Mass Spectroscopy: Principles, Applications, and Challenges
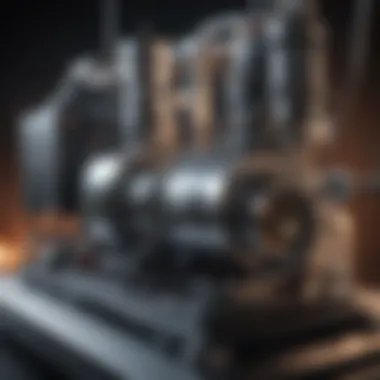
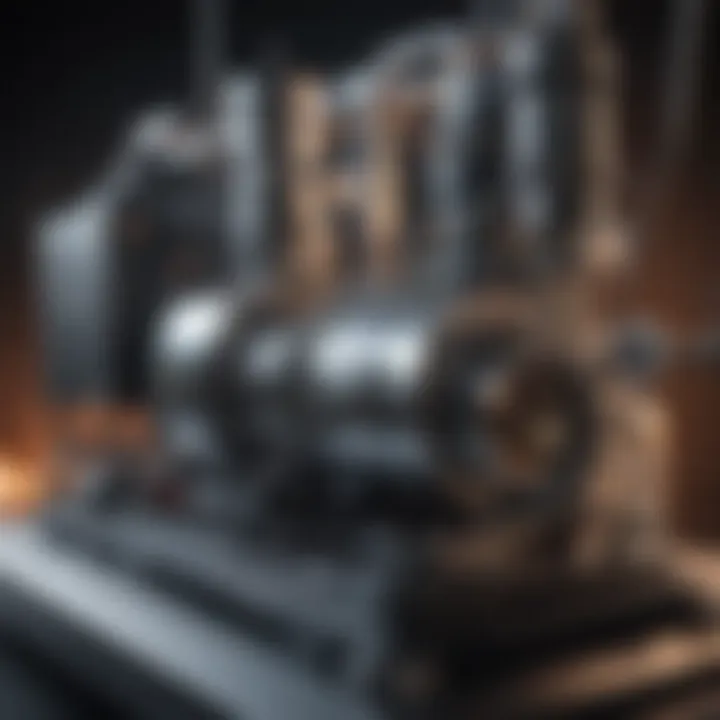
Intro
Mass spectroscopy analysis is an essential tool used in various scientific fields. This technique assists researchers in identifying and quantifying chemical substances. The complexity of materials in biology, chemistry, and other areas demands precise measurement methods. Mass spectroscopy provides this clarity through its unique principles and methods.
In this overview, we will explore the methodologies of mass spectroscopy, including the necessary tools and technologies. We will also discuss its applications and the implications for research. Lastly, we will consider the limitations and challenges this approach faces in modern science.
Understanding mass spectroscopy is crucial for both experienced researchers and those newly introduced to the field. This article seeks to provide relevant insights and useful information about this vital technique.
Intro to Mass Spectroscopy
Mass spectrometry is an important analytical technique in various scientific fields. It allows researchers to identify compounds, determine molecular weights, and analyze complex mixtures. Understanding this technique is essential for several reasons, including its vast applications in chemistry, biology, and environmental science. Through this article, we aim to present a comprehensive overview of mass spectroscopy, clarifying its principles, applications, limitations, and future directions.
Definition of Mass Spectroscopy
Mass spectroscopy is a method used to measure the mass-to-charge ratio of ions. Essentially, it involves transforming chemical compounds into ions. Once ionized, these molecules are separated based on their mass-to-charge ratios. This separation allows for detailed analysis and identification of substances. The critical components of mass spectrometry include the ion source, mass analyzer, and detector.
Historical Background
The inception of mass spectrometry dates back to the early 20th century. British scientist J.J. Thomson is often credited with the development of the first mass spectrometer in 1912. Thompson's device laid the foundation for future advancements, allowing scientists to understand molecular behaviors more clearly. Over the decades, numerous improvements have been made to mass spectrometry. For instance, the introduction of gas chromatography in the 1950s enhanced the analysis of volatile compounds, increasing the technique's applicability in chemistry and bioanalysis. More recent times have seen advancements in technology that continue to evolve this method further.
