Exploring Iron(III) Oxide: Properties and Applications
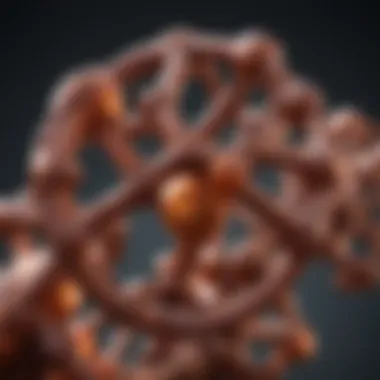
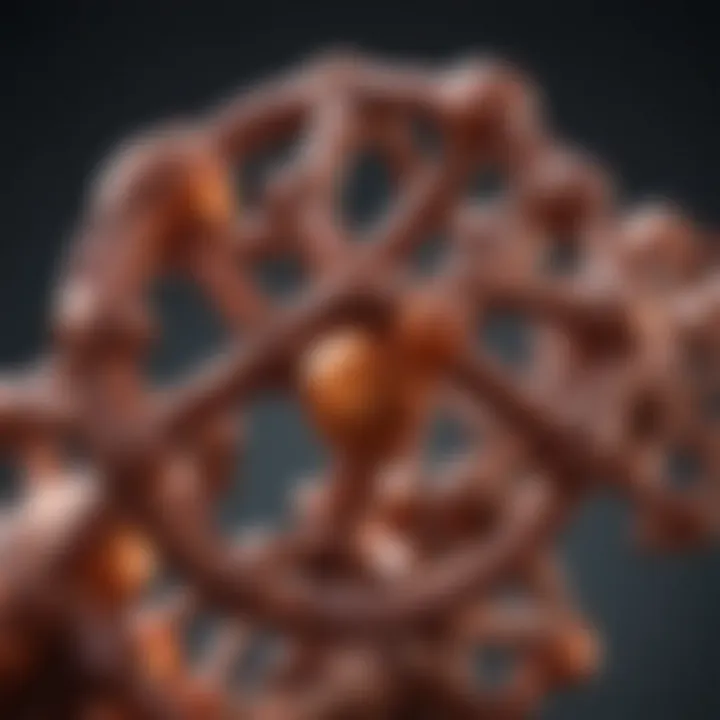
Intro
Iron(III) oxide, commonly denoted as Fe2O3, is more than just a simple compound. It plays a crucial role across various scientific domains, ranging from chemistry to environmental studies. This article provides a thorough analysis of Fe2O3, exploring its properties, applications, and implications.
As a ubiquitous material, Iron(III) oxide is found in nature in several forms, including hematite and magnetite. Its significance is not limited to academic research. Industries heavily rely on this compound for multiple applications, including catalysis and pigment production.
In this analysis, we will discuss its chemical properties, synthesis methods, and applications, alongside the environmental implications of its usage. Our objective is to equip researchers and professionals with a deep understanding of this vital compound and its relevance in today’s fast-paced technological landscape.
Preface to Iron() Oxide
Iron(III) oxide, or Fe2O3, is a compound that plays a critical role in various scientific disciplines. Its significance spans from materials science to environmental studies. Understanding its properties, applications, and implications is crucial for both academic and industry professionals.
Essentially, Iron(III) oxide is ubiquitous, finding applications in various sectors such as construction, electronics, and catalysis. Each domain utilizes its unique properties, making it a versatile material.
Definition and Composition
Iron(III) oxide is an inorganic compound composed of iron and oxygen. It consists of two iron atoms for every three oxygen atoms (Fe2O3). It appears in several forms, most notably as the mineral hematite, which is distinguished by its reddish-brown color. This compound can exist naturally or be synthesized in laboratories.
The molecular structure of Fe2O3 contributes to its unique properties. It is primarily known for its stability, resistnace to heat, and chemical reactivity. Due to its iron content, it has magnetic properties, making it valuable in various applications, particularly in electronics and data storage.
Historical Background
The history of Iron(III) oxide is rich. A compound known since ancient times, hematite was used by early humans for pigments and tool making. Its importance grew with the industrial revolution, leading to a surge in demand for iron-based materials.
