Inphase: Understanding Temporal Synchrony in Science
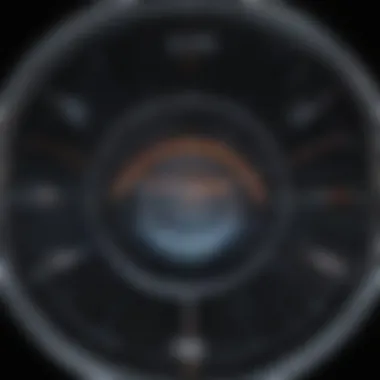
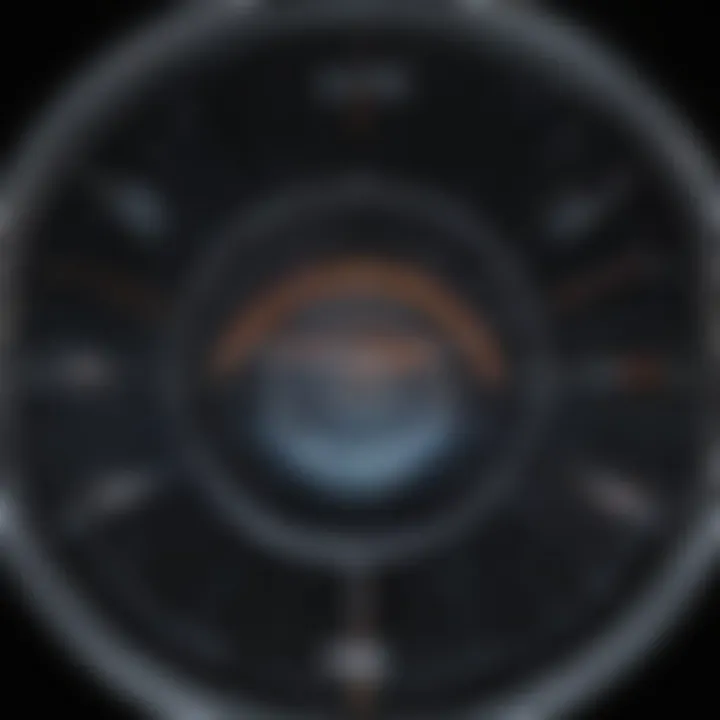
Intro
The concept of inphase is pivotal in examining how various systems synchronize over time. In its essence, temporal synchrony refers to the alignment of rhythmic processes, which can be observed in myriad fields such as biology and physics. Understanding these systems helps to shed light on complex interactions and behaviors found in nature and artificial constructs alike.
As we delve into the intricacies of inphase dynamics, this article explores its significance and implications across several scientific domains. This exploration aims not only to enhance comprehension of the subject but also to encourage further research that can lead to more profound insights.
Methodologies
Description of Research Techniques
The methodologies for studying temporal synchrony typically encompass both experimental approaches and theoretical analysis. In experimental settings, researchers often conduct real-time observations of systems where inphase phenomena are likely to manifest. For example, studying neural oscillations can reveal how brain regions synchronize during cognitive processes or motor functions.
Simulations are also a prominent technique. Researchers model complex systems, utilizing computational tools to emulate conditions favorable for inphase phenomena. By observing outcomes of various simulations, insights can be obtained about the underlying principles that govern synchrony across different contexts.
Tools and Technologies Used
To facilitate these research techniques, practitioners employ a range of tools and technologies. Some commonly used tools include:
- Software for data analysis: Programs like MATLAB and Python libraries (such as SciPy and NumPy) are employed for statistical analysis and modeling.
- Measurement devices: In biological studies, for instance, EEG and fMRI devices measure neural synchrony.
- Simulation platforms: Platforms like NetLogo allow researchers to create agent-based models that illustrate how multiple agents interact over time.
Incorporating these tools allows a more refined understanding of inphase dynamics and helps elucidate the principles that govern temporal synchrony.