Importance in Scientific Research
Mass spectroscopy serves a critical role in scientific research. Its ability to analyze complex samples rapidly and accurately makes it invaluable in various domains. Here are some vital aspects of its significance:
- Identification of Biomolecules: In biochemistry, mass spectrometry helps in identifying proteins, peptides, and metabolites, thus driving advancements in drug discovery and proteomics.
- Environmental Monitoring: This technique is crucial for analyzing pollutants, enabling scientists to assess the impact of chemicals on ecosystems and human health.
- Quality Control: Industries depend on mass spectroscopy for quality assurance in product formulations and development.
Principles of Mass Spectroscopy
Understanding the principles of mass spectroscopy is crucial for applying this analytical technique effectively. These principles lay the foundation for the operation of mass spectrometers, influencing their performance and the results they produce. The core elements include ionization techniques, mass analysis methods, and detection strategies. Mastering these principles facilitates accurate identification and quantification of substances. This understanding is vital for researchers across various fields, enhancing the credibility and accuracy of their findings.
Ionization Techniques
Ionization is the first step in mass spectroscopy, where molecules are converted into ions to be analyzed. Different ionization techniques serve various sample types, providing versatility in applications.
Electron Ionization
Electron ionization (EI) is a widely used technique in mass spectroscopy. This process involves bombarding gas-phase molecules with high-energy electrons, leading to the production of positive ions.
One of the key characteristics of EI is its efficiency in generating fragment ions, which helps identify the structure of compounds. As a popular choice, EI offers a robust method for analyzing volatile and thermally stable samples. One unique feature of EI is its high energy. This can produce extensive fragmentation, which is advantageous for structural analysis but may also complicate the interpretation of spectra due to overlapping peaks.
Chemical Ionization
Chemical ionization (CI) differs from EI by using a chemical reaction with a reagent gas to form ions. This technique is softer, producing fewer fragment ions, which can be beneficial in obtaining molecular weight information.
A key characteristic of CI is that it often results in more straightforward spectra. This simplicity aids in the analysis of more delicate or less volatile compounds. Its unique feature is the lesser degree of fragmentation, making it easier to identify parent ions but sometimes limiting structural analysis. CI is particularly useful when analyzing complex mixtures where clarity is essential.
Electrospray Ionization
Electrospray ionization (ESI) has gained prominence in the analysis of large biomolecules, especially proteins and peptides. This technique uses a high-voltage electric field to create an aerosol of charged droplets from a solution.
The key characteristic of ESI is its ability to analyze non-volatile, thermally labile compounds. This makes it a valuable choice for biochemistry and proteomics. The unique feature of ESI is that it can produce multiple charge states, providing extensive data on molecular weight, but can also lead to clustering of similar ions that complicate analysis.
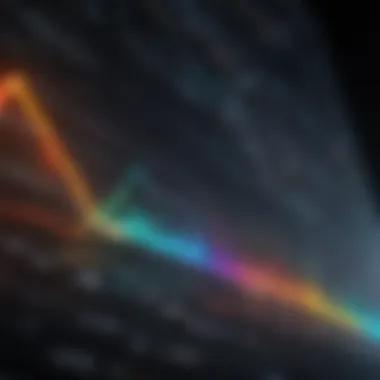
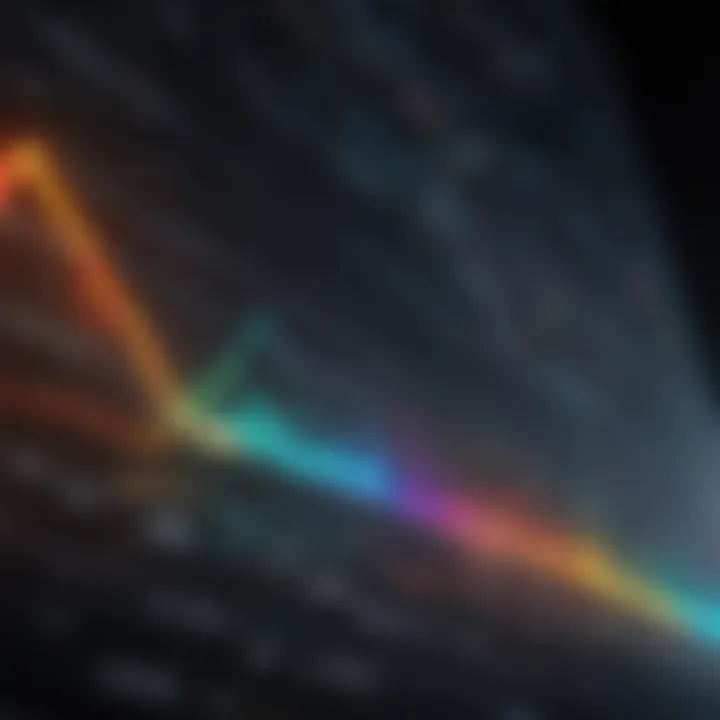
Matrix-Assisted Laser Desorption Ionization
Matrix-Assisted Laser Desorption Ionization (MALDI) is another innovative approach, particularly effective for large biomolecules. In this technique, a matrix absorbs laser light, causing it to vaporize along with the analyte.
