A Comprehensive Guide to Genes and Their Role
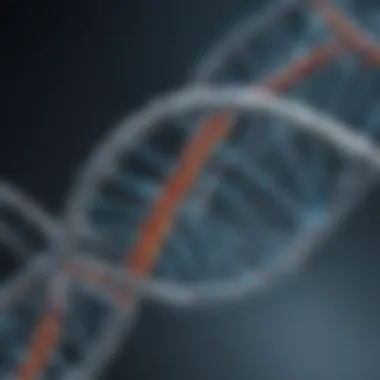
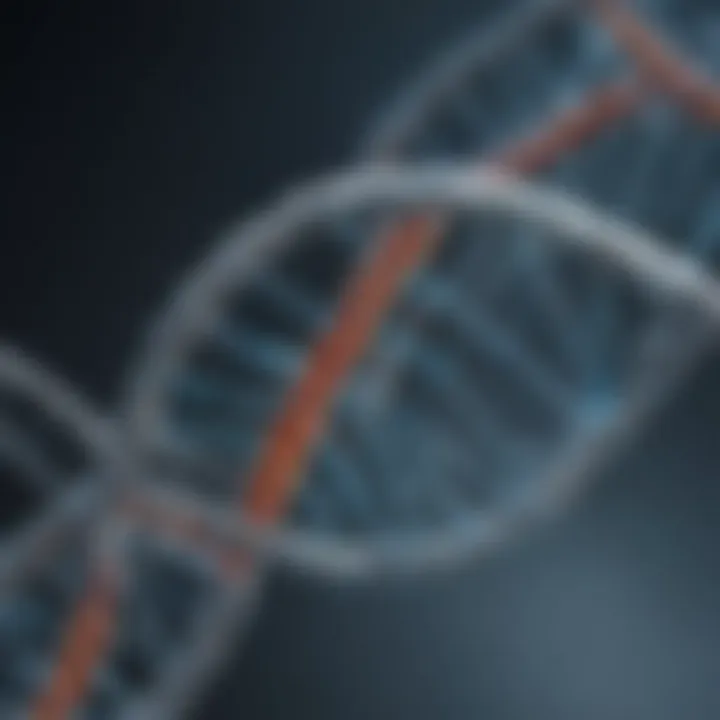
Intro
Understanding genes is essential for a wide array of scientific disciplines. Genes are the fundamental units of heredity and play a vital role in the functioning of all living organisms. They encode information necessary for the development, functioning, growth, and reproduction of life forms. This guide aims to provide a clear and detailed overview of the structure and function of genes, exploring various facets of their role in health, disease, and evolutionary biology.
In this guide, we will discuss the different methodologies used in genetic research, the tools and technologies that are advancing our knowledge, and the implications of recent findings in this complex field.
Methodologies
Description of Research Techniques
Genetic research employs numerous methodologies to unravel the complexities of genetic information. One of the primary techniques is polymerase chain reaction (PCR), which amplifies small segments of DNA to study genetic variations and mutations. This method has revolutionized molecular biology by enabling rapid analysis of genetic material.
Another vital technique is gene sequencing, which identifies the precise order of nucleotides in a DNA molecule. This information is crucial for understanding genetic disorders and evolutionary relationships among species. Techniques such as next-generation sequencing (NGS) facilitate high-throughput sequencing, allowing for the exploration of entire genomes.
Tools and Technologies Used
The field of genetics has been significantly enhanced by various tools and technologies. Bioinformatics software is one of the most critical resources for analyzing complex datasets generated through genetic research. Tools like BLAST and GenBank allow researchers to compare genes across different species and understand their functions better.
In addition, CRISPR-Cas9 has emerged as a groundbreaking technology for gene editing. This tool enables precise modifications to the genome, opening up possibilities for correcting genetic defects and advancing therapeutics for various diseases. The role of DNA microarrays also cannot be overlooked, as they allow researchers to analyze the expression of thousands of genes simultaneously, providing insights into cellular functions.
"Genes are not just instructions but active participants in the narrative of life, shaping the biological and evolutionary story of every organism."
Discussion
Comparison with Previous Research
Significant advances have been made in understanding genes compared to earlier research. Historically, genetics focused on inheritance patterns and phenotypic expressions. Contemporary research, however, investigates the intricate interactions between genes and environmental factors, emphasizing epigenetics. Epigenetic research explores how external influences can alter gene expression without changing the underlying DNA sequence, contributing to fields such as developmental biology and cancer research.
Theoretical Implications
The findings in genetic research have profound theoretical implications, particularly concerning evolutionary biology. Understanding the genetic basis for variation within and between species enhances the comprehension of natural selection and speciation. These insights contribute to the ongoing dialogue about genetic diversity and its significance in adapting to changing environments. Moreover, the ethical implications of genetic research prompt discussions about responsibility and the potential consequences of gene editing technologies.
By navigating the depths of genetic research, this guide enables students, researchers, educators, and professionals to grasp essential concepts and contemporary issues in genetics. Through dissecting methodologies and discussing theoretical implications, a clearer picture of the role genes play in life and health emerges. This understanding not only enriches scientific discourse but also fosters appreciation for the intricacies of genetic science.