In modern times, the understanding of Fe2O3 has advanced significantly. Research into its various applications, such as in catalysis and environmental remediation, continues to evolve. Today, its practical uses are more diverse than ever, and ongoing research aims to unlock even greater potential of this compound.
"The journey of Iron(III) oxide from a simple mineral to a key player in advanced technology showcases the evolving nature of material science."
In summary, Iron(III) oxide is not just a compound but a cornerstone of various scientific and industrial fields. Its definition, composition, and historical context provide a solid foundation for further exploration into its chemical properties, applications, and implications.
Chemical Properties of Fe2O3
The chemical properties of Iron(III) oxide, or Fe2O3, underpin its relevance across various domains, from material science to environmental applications. Understanding these properties is essential for its effective use in different technologies and processes. Fe2O3 exhibits distinct characteristics in molecular structure, thermal stability, and reactivity that make it suitable for a range of applications.
Molecular Structure
Iron(III) oxide has a complex molecular structure. In the bulk form, it mainly presents as a crystalline solid. Two primary structures are hematite and maghemite. Hematite primarily has a rhombohedral crystal system, whereas maghemite often displays a cubic structure. This structure significantly influences its physicochemical properties. For instance, the arrangement of iron and oxygen atoms affects how Fe2O3 interacts with other compounds, making it a good candidate for various chemical reactions.
Thermal Stability
Thermal stability is another crucial aspect of Fe2O3. This compound is stable at high temperatures, which is useful for processes such as sintering in metallurgy. When heated, Fe2O3 can undergo phase transitions, particularly from hematite to magnetite, at temperatures above approximately 500 °C. Understanding its thermal behavior can help in optimizing its use in industrial applications, especially in processes where temperature control is vital. Moreover, the stability of Fe2O3 at elevated temperatures ensures its reliability in catalytic reactions where heat is a factor.
Reactivity and Reactions
Reactivity of Fe2O3 varies based on environmental conditions. This compound can participate in redox reactions, acting as both an oxidizing and reducing agent. In acid-basic reactions, it can dissolve in acids, yielding iron ions. This property can be exploited in waste treatment facilities where Fe2O3 can assist in contaminant removal. Additionally, it can serve as a catalyst in various organic reactions, enhancing production efficiency. Significant interactions include:
- Oxidation of organic matter
- Reduction reactions under specific conditions
- Interaction with various acids in aqueous solutions
Understanding these reactivity patterns is crucial for researchers and professionals working with Fe2O3, as they inform its application in both industrial and environmental contexts.
"The adaptability of Fe2O3 in different chemical environments highlights its importance in research and practical applications."
Overall, the chemical properties of Iron(III) oxide serve as foundational knowledge for its utilization across scientific fields. Recognizing how molecular structure influences reactivity and stability is essential for advancing technologies that rely on this compound.
Preparation and Synthesis
The preparation and synthesis of Iron(III) oxide (Fe2O3) are crucial topics in understanding its versatility and applications. The choice of synthesis method influences its properties, including purity, particle size, and morphology. Researchers and industry professionals must be aware of each method's benefits and limitations to optimize results for specific applications.