Discussion
Comparison with Previous Research
Historically, the exploration of synchrony has evolved, with notable contributions from fields such as physics and biology. Earlier studies predominantly focused on the synchronization of physical systems, like metronomes. Recent investigations, however, extend to biological rhythms and complex networks, offering a broader perspective. Notably, the framework established by scholars like Winfree and Kuramoto has shaped contemporary understanding, paving the way for interdisciplinary applications.
Theoretical Implications
The implications of inphase phenomena extend beyond mere observation; they influence theoretical frameworks. For instance, insights from studies on cardiac rhythms provide a deeper understanding of physiological states and the importance of synchrony in health and disease. The existence of inphase conditions may also contribute to advancing theoretical constructs in chaos theory, highlighting stability and resilience in complex systems.
The exploration of inphase benefits not only a scientific audience but also extends its relevance into practical applications in technology and medicine.
Understanding the complexities of inphase dynamics equips researchers and professionals with the knowledge required to tackle both fundamental and applied challenges. As we continue to investigate this concept, the future research directions may lead to groundbreaking discoveries that further elucidate the significance of temporal synchrony.
Understanding Inphase
The concept of inphase refers to a state where two or more systems operate in synchrony, aligned in their cycles or phases. Understanding inphase is crucial as it presents insights into complex systems across different scientific domains. This exploration helps to elucidate the underlying principles that drive synchronization, shedding light on phenomena ranging from biological to physical systems.
The benefits of grasping the concept of inphase extend well beyond theoretical discussions. It plays an integral role in biological processes, impacting elements such as circadian rhythms and cellular coordination. Furthermore, it contributes to advancements in technology and engineering, particularly through the application of synchrony in communications and robotics. Among its numerous considerations, inphase dynamics raises questions about how best to observe and measure synchronization, bringing to light challenges in data collection and interpretation.
As we proceed, our dialogue will outline key areas merging different fields where inphase phenomena are relevant. This exploration offers not only a deeper understanding but also a framework for future research, pointing toward new technologies and methodologies.
Defining Temporal Synchrony
Temporal synchrony refers to the alignment of periodic processes in time. In a synchronised system, the phases of these periodic processes coincide, leading to coherent behavior among the components involved. It is an essential aspect of various scientific inquiries, including biology, physics, and Earth sciences.
Understanding temporal synchrony empowers researchers to predict how changes in one component of a system can influence others. The concept is particularly relevant when discussing complex biological networks where timing is critical. The ability to define and measure this synchrony can lead to significant advances in areas such as health sciences, where the coordination of cellular activities plays a vital role in maintaining proper function.
Principles of Inphase Dynamics
Inphase dynamics is governed by several core principles that help us understand how systems attain synchronized states. First, the principle of phase locking suggests that oscillators can adjust their rhythms to remain aligned, exhibiting stable synchrony under certain conditions. This process is crucial for understanding systems where timing and coordination are essential.
Second, feedback loops significantly influence inphase dynamics. These loops dictate how one part of a system affects another over time, leading to reinforcement or damping of a specific phase relationship. Such mechanisms can be observed in ecological systems, where various species interact and synchronize their behaviors for survival.
Third, the impact of external forces cannot be underestimated. In many systems, external stimuli induce changes in the phase relationship, leading to either synchrony or desynchronization. Understanding these external influences can greatly enhance our ability to manipulate systems in a controlled manner, whether for scientific research or practical applications.
