelets serve distinct purposes in oxygen transport, pathogen defense, and clot formation, respectively. The key characteristic of functional roles is their specialization, allowing the immune system to respond effectively to pathogenic threats. This specificity makes it a compelling focus in research related to disease modeling and therapy development. However, a unique feature of functional roles is the potential redundancy observed within these roles. This redundancy can complicate the understanding of how specific cells interact during complex immune responses.
Stromal Cells
Adipocytes
Adipocytes are a type of stromal cell that plays a role in energy storage and metabolic regulation within the bone marrow microenvironment. They contribute to homeostasis by regulating fat metabolism and interacting with hematopoietic cells. The key characteristic of adipocytes is their ability to influence the differentiation of hematopoietic stem cells towards various lineages based on energy needs. This aspect makes adipocytes a beneficial focus in the context of obesity-related hematological disorders. A unique feature of adipocytes is their plasticity in response to metabolic changes, which can lead to both advantages and disadvantages depending on the physiological state.
Fibroblasts
Fibroblasts are essential stromal cells that provide structural support within the bone marrow. They secrete extracellular matrix components and cytokines that regulate hematopoiesis. The key characteristic of fibroblasts is their role in creating a niche for hematopoietic stem cells, essential for their survival and function. This makes fibroblasts a popular choice for studying cell interactions in the bone marrow. However, a unique feature is their variability in function, influenced by local signals and microenvironment conditions, which can complicate the understanding of their overall role in hematopoiesis.
Macrophages
Macrophages within the bone marrow serve a dual purpose; they partake in phagocytosis and are crucial for maintaining tissue homeostasis. They can influence hematopoietic cell fate through cytokine production and cellular interactions. The key characteristic of macrophages is their ability to adapt their function based on environmental cues, making them a vital component in both immunity and tissue repair. Their importance in this article arises from their complex interactions with hematopoietic cells and their implications in both hematological disorders and immune responses. A unique feature of macrophages is their plasticity, allowing them to adopt different functional states, which poses challenges in clearly defining their role in different conditions.
Isolation and Characterization Techniques
The isolation and characterization of mouse bone marrow cells are essential for understanding their roles in health and disease. Such techniques enable researchers to obtain pure populations of specific cell types for more detailed analysis. It allows for the study of hematopeietic and stromal components within the bone marrow. Efficient isolation techniques lead to better characterization and understanding of cellular functions, ultimately enhancing research efforts. The accurate characterization of cells is crucial for determining their state of differentiation and functional capabilities.
Bone Marrow Harvesting
Bone marrow harvesting is a vital step in the process of studying these cells. The standard method involves aspiration from the iliac crest or the femur. This procedure is minimally invasive but requires careful handling to ensure sample integrity. The success of this step directly impacts the quality of isolated cells. Researchers often use sterile techniques to avoid contamination.
Moreover, it is important to collect adequate volumes to obtain sufficient cellular material. Proper preparation of the animal prior to harvesting also affects outcomes.
Flow Cytometry Applications
Flow cytometry offers an advanced method for characterizing cells within the bone marrow. It allows for the analysis of cell populations based on size, granularity, and fluorescent marking. This technique can identify specific cell subsets, which is crucial for understanding their roles in hematopoiesis and immunity.
Cell Surface Markers
Cell surface markers are proteins located on the cell membrane that can be targeted for identification. These markers provide essential information about cell lineage and stage of development. They play a crucial role in differentiating between various cell types, highlighting their unique characteristics. For instance, the expression of specific markers can help identify progenitor cells versus mature cells.
The benefit of using cell surface markers lies in their specificity and reproducibility. However, selecting the right markers is crucial, as not all markers are universally applicable.
Data Analysis
Data analysis is another critical component of flow cytometry. It involves interpreting complex data sets obtained from cell sorting and characterization. By employing various software tools, researchers can perform in-depth analysis of cell populations. Good data analysis practices can enhance the reproducibility and reliability of results.