Chemical Methods
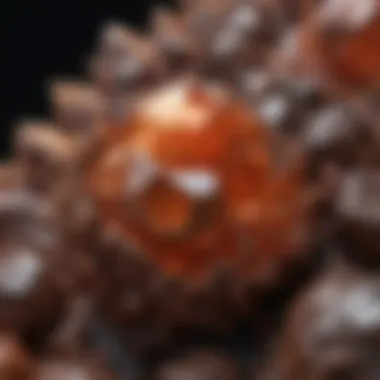
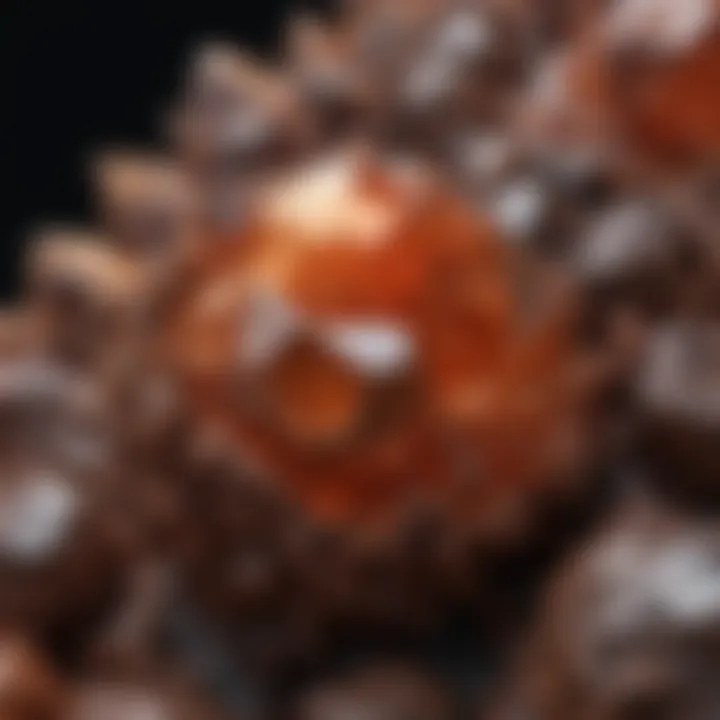
Fe2O3 can be synthesized through various chemical methods, each offering unique advantages. One common approach involves the precipitation of iron (III) salts, such as iron (III) chloride, by adding a base like sodium hydroxide. This results in the formation of iron hydroxide, which can be subsequently oxidized to Fe2O3. This method is valued for its simplicity and effectiveness in producing high-purity Fe2O3.
Another effective chemical method is sol-gel processing, where metal alkoxides are converted into metal oxides. In this process, an iron alkoxide undergoes hydrolysis and polymerization, forming a gel that, upon calcination, yields iron oxide. This technique allows for better control over the material's properties and is advantageous for applications requiring homogeneous materials.
Thermal Decomposition
Thermal decomposition is another significant method for synthesizing Iron(III) oxide. This generally involves heating iron-containing precursors like iron hydroxide or iron oxalate to high temperatures. Through controlled heating, these precursors lose their water or carbon dioxide components, leading to the formation of Fe2O3. The thermal stability of the product is enhanced by this method, which also aids in producing larger quantities of the material. However, the risk of agglomeration requires careful temperature control, as it can affect the particle size distribution.
Sustainable Production Techniques
As the demand for environmentally-friendly practices grows, sustainable production techniques for Fe2O3 are gaining prominence. One innovative approach is the utilization of biomass as a reducing agent in the synthesis process. This technique not only reduces waste but also minimizes energy consumption. Furthermore, researchers are exploring the recycling of industrial by-products containing iron to synthesize Fe2O3. This method effectively transforms waste into valuable materials, aligning with circular economy principles.
Moreover, the use of green solvents in extraction processes is being studied. These solvents can replace traditional toxic solvents, leading to safer and more sustainable synthesis methods. By focusing on sustainable techniques, the industry can contribute to lowering greenhouse gas emissions while also producing high-quality materials.
Crystal Structures of Fe2O3
The study of crystal structures of Iron(III) oxide (Fe2O3) is fundamental in understanding its properties and applications. The arrangement of atoms in a crystal lattice greatly influences the chemical behavior, stability, and potential use of Fe2O3 in various fields. The two primary forms of Fe2O3, hematite and maghemite, exhibit unique structural features that set them apart.
Hematite Structure
Hematite is the most stable and prevalent form of iron(III) oxide. Its crystal structure is known to adopt a rhombohedral lattice system, characterized by a specific arrangement of iron ions and oxygen ions. In hematite, each iron ion is coordinated by six oxygen ions, forming octahedral coordination. This leads to the formation of a layered structure, where iron and oxygen atoms alternate in arrangement.
The importance of this structure lies in its magnetic properties and red coloration. Hematite is often used as a pigment in cosmetics and paints due to its vibrant hue. Furthermore, its stability at high temperatures makes it suitable for applications in metallurgy and as a catalyst in chemical reactions.