Inphase in Biology
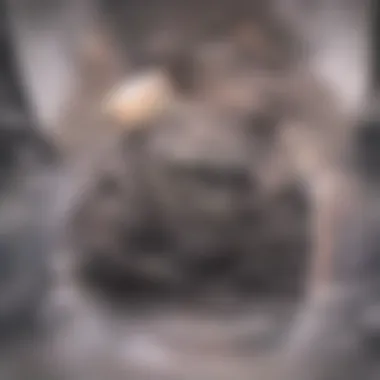
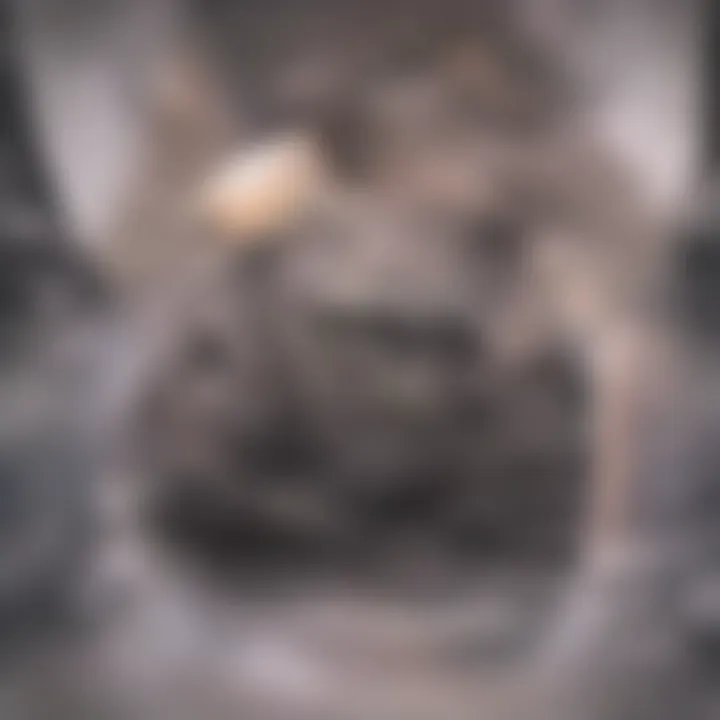
The study of inphase phenomena in biology offers valuable insights into the intricate mechanisms that govern life at various levels. Understanding inphase dynamics enhances comprehension of biological rhythms, cellular operations, and their implications in health and disease. This section explores the two primary areas where inphase plays a critical role: circadian rhythms and cellular synchronization.
Circadian Rhythms
Circadian rhythms represent a key biological process influenced by inphase dynamics. These rhythms follow a roughly 24-hour cycle, regulating various physiological processes such as sleep-wake cycles, hormone release, and body temperature. The synchronization of these cycles to external cues, primarily light and darkness, highlights the importance of inphase behavior in organisms.
Several factors contribute to the maintenance and accuracy of circadian rhythms. Among these, the suprachiasmatic nucleus (SCN) in the brain serves as the master clock, controlling the timing of peripheral clocks in tissues throughout the body. The role of signaling molecules like melatonin and cortisol is critical, as they are involved in the modulation of these rhythms.
"Circadian rhythms are not merely a reflection of environmental changes; they are fundamental to the operational efficiency of biological systems."
Furthermore, disruptions in these rhythms due to irregular sleep patterns or exposure to artificial light can have profound effects on health. Studies indicate that chronic misalignment may lead to increased risks of metabolic disorders, sleep disturbances, and mood disorders. This evidence solidifies the relevance of maintaining synchronicity in circadian systems through lifestyle and environmental adjustments.
Cellular Synchronization
Cellular synchronization refers to the coordinated activity of cells within tissues or organs. This phenomenon allows for efficient functioning, particularly in cardiac and neural systems. When cells are inphase, they respond collectively to stimuli, enabling coordinated contractions in the heart or synchronized signals in the nervous system.
Various mechanisms enable cellular synchronization. Gap junctions play a significant role by allowing ions and small molecules to pass directly between adjacent cells, facilitating rapid communication. Additionally, biochemical signaling pathways help communicate changes and ensure a unified response.
The implications of cellular synchronization extend into medical fields as well. Conditions like arrhythmias in the heart arise from desynchronization, leading to potentially life-threatening consequences. Understanding the principles of inphase dynamics could inform treatment strategies aimed at restoring synchronized activity in affected tissues.