A key characteristic of MALDI is its minimal fragmentation, which is favorable for analyzing large molecules like proteins, DNA, and polysaccharides. The unique feature of MALDI is the ability to analyze samples as solids, which can be advantageous for specific applications, but the reliance on matrix composition can introduce variability in results.
Mass Analysis Techniques
Once ions are generated, analyzing their mass-to-charge ratio is essential. Various mass analysis techniques provide the means to perform this analysis.
Time-of-Flight (TOF)
Time-of-Flight (TOF) is a technique that measures the time ions take to travel a specific distance in a vacuum after being accelerated by an electric field. TOF is favored for its ability to analyze a wide mass range in a single measurement.
The TOF method's primary advantage is its high resolution and accuracy. The unique feature of TOF is the time measurement, which can be affected by the ion's size and charge, leading to comprehensive mass analysis but requiring careful calibration and setup.
Quadrupole Mass Analyzer
Quadrupole mass analyzers utilize oscillating electric fields to allow only ions of specific mass-to-charge ratios to pass through. This is a well-established and widely used technique in mass spectroscopy.
The key characteristic of the quadrupole is its ability to filter ions efficiently. This technique is beneficial for routine analysis and provides reliable results. Its unique feature is the ability to perform multiple stages of filtering, but it may have limitations in resolving closely related compounds compared to other techniques.
Orbitrap
The Orbitrap is a mass analysis technique that captures ions in an electrostatic field, measuring their oscillations to determine their mass. This method is relatively newer compared to others but has quickly gained popularity.
The Orbitrap's main advantage is its exceptional mass resolution and accuracy, making it an attractive choice for complex mixture analysis. The unique feature of the Orbitrap is its high sensitivity to low-abundance ions, but the equipment can often be more expensive and require a steeper learning curve than traditional methods.
Detection Methods
The final step in mass spectroscopy is detecting the ions produced. Various detection methods exist, each with unique characteristics.
Electron Multiplier Detector
An electron multiplier detector is known for its sensitivity, allowing the detection of low-abundance ions. This technique amplifies the signal generated by hitting a surface and is commonly used in mass spectroscopy.
A key characteristic of this detector is its ability to achieve high sensitivity, making it a preferred choice for applications requiring detection of trace analytes. It offers rapid response time, but the downside may be increased noise at higher intensities.
Faraday Cup Detector
The Faraday cup detector works by collecting ions and measuring the resulting current. This method is straightforward and reliable, making it suitable for various applications.
The key characteristic of the Faraday cup is its simplicity and stability over a wide range of ion currents. The distinct advantage lies in its ability to directly measure ion current, but it can be less sensitive compared to other methods, which may limit its use for low-abundance ions.
Data Analysis in Mass Spectroscopy
Data analysis in mass spectroscopy is a crucial component that can significantly influence the results of any analytical study. The precision with which data is collected and interpreted can determine the validity of the scientific conclusions drawn from that data. Understanding how to effectively analyze mass spectra allows researchers to identify compounds accurately, quantify their concentrations, and draw meaningful insights from complex mixtures. Effective analysis directly impacts fields such as chemistry, biology, and environmental science, where knowing the exact compositions of substances is vital.