One of the key characteristics of data analysis is its ability to correlate different parameters. This capability provides insights into cell behavior and interactions in the bone marrow microenvironment. However, the complexity of the data may pose challenges, necessitating statistical rigor and careful interpretation.
Gene Expression Profiling
Gene expression profiling is fundamental in understanding the biological processes occurring within isolated bone marrow cells. By analyzing the expression levels of specific genes, researchers can assess the functional states of the cells. This technique helps in identifying pathways that are active during hematopoiesis or immune responses.
Furthermore, profiling contributes to personalized medicine by uncovering molecular signatures associated with various diseases. The technique has advanced significantly, with newer methods allowing for high-throughput analysis of thousands of genes simultaneously. However, the interpretation of gene expression data must be conducted carefully to draw valid conclusions.
Physiological Functions of Bone Marrow Cells
The physiological functions of mouse bone marrow cells are interlinked with critical processes such as hematopoiesis and immune response regulation. Understanding these functions is vital as they provide insights into how these cells maintain homeostasis and respond to challenges such as injury or infection.
Hematopoiesis Mechanisms
Stem Cell Dynamics
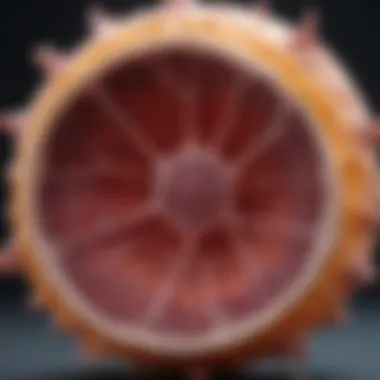
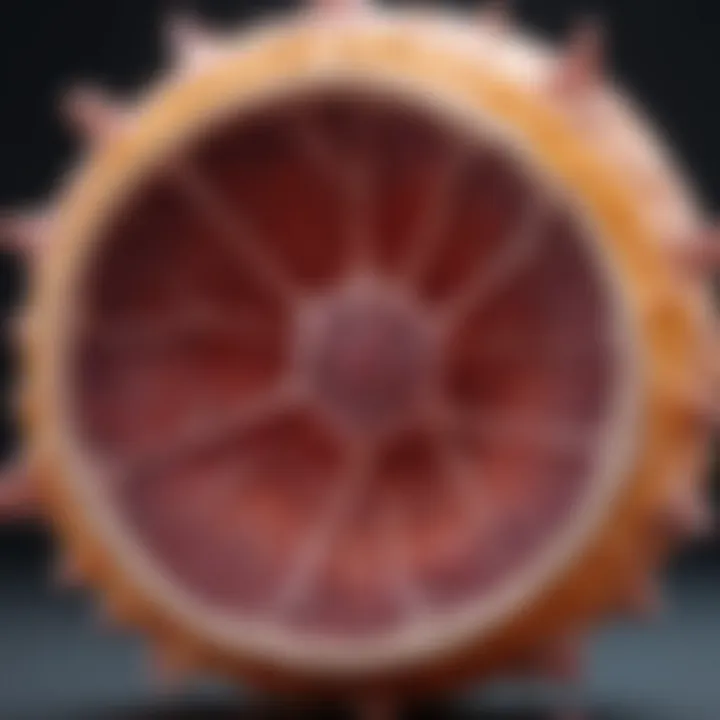
Stem cell dynamics in bone marrow refer to the behaviors and characteristics of hematopoietic stem cells. These cells are responsible for generating various blood cell types, which include red blood cells, white blood cells, and platelets. The key characteristic of stem cell dynamics is their ability to undergo symmetric and asymmetric division. This is important because it allows the self-renewal of stem cells while also producing differentiated progeny for blood cell lineages. This property makes stem cell dynamics exceptionally beneficial in the context of regenerative medicine and cancer therapies.