Inphase Phenomena in Physics
Inphase phenomena in physics play a critical role in understanding the behavior of various systems, ranging from the very small scale of particles to the larger scale of mechanical systems. These phenomena are characterized by the synchronous behavior of waves or oscillators, which can lead to constructive interference and enhance the overall system's efficiency and stability. In this section, we will discuss two key aspects of inphase phenomena within physics: wave interference and synchronization in mechanical systems.
Wave Interference
Wave interference occurs when two or more waves traverse the same space simultaneously, leading to a combination of their effects. This is a fundamental concept in physics that illustrates how waves can interact with each other. The two primary types of interference are constructive and destructive:
- Constructive interference happens when waves align in phase, resulting in an increase in amplitude. This phenomenon is often observed in sound waves, light waves, and other types of wave functions.
- Destructive interference is when waves are out of phase and cancel each other out, leading to a reduced amplitude.
Wave interference has several applications in various fields:
- Acoustics: Understanding interference is vital in designing concert halls and sound systems to enhance audio quality.
- Optics: Interference patterns assist in resolving details in optical instruments like microscopes and telescopes.
- Signal Processing: Engineers use interference principles to enhance communication technologies, such as radio waves and internet signals.
Through rigorous studying of wave interference, scientists have developed methods for controlling and utilizing inphase phenomena to optimize performance in these applications.
Synchronization in Mechanical Systems
Synchronization in mechanical systems is another compelling area where inphase dynamics manifest. This involves the coordination of oscillations in mechanical components, often resulting in more efficient performance. For example, consider a set of pendulum clocks. If they are slightly displaced from their equilibrium position, they tend to adjust their movements and synchronize over time.
Some notable points about synchronization in mechanical systems include:
- Enhanced Stability: When components synchronize in phase, the collective stability can be enhanced compared to solitary, derivative actions.
- Energy Efficiency: Synchronous systems often operate with less energy loss, making them desirable in various mechanical designs.
- Applications in Robotics: Coordinated movements of robotic components allow for more sophisticated and effective operation in automated environments.
Overall, understanding synchronization in mechanical systems provides foundational insights into designing advanced technologies, from vehicles to robotics, where the timing and coordination of parts are crucial for effective operation.
"The ability to harness inphase phenomena is a cornerstone of modern innovations in physics and engineering."
By studying inphase phenomena within wave interference and synchronization in mechanical systems, researchers can gain a deeper understanding of the principles governing these interactions, thus enhancing future technological advancements.
Inphase in Earth Sciences
Inphase dynamics play a crucial role in Earth sciences, presenting a framework for understanding natural phenomena through the lens of temporal synchrony. This section highlights the significance of inphase in studying various Earth-related systems, such as tidal patterns and climate cycles. Understanding these elements can provide insights into how different systems interact, and the implications for environmental management and forecasting. The study of inphase mechanics can help explain variations in these systems, revealing underlying processes that affect ecological balances, which is pivotal for researchers and professionals in the field.
Tidal Patterns
Tides are a classic example of inphase dynamics in Earth sciences. They arise primarily due to the gravitational forces exerted by the moon and the sun, creating rhythmic patterns that influence coastal environments. The regular rise and fall of sea levels demonstrate how inphase characteristics govern large-scale oceanic movements.
The importance of understanding tidal patterns cannot be overstated. Regular tidal activity maintains coastal ecosystems, supports navigation, and affects fishing practices. Additionally, these patterns interact with human activity. For instance, coastal constructions must consider tidal changes to minimize environmental impact.
"The synchronization of tidal movements reflects intricate relationships between celestial bodies and Earth sciences."