Mass Spectrum Interpretation
Interpreting mass spectra is not a simple task; it requires a strong grasp of both the theory behind mass spectroscopy and practical experience. The mass spectrum presents data in a graphical form, with intensity on the vertical axis and mass-to-charge ratio (m/z) on the horizontal axis.
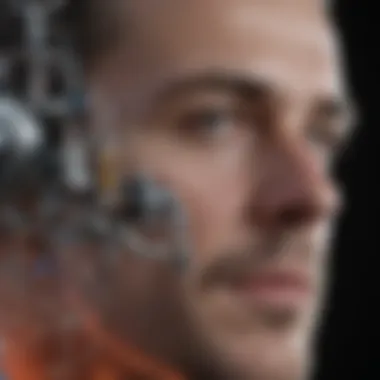
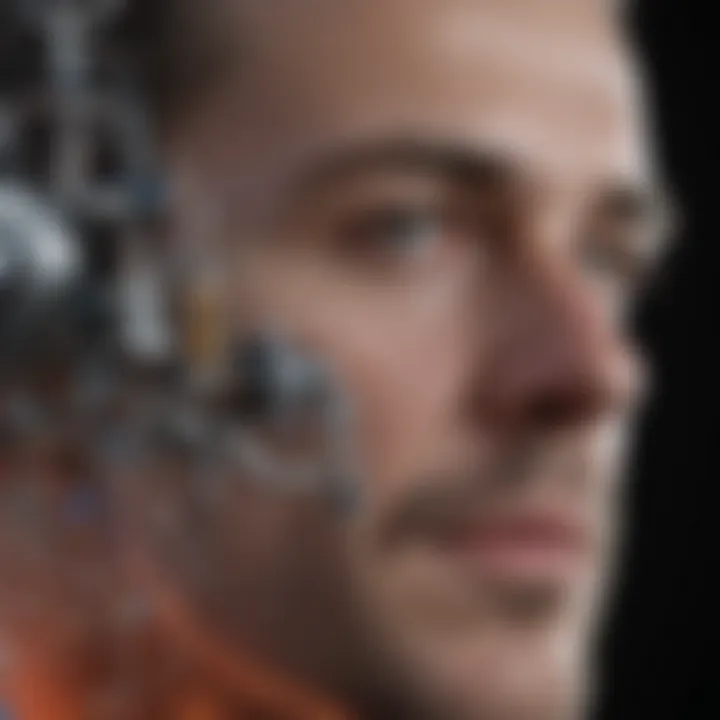
Key points in interpretation include:
- Peaks as Identifiers: Each peak corresponds to an ion. The position of a peak indicates the mass-to-charge ratio, while its height reflects the abundance of the ion. Understanding how to match these data points to known compounds forms the basis of identification.
- Isotopic Patterns: Mass spectra often display isotopic distributions which can offer clues about the elemental makeup of a compound. Analyzing these patterns can shed light on the molecular formula.
- Fragmentation Patterns: Knowledge of how molecules fragment helps in elucidating structural information. Each compound may produce a unique set of fragments, creating a spectral fingerprint.
"An accurate interpretation of mass spectra can reveal not only the identity but also the structure of the analyte."
Software Tools for Data Processing
The complexity of raw data generated by mass spectrometry necessitates the use of sophisticated software tools for effective analysis. These tools allow for both automation of tedious tasks and the application of advanced algorithms, which enhance data processing efficiency and accuracy. Common software tools include:
- Molecular Weight Calculator: Helps determine molecular weights of unknown samples.
- Software like OpenMS or Skyline: Focus on quantification and visualization. These tools are often capable of integrating with various spectrometric platforms, allowing streamlined workflows.
- Data Analysis Suites: Such as Thermo Fisher’s FreeStyle or Agilent’s MassHunter, which provide comprehensive toolsets for analysis, interpretation, and reporting.
These software solutions facilitate the processing of large datasets and help in achieving consistency in the results. Many also offer features for statistical analysis and can accommodate the needs of both quantitative and qualitative analysis, tailoring the experience to the specific requirements of various investigations.
Quantitative vs. Qualitative Analysis
Understanding the distinction between quantitative and qualitative analysis is fundamental for effective mass spectroscopy data interpretation.