A unique feature of stem cell dynamics is the concept of niche interaction, where stem cells interact with their microenvironment to receive signals that dictate their fate. The advantages of these dynamics include the capacity for long-term replenishment of the blood system, which is crucial for recovery after blood loss or anemia. However, a disadvantage could arise when stem cell function is impaired, leading to hematological disorders.
Differentiation Pathways
Differentiation pathways are processes through which hematopoietic stem cells develop into specific blood cell types. These pathways are finely tuned and involve several signaling mechanisms that guide stem cells through stages of lineage commitment. A key characteristic of differentiation pathways is their hierarchical nature. This hierarchy helps to efficiently produce different cell types based on the body's needs. This makes differentiation pathways essential for maintaining a balanced immune system and adequate oxygen transport.
One unique aspect of these pathways is the role of transcription factors, which regulate gene expression during differentiation. Their advantages include the ability to respond rapidly to physiological demands, ensuring a swift adaptation to stress or infection. However, the complexity of these pathways can lead to errors in differentiation, resulting in diseases such as leukemia or other blood disorders.
Immune System Regulation
Bone marrow cells also play an important role in the regulation of the immune system. Bone marrow produces various immune cells, including lymphocytes, which are crucial for the adaptive immune response. These cells respond to foreign pathogens, aiding in the development of long-term immunity. The relationship between bone marrow cells and immune functions emphasizes the integrated nature of hematopoietic processes and immune responses.
Moreover, the interaction between different cell types within the marrow, like stromal cells and hematopoietic cells, helps create a supportive environment for immune cells. This regulation is vital during infections or inflammatory responses. It becomes clear that disruption to this balance can lead to immunodeficiencies or autoimmune conditions.
Understanding the physiological functions of bone marrow cells provides essential knowledge that helps characterize their role in health and disease, guiding future research and therapeutic strategies.
Mouse Models in Biomedical Research
Mouse models are essential tools in biomedical research, providing significant insights into complex biological processes and diseases. With their genetic, biological, and behavioral characteristics closely resembling those of humans, they serve as valuable organisms to study both fundamental biological phenomena and specific pathologies. The use of mouse models in research not only facilitates the understanding of various diseases but also plays a crucial role in the safety and efficacy testing of new therapeutic interventions.
Research scientists often use these models to uncover the mechanisms of diseases, tracing through genetic and environmental factors impacting health. Voicing their advantage in experimental setups, they allow researchers to manipulate genetics, control environmental conditions, and monitor physiological responses in real-time. Additionally, mice have relatively short lifespans, yielding quicker results compared to larger animals.
Relevance of Mouse Models
Mouse models are relevant in many aspects of biomedical research. One key area is the study of genetics. Due to the advanced techniques of genetic modification available, researchers can produce specific gene knockout or transgenic mice to examine the role of certain genes in health and disease. This aids in elucidating genetic disorders and can guide the development of gene therapies.
Moreover, these models facilitate drug discovery processes. They allow for preclinical testing, where the safety and effectiveness are evaluated before human trials. In the field of cancer research, for instance, mouse models enable the investigation of tumor biology and the response to targeted treatments.
Genetic Manipulation Techniques
Knockout Models
Knockout models are engineered to have specific genes inactivated or 'knocked out.' This technique is crucial for studying the function of genes and their contributions to various biological pathways. One key characteristic of these models is their ability to reveal phenotypic consequences of losing gene function. Knockout mice can be incredibly beneficial resources for understanding genetic diseases and the roles of genes in various physiological processes.
The unique feature of knockout models is the specificity of gene targeting. Researchers can select which gene to deactivate, allowing targeted studies that are methodologically robust. However, some drawbacks include potential off-target effects and compensatory mechanisms that may obscure true phenotypic outcomes. These factors must be considered when interpreting results.
Transgenic Models
Transgenic models differ from knockout models in their purpose and methodology. They involve inserting foreign DNA into the genome of the mouse. This allows scientists to investigate gene function and regulation in vivo, providing insights into how genes affect health and disease. A key characteristic of transgenic mice is that they can be used to study gene expression and the effects of overexpression on phenotype.