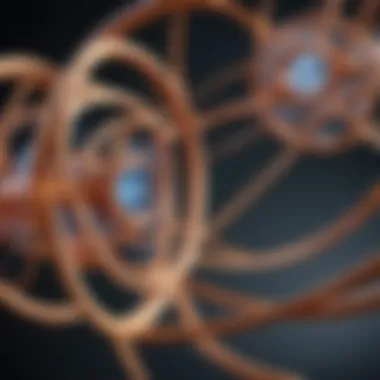
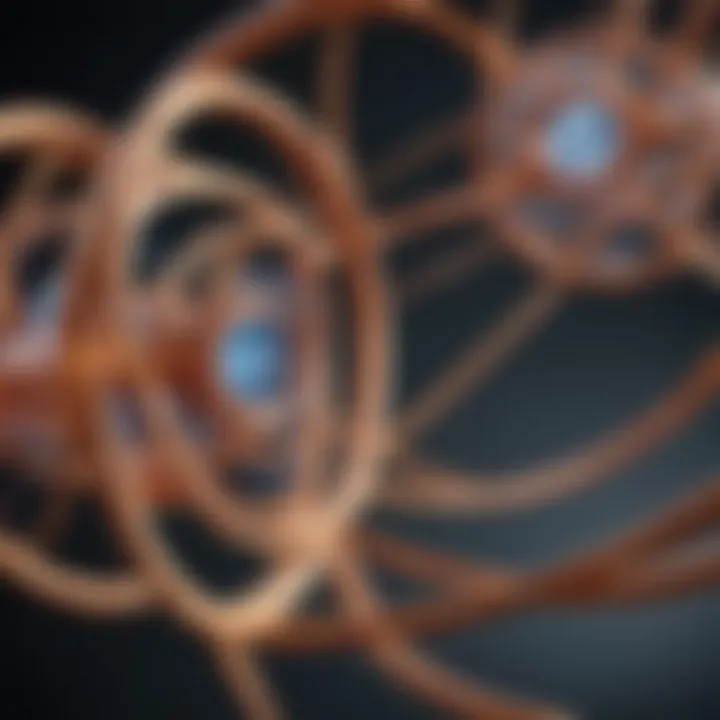
Moreover, researchers utilize mathematical modeling to predict these tidal systems, which aids in managing coastal resources effectively. Tools such as harmonic analysis help in forecasting tide levels by assessing historical data and recognizing lunar cycles.
Climate Cycles and Synchrony
Climate cycles also exemplify inphase dynamics within Earth sciences. Various environmental factors, including atmospheric conditions, ocean currents, and solar radiation, interact to create predictable climate patterns. These cycles can span from short-term weather changes to long-term phenomena, such as ice ages.
Understanding climate synchrony allows scientists to discern patterns and anomalies within climate data. For example, the El NiΓ±o and La NiΓ±a phenomena showcase how disruptions in oceanic temperatures can lead to global climatic shifts. Recognizing these connections is key for predicting weather extremes and preparing for climate-related disasters.
In addition to natural cycles, human influence complicates these synchrony patterns. Anthropogenic factors such as pollution and greenhouse gas emissions can disrupt established cycles, thus impacting ecosystems and weather. This highlights the need for a nuanced understanding of inphase mechanisms when studying climate change and developing effective responses.
Mathematical Modelling of Inphase Systems
Mathematical modeling of inphase systems provides a critical framework for understanding how various elements interact synchronously over time. This capability is fundamental across disciplines, including biology, physics, and engineering. By applying mathematical concepts, researchers can predict behavior, analyze stability, and explore complex dynamical systems. The insights gained from these models can lead to practical applications that affect everyday life, from developing better communication technologies to improving medical devices.
Differential Equations
Differential equations play a pivotal role in the mathematical modeling of inphase systems. They describe how a quantity changes in relation to another, capturing the essence of dynamics involved in synchronized systems. For instance, consider oscillators that exhibit inphase behavior. The interaction between different components can be represented through coupled differential equations. These equations enable researchers to explore stability, convergence, and other critical phenomena relevant in various scientific fields.
The general form can be represented as:
[ \fracdxdt = f(x, y) ]
Where (x) and (y) are dependent variables representing the systems under consideration, and (f) is a function that describes their interaction over time. By solving these equations, one may determine conditions that lead to synchronization, quantify the response to perturbations, or assess the effects of external influences on oscillatory systems.