- Quantitative Analysis: This involves measuring the amount of a substance in a sample. Researchers can achieve this through methods like calibration curves or internal standards to ascertain concentration levels. The precision of quantitation hinges on sample preparation, instrument calibration, and analytical techniques.
- Qualitative Analysis: This focuses on identifying compounds rather than measuring their quantity. Techniques involve comparing spectra against databases and utilizing libraries of known spectra. For researchers, qualitative analysis is essential during hypothesis testing or exploring unknown samples.
Understanding data analysis intricacies can greatly enhance the quality and reliability of the results obtained from mass spectroscopy.
Applications of Mass Spectroscopy
Mass spectroscopy is not only a tool for identifying chemical substances but also finds vast applications across various fields. Its versatility allows scientists and researchers to analyze complex mixtures in different settings. By applying mass spectroscopy in chemistry, biomedical research, environmental science, and industry, one can gain insights that directly influence practical applications and advancements in technology and health.
Applications in Chemistry
In chemistry, mass spectroscopy serves as a fundamental analytical tool. It helps chemists identify molecular structures and determine the molecular weight of compounds. The technique enables the elucidation of complex organic structures, especially in the field of natural products chemistry. Moreover, mass spectroscopy aids in the characterization of polymers, ensuring quality control in product formulation. The combination of mass spectrometry with techniques such as chromatography amplifies its effectiveness, enabling the separation and identification of components in a mixture.
Mass spectroscopy is crucial in understanding the composition of materials and the dynamics of chemical reactions.
Role in Biomedical Research
Biomedical research heavily relies on mass spectroscopy for various applications. It is instrumental in proteomics, allowing researchers to analyze proteins in complex biological samples. Mass spectroscopy facilitates the quantification of biomolecules, which is essential in drug development and biomarker discovery. Furthermore, by using targeted mass spectrometry techniques, scientists can investigate metabolic pathways, leading to better understanding of disease mechanisms. The accuracy and sensitivity of mass spectroscopy make it invaluable for clinical diagnostics.
Environmental Analysis
Environmental applications of mass spectroscopy address pressing issues related to pollution and safety. The technique assists in monitoring trace contaminants in air, water, and soil samples. Accurate identification of environmental pollutants provides insights into their sources and impacts. For example, mass spectrometry can detect persistent organic pollutants (POPs) that pose risks to both human health and ecosystems. This analytical capability allows for better environmental assessment and regulatory compliance, promoting sustainability and safety.
Industrial Applications
In industrial contexts, mass spectroscopy plays a crucial role in quality assurance and process optimization. Industries, such as pharmaceuticals, agriculture, and food production, utilize mass spectroscopy to ensure product safety and efficacy. It helps monitor raw materials, validate formulations, and ensure consistent production quality. Additionally, mass spectrometry supports research in developing new materials and innovative processes. The integration of mass spectroscopy in industrial settings boosts efficiency and reliability, addressing consumer demands effectively.
Overall, applications of mass spectroscopy continue to expand across fields, showcasing its importance in enhancing scientific understanding and addressing real-world challenges.
Limitations and Challenges in Mass Spectroscopy
Understanding the limitations and challenges associated with mass spectroscpy is crucial for advancing its applications and ensuring accurate results. Despite its prominence in analytical chemistry, mass spectroscopy is not without its flaws. Recognizing these issues allows scientists and researchers to innovate and develop better methodologies. Addressing limitations can help refine the techniques and improve the reliability of data gathered through mass spectroscopy.