Maghemite Structure
Maghemite, another form of Fe2O3, has a cubic spinel structure. While it bears resemblance to hematite, maghemite exhibits a defect in its oxygen lattice, which results in a different magnetic and electronic behavior. The cubic arrangement allows for better ionic mobility, making maghemite particularly useful in certain catalysts.
In practical terms, maghemite is often used in data storage devices, due to its unique magnetic properties. Its structure allows for a higher density of information storage compared to other materials. This presents a significant advantage in technological applications, especially in the fields of magnetism and electronics.
Understand the significance of the crystal structure of Fe2O3 provides insights into its varied applications and effectiveness in specific roles.
Overall, the crystal structures of hematite and maghemite are critical to the understanding of Iron(III) oxide. They determine the material's properties, influencing its implementation in both industrial and environmental applications. As research progresses, elucidating these structures may unveil new potential applications and innovations.
Applications of Fe2O3
Iron(III) oxide (Fe2O3) exhibits a wide range of applications across various fields, significantly impacting both industrial processes and environmental sustainability. This section details the most notable uses of Fe2O3, emphasizing its critical role in the development of several technologies and its application in addressing ecological challenges.
Industrial Uses
Fe2O3 finds extensive application in several industries due to its unique properties. One of its primary uses is as a pigment. Iron(III) oxide is commonly found in paints, coatings, and plastics, where it provides vibrant colors and improves durability. The red and yellow hues produced by Fe2O3 are particularly desirable for architectural purposes.
In steel production, Fe2O3 serves as a key component in various iron-making processes. Its presence is crucial in reducing iron ores to metallic iron, which is a significant step in the manufacturing of steel.
Additionally, the compound acts as a catalyst in several chemical reactions, facilitating the synthesis of various organic compounds. Examples include its use in the oxidation of organic compounds and reactions involving hydrogen production. Furthermore, the abrasiveness of Fe2O3 makes it suitable for polishing agents in metal finishing, further showcasing its versatility.
Environmental Applications
Iron(III) oxide plays a critical role in environmental applications, particularly in soil and water treatment processes. One of its significant roles in soil chemistry is as a stabilizing agent for contaminants. Fe2O3 helps immobilize heavy metals in contaminated soils, mitigating their mobility and bioavailability. This property aids in the remediation of polluted sites, enhancing soil quality.
When it comes to water quality, Fe2O3 is utilized as a coagulant in wastewater treatment. It assists in the removal of suspended solid particulates and toxins, improving overall water clarity. This application demonstrates its importance in maintaining ecosystems and protecting marine life from harmful substances.
Another noteworthy environmental application is found in the field of photocatalysis, where Fe2O3 is employed in the degradation of organic pollutants under sunlight. This process not only cleans up polluted waters but also promotes the utilization of solar energy, making it a dual benefit for ecosystem restoration.
In Innovative Technologies
The utilization of Iron(III) oxide is becoming more prevalent in modern technological applications. Its properties have led to advancements in solar energy conversion technologies. Fe2O3 is used in photocatalytic applications, aiding in the transformation of solar energy into chemical energy. This innovation has significant implications for renewable energy, addressing climate change and promoting sustainable practices.
Moreover, research is exploring Fe2O3's role in advanced battery technology. Its ability to enhance the energy density of lithium-ion batteries could lead to more efficient energy storage solutions. As renewable energy systems become more integrated into our lives, the importance of Fe2O3 in these emerging technologies cannot be overstated.