Chaos Theory and Synchrony
Chaos theory presents an intriguing dimension when studying inphase systems. While synchrony asserts that systems behave in a predictable and organized manner, chaos introduces complexity and unpredictability. In such contexts, even small perturbations can lead to drastically different outcomes. This sensitivity makes chaos theory essential for understanding the boundaries of synchronization.
For inphase conditions, chaos can initially appear as a disruption. However, it often underlines the fragility of synchronized states. The use of mathematical tools allows researchers to delineate the thresholds where systems transition from coherent behavior to chaotic states. These insights have profound implications for various applications ranging from climate predictions to neural networks.
In summary, mathematical modeling via differential equations and an understanding of chaos theory enrich the study of inphase systems. Together, they contribute to a more nuanced comprehension of synchronization, aiding both theoretical and practical advancements in numerous domains.
Applications of Inphase Concepts
Understanding the applications of inphase concepts is essential for grasping its practical implications across various sectors. The significance of these applications lies in their ability to enhance efficiency, optimize performance, and foster innovations in technology and medicine. The concept of being in phase helps in cultivating synchronization, which can be crucial, particularly in dynamic systems. By analyzing specific examples, we can appreciate how inphase principles improve functionalities in daily life and advanced scientific endeavors.
Communications Technology
In communications, the principle of inphase plays a vital role. Synchronization ensures that data transmission occurs seamlessly, reducing errors and increasing bandwidth efficiency. Digital communication systems often rely on phase synchronization to achieve precise data integrity.
For instance, technologies like 4G and 5G networks utilize inphase signals to ensure that mobile communications are fast and reliable. These networks employ multiple-input and multiple-output (MIMO) technology, which can only function properly if the signals are synchronized.
The influence of inphase principles can also be seen in satellite communications. Here, the synchronization of signals is crucial for accurate positioning and data transfer. Failure to maintain inphase synchrony can result in data loss or decreased communication quality.
Key benefits of implementing inphase concepts in communications include:
- Increased data transfer rates: Enhanced synchronization allows for higher data throughput.
- Improved connectivity: Minimization of latency issues leads to better user experience.
- Reduced interference: Helps in mitigating issues caused by overlapping signals.
Medical Applications
In the field of medicine, the relevance of inphase concepts extends to various diagnostic and therapeutic techniques. One area where this is especially critical is in medical imaging. Techniques like Magnetic Resonance Imaging (MRI) work on the principles of phase synchronization. Accurate phase alignment of radiofrequency signals is vital for high-resolution images, helping to guide healthcare decisions effectively.
Moreover, the synchronization of biological signals is significant in physiological studies. Heart rhythm monitoring relies on detecting inphase signals to assess cardiac health accurately. Devices like Holter monitors and pacemakers are designed to ensure that the electrical signals in the heart operate in a synchronized manner. Analyzing these signals can lead to early detection of arrhythmias, thereby reducing the risk of severe health issues.
Aspects of inphase applications in medicine include:
- Enhanced diagnostic capabilities: Early detection of health problems through synchronized monitoring of vital signs.
- Improved treatment outcomes: Synchronization can lead to better patient compliance and therapeutic efficacy.
- Data integration in healthcare: Helps in combining patient data for comprehensive treatment plans.
"The coordination of signals in both communication and medical fields illustrates the broad relevance of inphase principles, shaping the evolution of technology and healthcare."
In summary, applying inphase concepts across communications technology and medical applications indicates a substantial impact on efficacy and innovation, highlighting the need for further research and development in these areas.