A unique feature of transgenic models is the ability to drive gene expression in a controlled manner. This can be temporal or tissue-specific, which enhances the precision of experiments. Although transgenic models provide significant insights, they also have limitations. Inserting foreign DNA can sometimes lead to unpredictable expression patterns or potential insertional mutations, complicating interpretations of research findings.
Applications in Disease Modeling
Mouse models have broad applications in disease modeling. They are commonly used to replicate human diseases, enabling researchers to study disease progression and evaluate potential therapies. For instance, they have been instrumental in the study of autoimmune diseases, metabolic disorders, and neurodegenerative conditions. By evaluating responses to treatments in these models, researchers can contribute valuable data to the field of personalized medicine.
Clinical Implications of Mouse Bone Marrow Research
Mouse bone marrow research plays a significant role in advancing our understanding of various medical conditions and therapeutic strategies. The clinical implications underscore both the potential benefits and ethical considerations surrounding the use of mouse models in research. By focusing on the cellular and molecular mechanisms active within bone marrow, scientists can identify pathways relevant to human diseases and thereby enhance treatment options.
Regenerative Medicine Applications
Mouse models provide essential insights into regenerative medicine, particularly regarding how to repair or replace damaged tissues. Researchers can investigate how bone marrow-derived stem cells contribute to tissue regeneration. These cells can differentiate into various cell types, enabling the healing processes in different organs. The study of mouse bone marrow cells identifies how manipulation of these cells can optimize their regenerative capabilities.
Key aspects of regenerative medicine include:
- Stem cell therapy: Leveraging hematopoietic stem cells from mouse bone marrow can support clinical practices for various diseases such as leukemia and anemia.
- Tissue engineering: Integrating bone marrow cells into scaffolds can lead to the successful regeneration of complex tissues, including cardiac and neural tissues.
- Development of biomaterials: Research focusing on interactions between bone marrow cells and biomaterials can improve any regenerative treatment designs.
By applying findings from mouse models, researchers are better equipped to develop targeted therapies that could significantly improve patient outcomes.
Immunotherapeutic Strategies
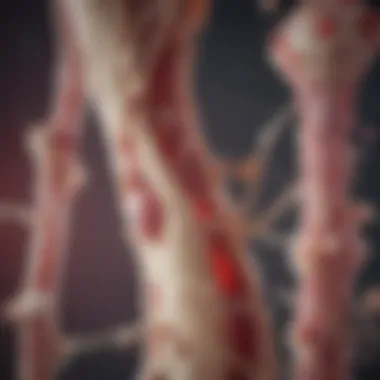
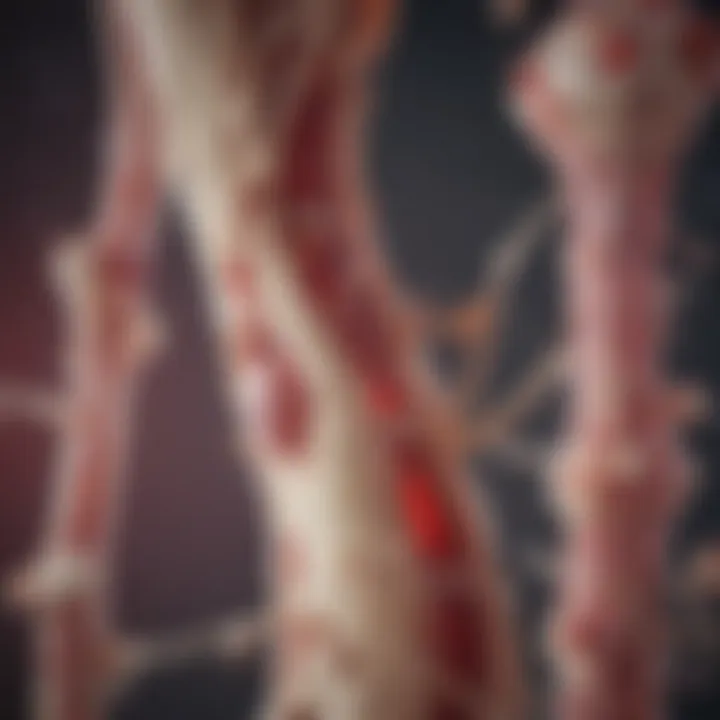
Understanding the immune landscape through mouse bone marrow cells presents a transformative avenue for immunotherapy. Bone marrow cells, particularly the various types of immune cells, play vital roles in defense mechanisms. Their characteristics and functions in modulating immune responses offer critical insights into potential immunotherapeutic applications.