Sensitivity Issues
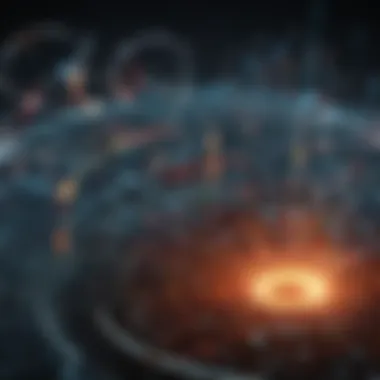
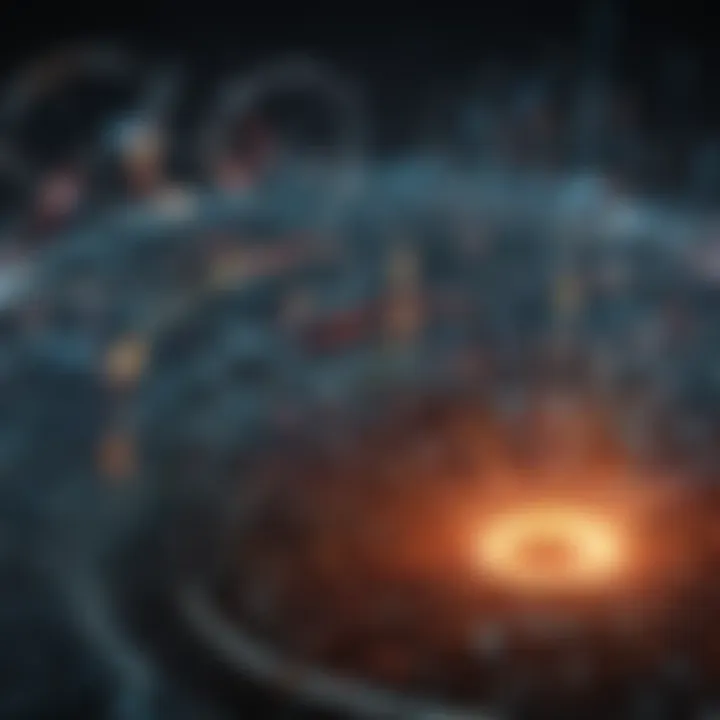
Sensitivity is a key factor in mass spectroscopy. While most mass spectrometers can detect small quantities of substances, the sensitivity levels may vary significantly among different ionization techniques and types of mass analyzers. Some compounds, particularly those with low molecular weights or those that are thermally labile, may not ionize efficiently. As a result, their detection can be compromised, leading to inaccuracies in quantification and identification. This poses a challenge, especially when analyzing complex biological samples where the quantity of target analytes is low compared to other background substances. To enhance sensitivity, researchers often need to optimize ionization conditions or adopt more sophisticated mass spectrometric methods.
Matrix Effects on Ionization
Matrix effects are a well-known issue in mass spectroscopy. The presence of other compounds in a sample can influence the ionization efficiency of the target analytes. For instance, ions produced from the matrix might compete with the target analytes, leading to suppression or enhancement of the signal. This phenomenon can drastically alter the interpretation of mass spectra, making it challenging to establish accurate quantitative and qualitative analyses. To mitigate matrix effects, scientists may employ sample preparation techniques like solid-phase extraction or use internal standards to calibrate the responses of the analyzes.
Complex Data Analysis
Data analysis in mass spectroscopy can be exceedingly complex. The mass spectra generated contain a wealth of information, but interpreting these spectra demands a sophisticated understanding of both the technique and the software tools used for analysis. Many researchers find themselves overwhelmed by large datasets, particularly when dealing with high-resolution spectral data. The complexity is further intensified when considering overlapping peaks and isotopic patterns. Hence, effective data processing not only requires strong analytical skills but also proficiency with advanced software tools designed for mass spectral analysis. Continuous development in this area is crucial to enhance understanding and facilitate more accessible analysis methods.
"Mass spectroscopy holds immense potential, yet its effectiveness heavily relies on the capability to manage its limitations."
In summary, while mass spectroscopy is a powerful analytical tool, its limitations must be carefully navigated. Sensitivity issues, matrix effects on ionization, and complex data are significant challenges that demand attention. By understanding these limitations, researchers can better design experiments and choose appropriate methodologies to ensure the reliability and accuracy of their mass spectral analyses.
Future Developments in Mass Spectroscopy
The future of mass spectroscopy promises exciting advances that could reshape the landscape of analytical chemistry. As technology evolves, so do the methodologies employed in mass spectroscopy. This section will explore several critical areas where innovations are likely to occur. Focusing on ionization techniques, detection methods, and the integration with other analytical techniques, we can gain insights into how these developments will enhance the efficacy and application of mass spectroscopy.