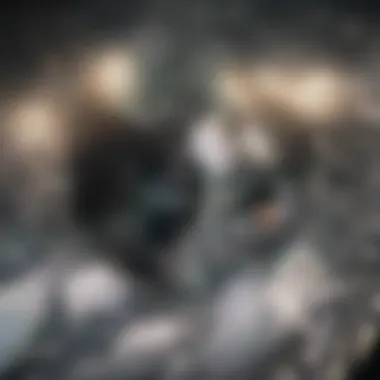
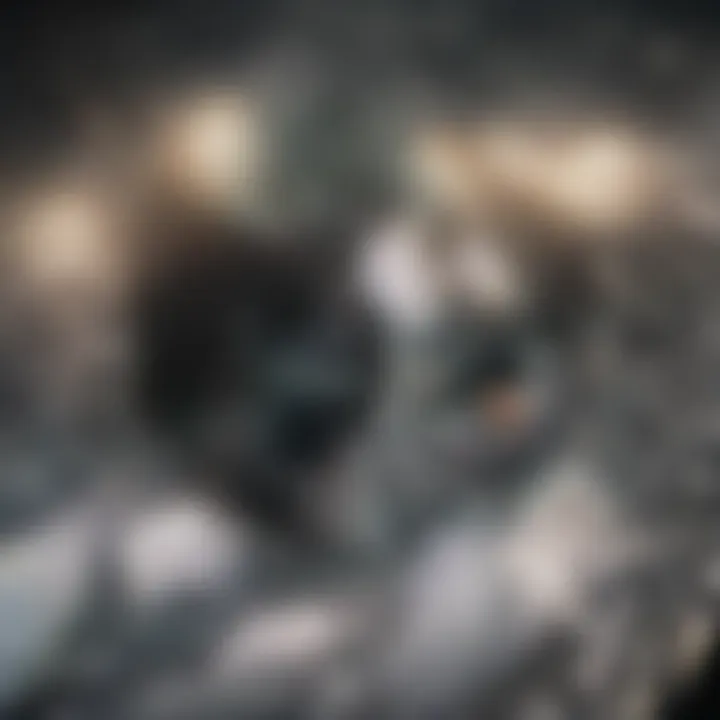
Challenges in Studying Inphase Dynamics
The study of inphase dynamics presents significant challenges that can impact research methodology and outcomes. Understanding these challenges is crucial as they inform both the design of experiments and the interpretation of results in various fields. Furthermore, overcoming these obstacles can lead to meaningful advancements in applications of temporal synchrony across different scientific domains.
Measurement Difficulties
Accurately measuring inphase dynamics can be a complex task. One major issue lies in the precision of timing measurements. For instance, in biological research, synchronizing circadian rhythms among different organisms requires precise timekeeping mechanisms. Typical measuring tools often fall short, leading to potential errors in data interpretation. Additionally, variability in biological systems can skew results, making it hard to establish consistent patterns of synchronization.
Another aspect is the scale at which measurements occur. For example, in biological studies, researchers may need to observe cells or organisms in real-time to assess their inphase states. However, many current technologies, such as traditional imaging techniques, have limitations regarding temporal resolution. This means that small but significant fluctuations can be lost, obscuring our understanding of the dynamics involved.
"The reliability of measurements is directly correlated with the validity of our conclusions about inphase phenomena."
Data Collection Issues
Data collection methods also face considerable challenges. Firstly, the complexity of the systems being observed often results in large volumes of data that require sophisticated analysis. Researchers need to ensure that data collection instruments are not only sensitive but also capable of capturing relevant information over appropriate time intervals.
Moreover, ensuring data integrity is paramount. Inphase dynamics can be influenced by external factors that may introduce noise into the data or lead to misinterpretations. For example, environmental conditions such as temperature and light can affect biological rhythms, and researchers must account for these variables when collecting data.
To address these challenges, interdisciplinary approaches integrating technology, biology, and analytical methods may offer potential solutions. Ultimately, overcoming data collection issues is essential for advancing our understanding of inphase synchronization and its implications across various fields.
Future Directions in Inphase Research
In the dynamic landscape of scientific inquiry, the exploration of inphase dynamics is not merely an academic exercise; it holds profound implications across multiple disciplines. Understanding the future directions in inphase research is crucial as it provides insights into evolving technologies and methodologies that can enhance our grasp of temporal synchrony. This section discusses the significant elements of emerging technologies and interdisciplinary approaches that will shape the future of this field.