Immunotherapeutic strategies influenced by mouse bone marrow research include:
- Monoclonal antibodies: Mouse models have been pivotal in developing antibody-based therapies against specific tumor antigens, enhancing the effectiveness of cancer treatments.
- Cell therapy: Analyzing immune cell populations within mouse bone marrow aids in refining T cell therapies, contributing to the rise of CAR-T cell therapies.
- Vaccine development: Understanding immune responses generated by bone marrow cells helps in designing effective vaccines, particularly against cancers or chronic infections.
Overall, the intersection between mouse bone marrow research and immunotherapy is a promising field that holds the potential for innovative treatments in various diseases.
"Through detailed exploration of mouse bone marrow cells, researchers can tailor therapies that enhance regeneration or modulate immune responses, thereby influencing future clinical practices."
Engagement with these implications ensures that the findings from mouse studies do not only remain within the lab but contribute to real-world advancements in medicine.
Ethical Considerations and Best Practices
Ethical considerations in research involving mouse bone marrow cells are essential for maintaining scientific integrity and ensuring humane treatment of animal subjects. Researchers must abide by established guidelines, as these considerations shape the legitimacy and applicability of their work. Importantly, ethical practices enhance the credibility of research findings and foster public trust in scientific endeavors.
Animal Welfare Guidelines
Animal welfare guidelines are a cornerstone of ethical research involving laboratory animals. These guidelines help to ensure that the treatment of animals used in experiments minimizes suffering and distress. Compliance with these protocols includes the following aspects:
- Housing Conditions: Animals should be housed in environments that meet their physiological and behavioral needs. This includes proper space, enrichment, and nutrition.
- Minimizing Harm: Researchers must design experiments that minimize pain and suffering. Effective pain relief should be provided whenever necessary.
- Euthanasia Methods: When euthanasia is required, it must be performed humanely and in accordance with recognized standards to minimize suffering. Appropriate training and guidelines are critical.
"The ethical treatment of animals is not only a moral obligation but also a prerequisite for valid scientific research."
Following these guidelines not only safeguards the well-being of the animals but also ensures the validity of the research outcomes. If animals are subjected to undue stress or harm, the reliability of the data generated may become questionable.
Research Compliance Standards
Research compliance standards are regulations and policies designed to ensure that research involving animals is conducted in an ethical manner. These standards vary across institutions but generally encompass the following:
- Institutional Animal Care and Use Committees (IACUC): Most research institutions require proposals involving animal studies to be reviewed by IACUC. This body evaluates the scientific justification and ethical considerations of the research.
- Reporting Requirements: Researchers are often mandated to report their findings accurately, including all data pertaining to animal welfare. Inaccurate reporting can lead to scientific misconduct.
- Training for Personnel: Those conducting research involving animals should undergo proper training on ethical standards and animal care. Continuous education reinforces best practices and compliance.
Adhering to these compliance standards ensures that research is not only ethical but also scientifically robust. Any deviation from these norms can lead to serious ramifications, including potential legal consequences and the loss of research funding.
Future Directions in Bone Marrow Cell Research
Research on mouse bone marrow cells has continuously evolved. This evolution is critical, as it drives our understanding of hematopoiesis and related pathologies. Several important trends are emerging, showing promise for future applications in therapy and understanding disease mechanisms.