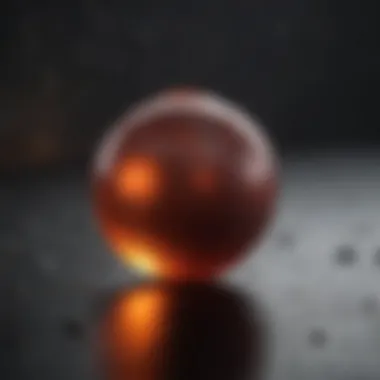
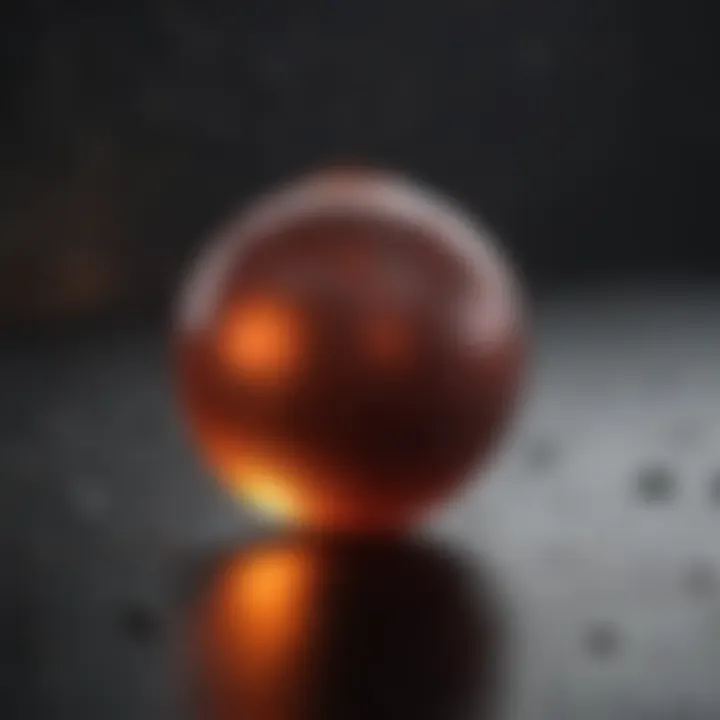
By understanding and promoting the applications of Fe2O3, we enable innovations that align with ecological and industrial needs.
Iron() Oxide in Environmental Science
Iron(III) oxide (Fe2O3) plays a vital role in environmental science. Its unique properties make it significant in various ecological processes. In this section, we will discuss three key areas: its role in soil chemistry, its impact on water quality, and its bioremediation potential. Understanding these aspects is crucial for appreciating Fe2O3’s implications on the environment and for developing sustainable practices.
Role in Soil Chemistry
In the context of soil chemistry, Iron(III) oxide acts as a key component of soil minerals. It contributes to the formation and stability of soil aggregates, which are essential for maintaining soil structure. This stabilization supports water retention and nutrient availability for plant growth. Additionally, Fe2O3 can influence the bioavailability of nutrients, such as phosphorus. When iron oxides are present, they can bind with phosphorus, making it less accessible to plants. However, under certain conditions, Iron(III) oxide can release these nutrients, thereby enhancing soil fertility.
Moreover, the redox reactions of Iron(III) oxide are crucial for the soil's microbial activity. Microbial populations often rely on iron for metabolic processes. Thus, the presence of Fe2O3 in soils can affect not only physical properties but also biological interactions within the ecosystem.
Impact on Water Quality
Iron(III) oxide significantly impacts water quality. When present in aquatic environments, it can affect the solubility of various metals. Fe2O3 has a sorption capacity which allows it to bind heavy metals, such as lead and cadmium. This process reduces the concentration of toxic elements in water. However, this can also lead to a challenge; in certain conditions, these metals may be released back into the water, depending on pH or redox conditions.
Furthermore, the presence of Iron(III) oxide contributes to the visual clarity of water bodies. It precipitates as sediment, which can improve light penetration, thereby promoting aquatic plant growth. However, excessive amounts can lead to turbidity, which may have negative effects on aquatic life.
Bioremediation Potential
The potential of Iron(III) oxide in bioremediation is promising. It can facilitate the degradation of organic pollutants through various microbial processes. Fe2O3 can act as an electron acceptor in anaerobic conditions, supporting microbial metabolism that breaks down contaminants. This is especially relevant in sites affected by petroleum hydrocarbons and other organic pollutants.
Additionally, as a low-cost material, Iron(III) oxide offers a sustainable option for environmental clean-up. Its abundance and relative non-toxicity make it a favorable choice for remediation strategies. Ongoing research aims to optimize its applications in treating contaminated soils and waters effectively.