Emerging Technologies
The advancement of technology continues to impact research in inphase phenomena. New tools provide the ability to observe and measure synchrony at scales previously deemed impossible. For instance, developments in quantum computing and machine learning algorithms are pivotal in analyzing complex data patterns within inphase systems.
In particular, quantum sensors are measuring precision that can lead to breakthroughs in understanding synchronization in natural systems. These technologies can bridge gaps in current methodologies that rely heavily on classical mechanics.
Additionally, advancements in biotechnology, such as CRISPR and synthetic biology, present opportunities to manipulate biological rhythms with precision. This can lead to new insights in areas like circadian biology and cellular synchronization. Future research utilizing these technologies might address questions that remain unanswered due to limitations in current methodologies.
"The integration of emerging technologies in studying inphase phenomena is essential to unlock potentials in various scientific domains."
Interdisciplinary Approaches
As challenges in studying inphase dynamics often stem from overly narrow focus within disciplines, adopting interdisciplinary approaches is vital for future research. Areas like neuroscience, climatology, and computer science can benefit significantly from incorporating concepts of temporal synchrony. By fostering collaboration among researchers from diverse fields, new frameworks can emerge that allow for holistic understanding and innovative solutions.
For example, the study of brain synchrony in neuroscience could uncover fundamental mechanisms behind cognition and behavior. Additionally, integrating climate science with synchrony principles may offer deeper insights into climate change patterns and cycles.
Potential interdisciplinary initiatives could include:
- Collaborative models linking data from biological and environmental sciences.
- Exploring the impact of human activity on natural synchrony cycles.
- Utilizing computational techniques to simulate inphase phenomena across different domains.
In summary, pursuing future directions in inphase research offers invaluable opportunities not only for enhancing scientific knowledge but also for addressing real-world issues through innovative applications. Emphasizing emerging technologies and encouraging interdisciplinary collaboration stands to significantly advance the field.
Epilogue
The conclusion plays a pivotal role in encapsulating the discussions surrounding inphase phenomena. This section synthesizes the key insights gained throughout the article and highlights their importance across diverse scientific fields. By reflecting on the findings related to temporal synchrony, readers can appreciate the interconnectedness of the various applications and theories presented.
In the summary of key insights, major themes such as the principles of inphase dynamics, biological rhythms, and applications in various technologies are revisited. Each element serves to reinforce not only the relevance of inphase concepts in contemporary research but also their practical implications in real-world contexts. The significance of studying inphase dynamics extends beyond academic interest; it has tangible benefits in fields such as medicine, environmental science, and engineering.
Understanding inphase offers a clearer picture of synchronization patterns in nature and human-made systems. This knowledge can lead to innovations and improvements, particularly in technologies that require precise timing and coordination. For scholars and practitioners alike, being versed in these concepts is essential.
Summary of Key Insights
- Temporal Synchrony is fundamental in understanding the interaction between different systems, from cells to celestial bodies.
- Biological Applications illustrate how circadian rhythms and cellular behaviors are influenced by inphase dynamics.
- Physical Phenomena include wave interference, showcasing the principles of synchronization in physics.
- Earth Sciences detail the relevance of tidal patterns and climate cycles in ecological synchrony.
- Mathematical Models provide a framework for predicting and analyzing inphase behaviors.
- Practical Applications span communications and medical technologies, revealing the benefits of grasping inphase concepts.
The diverse applications and implications of inphase dynamics merit further exploration. Scholars and practitioners may draw from the findings to push the boundaries within their own fields.
Final Thoughts
In summary, the exploration of inphase phenomena inspires future inquiries and encourages interdisciplinary collaboration. The challenges identified in studying inphase dynamics are significant but not insurmountable. They offer avenues for research advancement and technological innovation. As our understanding deepens, so too does our potential to harness the power of synchronization in both natural and engineered systems.
"The quest for knowledge about inphase phenomena reflects a broader desire to understand the world around us."
Through this detailed exploration, we encourage ongoing research, emphasizing that the fullness of understanding temporal synchrony is still a work in progress, ripe for further investigation.