Emerging techniques and technologies play a significant role in shaping these future directions. Modern advancements in biotechnology are paving the way for more precise manipulation and analysis of bone marrow cells. This includes the use of advanced imaging techniques, CRISPR gene editing, and single-cell RNA sequencing. Each of these tools enables researchers to delineate cellular functions with great accuracy. Moreover, they facilitate the exploration of complex cellular interactions that were once challenging to study, allowing researchers to observe cellular dynamics in real time.
Emerging Techniques and Technologies
The integration of new technologies into bone marrow cell research offers a range of advantages:
- Single-cell sequencing allows detailed insights into the transcriptomic profiles of individual cells. This prospective elucidation can reveal cellular heterogeneity and uncover unknown functions.
- CRISPR-based methods enable targeted edits in the genome, offering the potential to develop models for specific human diseases. Such manipulations could yield novel therapeutic strategies that rely on understanding the altered gene expression in diseases like leukemia or anemia.
- Advanced imaging techniques, such as intravital microscopy, provide real-time visualization of bone marrow interactions, which are vital for studying cell maturation and function.
As researchers adopt these novel approaches, they must also consider the implications these technologies bring. Proper standards for reproducibility and data management will be crucial to ensure findings are robust and reliable.
Potential Areas of Exploration
Looking ahead, several significant areas are ripe for investigation, warranting focused research efforts:
- Homogeneous cell populations: Understanding the distinctions between various hematopoietic stem and progenitor cells can lead to the development of targeted therapies. Identifying markers that are specific to these populations will enhance their isolation and application.
- Bone marrow microenvironment: Researching the role of the bone marrow niche and its components can unveil how cellular interactions influence hematopoiesis and immune responses. This line of inquiry may lead to innovations in regenerative medicine.
- Regenerative therapy enhancements: Efforts could be aimed at improving cell survival and function post-transplantation. Techniques that enhance cell engraftment or resilience could revolutionize treatments for different hematological disorders.
- Immunological implications: Exploring how bone marrow cells interact with the immune system could provide insights into both immune tolerance and autoimmunity. These findings are paramount, especially for developing novel immunotherapies.
Finale
The conclusion of this article synthesizes the plethora of information related to mouse bone marrow cells. It is crucial to appreciate the multi-faceted role these cells play in not only fundamental biological processes like hematopoiesis but also their applications in modern medicine. By understanding the structure and function of these cells, researchers can elucidate the mechanisms underlying various diseases and develop innovative therapeutic strategies.
Summary of Key Findings
This article provided a thorough exploration of several key aspects:
- Hematopoietic Cell Lineages: Researchers identified and classified multiple lineages, emphasizing their unique roles in blood formation. Various developmental stages highlight the dynamic nature of these cells.
- Stromal Cell Contributions: The roles of adipocytes, fibroblasts, and macrophages within the bone marrow ecosystem were articulated. Their support is vital for maintaining a healthy hematopoietic niche.
- Isolation Techniques: Methods such as flow cytometry and gene expression profiling were discussed, showcasing modern tools available for bone marrow research.
- Clinical Applications: The potential for regenerative medicine and immunotherapy foundations in bone marrow research was explored, indicating a growing avenue for practical applications of this research.
Implications for Future Research
Looking ahead, further studies involving mouse bone marrow cells promise to expand our current understanding in various directions:
- Emerging Technologies: Advances in imaging and single-cell analysis will likely allow for more detailed insights into the cellular microenvironment of bone marrow. These technologies could reveal interactions that were previously undetectable.
- Applications in Diseases: Continued exploration of bone marrow cells in models of diseases like leukemia and autoimmune disorders can provide new pathways for treatment strategies.
- Impacts on Human Health: Research outcomes can facilitate enhancements in clinical practices, particularly in stem cell therapies.
In summary, the study of mouse bone marrow cells is not just a specialized field but a cornerstone for understanding broader biological phenomena and improving health outcomes.