Iron(III) oxide bridges multiple realms of environmental science, offering insights into soil health and water quality while presenting innovative avenues for remediation.
Fe2O3 and Catalysis
Iron(III) oxide (Fe2O3) serves a crucial role in the field of catalysis. Its ability to facilitate various chemical reactions makes it a valuable component in both industrial applications and environmental processes. The properties of Fe2O3 provide unique advantages in catalytic processes. This section explores those properties, benefits, and considerations of using Fe2O3 as a catalyst.
Catalytic Properties
Fe2O3 displays several catalytic properties that enable its use in diverse reactions. One important aspect is its high surface area. This quality enhances the interaction between the catalyst and the reactants. The surface sites of Fe2O3 provide numerous active centers for catalysis, which increases reaction rates substantially.
In addition, Fe2O3 is thermally stable, allowing it to maintain performance under various temperatures, which is essential for industrial applications. Furthermore, it can participate in redox reactions due to its ability to switch between different oxidation states. This flexibility enhances its utility in catalyzing both oxidation and reduction processes.
Applications in Chemical Reactions
Fe2O3 has a wide range of applications in chemical reactions. Some prominent examples include:
- Fischer-Tropsch Synthesis: In producing liquid hydrocarbons from carbon monoxide and hydrogen, Fe2O3 acts as a catalyst to optimize yields.
- Decomposition Reactions: Fe2O3 can facilitate the breakdown of pollutants in environmental chemistry, turning harmful compounds into less toxic forms.
- Epoxidation: Its use in reactions to convert alkenes into epoxides is valuable in making chemical intermediates for various products.
"Iron(III) oxide showcases versatility, enabling advancements across fields such as energy production, environmental remediation, and materials science."
The applications of Fe2O3 reflect its significance in enhancing chemical processes. By examining both its catalytic properties and real-world applications, we can appreciate the breadth of Fe2O3's role in catalysis.
Iron() Oxide in Solar Energy Conversion
Iron(III) oxide, or Fe2O3, has gained prominence in solar energy conversion, thanks to its unique properties and advantages over other materials. This section examines how Fe2O3 is utilized in solar energy applications, the specific benefits it brings, and some considerations for its use in this critical field.
Photocatalytic Applications
Photocatalysis is a process where light energy is used to accelerate a chemical reaction. Fe2O3 plays a prominent role in this process because of its photocatalytic properties. When exposed to sunlight, Fe2O3 can produce reactive species that facilitate various reactions, such as water splitting and pollutant degradation.
Some notable benefits of using Fe2O3 in photocatalytic applications include:
- Stability: Iron(III) oxide is chemically stable, which enhances its longevity in photocatalytic systems.
- Non-toxicity: Unlike some metal oxides, Fe2O3 is non-toxic and environmentally friendly, promoting sustainable practices.
- Cost-effectiveness: Fe2O3 is relatively inexpensive compared to alternatives, making it accessible for extensive applications.
The potential for using Fe2O3 in photocatalysis extends to environmental remediation, where iron oxide can help degrade harmful pollutants in water, thus contributing to cleaner ecosystems.