Innovations in Ionization Techniques
Traditionally, ionization techniques have set the foundation for obtaining mass spectra. New innovations are emerging in this area, enhancing both the sensitivity and specificity of ionization processes. For instance, research is ongoing into ambient ionization techniques, which allow for the direct analysis of samples without the need for extensive sample preparation. Techniques such as desorption electrospray ionization (DESI) are being developed, allowing for in-situ analysis of surfaces and fluids. These methods not only streamline workflows but also integrate seamlessly with natural environments.
Another area of focus is the improvement of existing methods like Electrospray Ionization. Enhancements to this technique may include the development of more stable ion sources or the application of higher electric fields to increase the efficiency of ion formation. Such innovations could drastically reduce the limit of detection for various compounds, making mass spectrometry a choice technique in trace analysis.
Advancements in Detection Methods
The effectiveness of mass spectroscopy heavily relies on its detection capabilities. Recent advancements are emerging in detector technology, aimed at increasing precision and reducing noise. For example, developments in hybrid detector systems combine multiple detection methods, improving the dynamic range of mass spectrometers.
These innovations also involve enhancing current technologies. The use of time-of-flight (TOF) detection systems has seen significant improvements, leading to faster analysis times and higher resolution. Another promising advancement is the introduction of single-molecule detection techniques, which could revolutionize the quantification of low-abundance compounds. The ability to analyze extremely small quantities with high fidelity offers potential in areas such as biomarker discovery and environmental monitoring.
Integration with Other Analytical Techniques
As the complexity of samples increases, so does the need for more robust analytical strategies. Future developments will see a stronger integration of mass spectroscopy with other techniques, like chromatography and nuclear magnetic resonance (NMR). This synergy will enhance the analytical capability by combining the strengths of each technique, leading to more comprehensive data.
One example of this integration is the combination of mass spectrometry with high-performance liquid chromatography (HPLC). This method improves separation before mass analysis, leading to clearer and more distinct mass spectra. Overall, such combinations can facilitate multidimensional analysis, aiding researchers in tackling complex biological and chemical samples effectively.
The ongoing advancements in mass spectroscopy are not just enhancing existing capabilities; they are opening new avenues for research and application.
"The future of mass spectroscopy is not just about detection, it is about enhancing understanding across disciplines. As methods evolve, our ability to solve complex problems increases."
The culmination of these developments indicates a promising horizon for mass spectroscopy, solidifying its role as an indispensable tool in scientific discovery.
End
The Conclusion section holds significant importance in this article as it encapsulates the essence of mass spectroscopy analysis. It serves to reinforce the key points discussed throughout the narrative, facilitating a clearer understanding for the reader.
This technique is crucial for the identification and quantification of chemical substances across various fields, most notably in chemistry and biology. As we delve into the summary of critical aspects, readers can grasp the transformative impact of mass spectroscopy on scientific research and industry applications.
Summary of Key Points
In the discussion, several aspects have been highlighted:
- Definition of Mass Spectroscopy: A technique that measures the mass-to-charge ratio of ions, allowing for the identification of substances.
- Historical Impact: Understanding the evolution of mass spectroscopy underscores its growing relevance in scientific advancements and techniques.
- Ionization Techniques: The various methods, such as Electron Ionization and Electrospray Ionization, are essential for producing ions from compounds for analysis.
- Mass Analysis Techniques: Instruments used to separate ions based on mass, including Time-of-Flight and Quadrupole mass analyzers, contribute to the accuracy of the results.
- Applications: Its diverse use in chemistry, biomedical research, and industry showcases its adaptability and significance in solving complex problems.
- Limitations: Acknowledging challenges such as sensitivity issues and matrix effects encourages continuous improvement in methodologies.
- Future Developments: Innovations in detection methods and integration with other techniques signal a forward trajectory that promises enhanced efficacy in analysis.
The integration of mass spectroscopy with other analytical techniques is likely to revolutionize current methodologies, presenting opportunities for deeper insights into chemical composition.
As we encapsulate these insights, it becomes evident that mass spectroscopy is not just a technical method; it is a foundational pillar in contemporary analytical chemistry, holding immense potential for future research and application. An understanding of the comprehensive landscape provided in this article will prepare readers for further exploration in their respective fields.