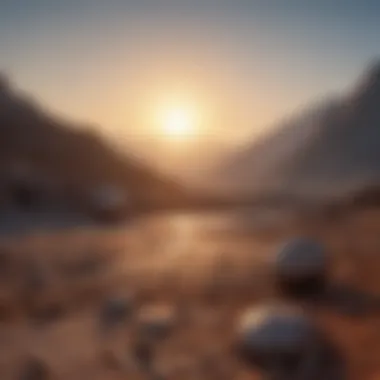
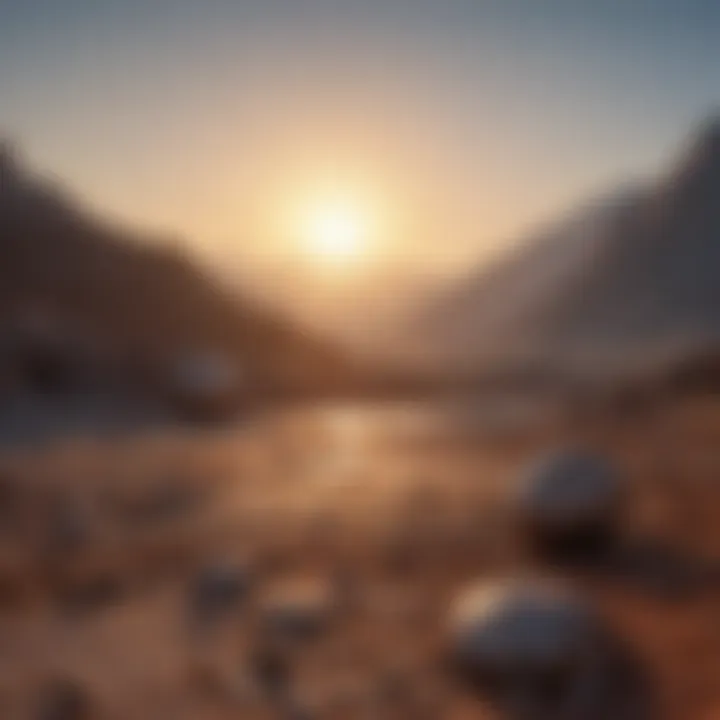
Fe2O3 in Photovoltaic Cells
Iron(III) oxide's role in photovoltaic cells is growing. While traditional solar cells often rely on silicon, research has shown that Fe2O3 can enhance performance in certain types of solar energy systems. It serves as a suitable material for both anti-reflective coatings and as a part of composite materials.
Some aspects to consider include:
- Light Absorption: Fe2O3 has a tunable bandgap, which allows it to effectively absorb a broader spectrum of sunlight, contributing to higher efficiencies in energy conversion.
- Doping Potential: Developers can dope Fe2O3 with other elements to improve its electrical properties, further optimizing its usage in solar cells.
- Integration with Other Technologies: It can be combined with other materials, such as perovskites, in tandem solar cells, potentially leading to breakthroughs in efficiency.
"The integration of Fe2O3 into solar energy systems not only combines its affordability with efficiency but also paves the way for eco-friendly technologies that address global energy needs."
Challenges and Limitations of Fe2O3 Applications
The use of Iron(III) oxide (Fe2O3) spans across various fields, yet its applications come with challenges and limitations that merit thorough examination. Understanding these issues is vital for both researchers and industry professionals who seek to leverage its properties. Here, we discuss two critical aspects: material limitations and economic considerations.
Material Limitations
Despite its diverse applications, Fe2O3 presents several material limitations that can restrict its functionality. One primary concern is its thermal stability. While hematite (the most stable form of Fe2O3) withstands high temperatures, it can undergo phase transitions that may alter its properties under specific conditions. For example, when exposed to reducing atmospheres, Fe2O3 can convert to magnetite (Fe3O4), potentially affecting its application where phase purity is crucial.
Additionally, the particle size and morphology of Fe2O3 influence its performance. For certain applications, ultra-fine particles are necessary to achieve desired reactivity. However, synthesizing these fine particles often leads to issues like agglomeration, which reduces surface area and, subsequently, reactivity. Also, the surface defects may lead to varied catalytic behavior, complicating its use in catalysis. Understanding these material constraints is essential for optimizing Fe2O3 usage in various contexts.
Economic Considerations
The economic viability of using Iron(III) oxide is another significant challenge. While Fe2O3 is abundant, its extraction and processing involve costs that can vary depending on factors like location and processing technology. The economic implications are particularly pronounced in industries where profit margins are tight. If sourcing or processing costs fluctuate greatly, this can influence the feasibility of employing Fe2O3 in large-scale applications.
Moreover, competition from alternative materials can drive costs and limit the adoption of Fe2O3. For example, materials like titanium dioxide (TiO2) or other catalytically active metals may be favored if they offer better performance or lower costs. Therefore, understanding both the economic landscape and the comparative advantages of Fe2O3 is crucial for its application in new technologies.
Overall, the challenges surrounding Iron(III) oxide applications underscore the need for continual research. Addressing material limitations and economic considerations is key for maximizing its potential in various fields.
Future Directions of Fe2O3 Research
Understanding the future directions of Iron(III) oxide (Fe2O3) research is essential. It reflects the ongoing innovation and exploration in this compound's applications and synthesis methods. Fe2O3, known for its versatility, plays a significant role in fields ranging from environmental science to materials engineering. As demands for sustainable and efficient materials grow, the study of Fe2O3 must evolve.
Innovations in Synthesis
Innovative synthesis methods are at the forefront of Fe2O3 research. Traditional methods, such as thermal decomposition and chemical precipitation, have served well. However, new approaches offer enhanced efficiency and environmental benefits. For instance, sol-gel processing allows for tailored properties, achieving uniform particle sizes and higher surface areas. This method is not only cost-effective, but it also minimizes energy consumption.
Another promising technique involves hydrothermal synthesis. This process enables the production of nanostructured Fe2O3 with specific morphologies, potentially improving performance in applications, such as catalysis or solar energy conversion. Additionally, microwave-assisted synthesis is gaining attention. It reduces the time required for Fe2O3 production and often leads to enhanced structural characteristics.
Achieving greener methods of synthesis is also crucial. Researchers are exploring bio-inspired approaches, utilizing organic compounds or waste materials to produce Fe2O3. This aligns with global sustainability efforts and reduces the environmental impact associated with traditional synthesis methods.
Potential New Applications
The potential applications of Fe2O3 are vast and still largely untapped. In the realm of energy storage, Fe2O3 can function as an efficient anode material for lithium-ion batteries. Its electronic properties could lead to higher capacities and longer life cycles. This is essential as the demand for portable energy solutions continues to rise.
In environmental science, researchers are exploring Fe2O3's role in photocatalysis for wastewater treatment. The compound exhibits significant photocatalytic activity under visible light irradiation. This opens pathways to more sustainable ways to treat contaminated water, addressing pressing global challenges.
Moreover, Fe2O3 exhibits promise in the field of gas sensing. Its sensitivity toward various gases can be harnessed for pollution monitoring and industrial safety. With further research and development, Fe2O3-based sensors could enhance air quality management in urban settings.
"The future of Fe2O3 research is promising, with innovations likely to reshape its applications in technology and environmental solutions."
Thus, exploring both the synthesis innovations and new application domains presents an exciting frontier in the utilization of Iron(III) oxide. As researchers delve into these areas, we can expect significant advancements that enhance not only the material's performance but also its role in addressing global challenges.
End
The conclusion of this article serves as a pivotal segment in solidifying the importance of Iron(III) oxide. Throughout the preceding sections, we have examined its molecular properties, methodologies for synthesis, and multifaceted applications across various disciplines. Iron(III) oxide is not merely a compound of academic interest; its implications extend deeply into environmental science, industrial practices, and emerging technology sectors.
Summary of Key Findings
In summary, several key findings emerge from this exploration of Iron(III) oxide:
- Chemical Properties: Iron(III) oxide exhibits unique thermal stability and reactivity patterns, making it suitable for diverse reactions in industrial processes.
- Synthesis Techniques: Various methods for synthesizing Fe2O3, including chemical and sustainable approaches, highlight its potential for innovation in production.
- Applications: The compound finds extensive application in sectors such as catalysis, environmental remediation, and solar energy conversion. Each of these domains benefits from specific properties of Fe2O3, showcasing its versatility.
- Environmental Impact: Understanding the role of Iron(III) oxide in soil chemistry and water quality underscores its significance to environmental studies and bioremediation strategies.
The Broader Impact of Fe2O3
The implications of Iron(III) oxide extend beyond individual applications. The advancement of materials science hinges on compounds like Fe2O3 that can optimize existing technologies and lead to sustainable practices. The integration of Iron(III) oxide in solar energy systems, for instance, reflects a critical step toward green technologies. By facilitating photocatalytic processes, it aids in addressing energy challenges while also contributing to environmental preservation.
Furthermore, in the context of catalysis, the innovative application of Fe2O3 opens new avenues for efficient chemical reactions. This not only advances scientific understanding but also enhances industrial processes, leading to more effective production lines.