Foreword to Genes
Genes are fundamental units of heredity that play a critical role in defining who we are as living organisms. Understanding genes extends beyond mere scientific curiosity; it has profound implications on fields such as medicine, biotechnology, and evolutionary biology. This section introduces the concept of genes, emphasizing their importance in various biological processes.
Defining Genes
In the simplest terms, a gene is a segment of DNA that contains the information necessary to produce proteins or RNA molecules. Genes dictate everything from physical characteristics to biochemical processes vital for survival. Each gene resides on chromosomes, which are organized structures that house an organism's genetic material.
Genes are composed of sequences of nucleotides, which are the building blocks of DNA. These sequences guide the synthesis of proteins, which in turn govern cellular functions. They are therefore central to multiple aspects of life, including development, metabolism, and response to environmental stimuli.
To fully grasp the concept of genes, it’s essential to recognize their variability. Genetic variations, or mutations, can lead to differences in traits, which can affect an individual's health and functionality.
The Historical Context of Genetic Research
The study of genetics has evolved significantly over the past century. Early explorations began with Gregor Mendel's work in the 19th century, where he established foundational principles of inheritance through his experiments with pea plants. Mendel’s findings introduced the idea that traits are passed from parents to offspring in predictable patterns, laying the groundwork for modern genetics.
With the discovery of the structure of DNA by James Watson and Francis Crick in 1953, the field entered a new era. This pivotal moment not only elucidated how genetic information is stored but also sparked decades of research focused on gene sequencing and manipulation.
Today, advancements such as the Human Genome Project, completed in 2003, have significantly enhanced our understanding of the human genome, exposing the intricate relationship between genes and health. The insights gained from this research continue to inform genetic counseling and precision medicine, illustrating the real-world applications of genetic knowledge.
Understanding the history of genetic research is vital as it contextualizes current scientific endeavors. By tracing back the origins, we can better appreciate the complexities surrounding gene function and its implications in contemporary science.
"The study of genes is a journey through time, revealing the intricacies that define both individuality and commonality in all living forms."
The exploration presented in this section sets the stage for further discussions on gene structure, function, and their crucial roles within biological systems.
The Structure of Genes
The structure of genes is a cornerstone of understanding genetics and provides insight into the complexities of biological systems. Genes are the functional units of heredity, and their organization informs everything from basic cellular functions to the development of complex organisms. By examining the structural elements of genes, we can appreciate how they contribute to genetic diversity, and how alterations in these structures can lead to variations in traits.
DNA and RNA: The Genetic Blueprint
Deoxyribonucleic acid (DNA) is often called the genetic blueprint of life. It is a double-helix structure composed of nucleotides, which contain a phosphate group, a sugar, and a nitrogen base. The sequence of these nucleotide bases—adenine, thymine, cytosine, and guanine—encodes the information needed to build proteins and regulate cellular activities.
RNA, or ribonucleic acid, plays a critical role in translating this genetic information into action. Unlike DNA, RNA is typically single-stranded and uses uracil instead of thymine. There are various forms of RNA, each with distinct functions:
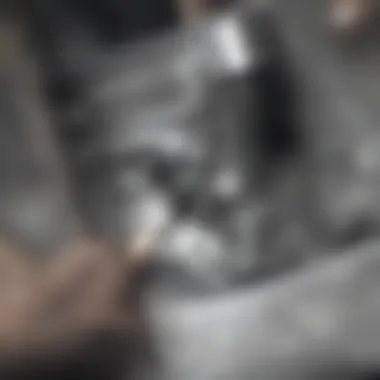
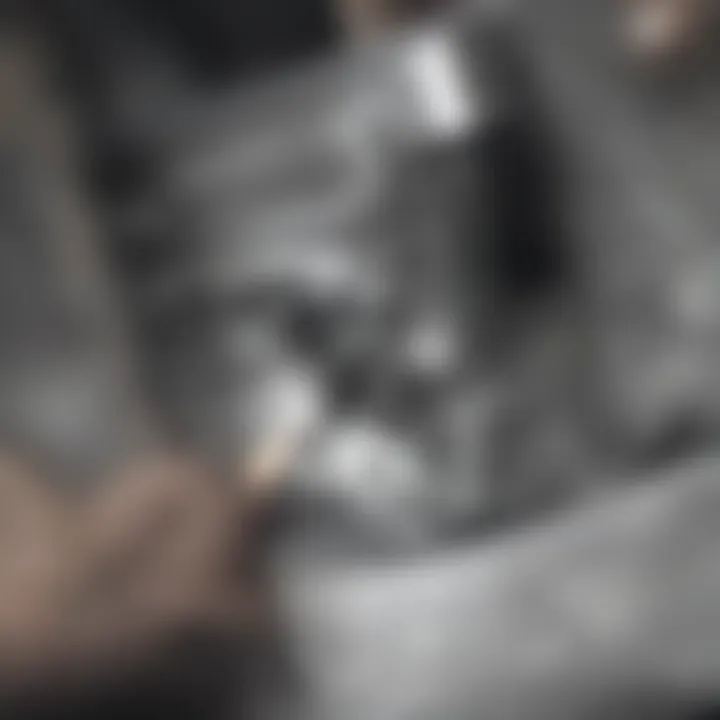
- Messenger RNA (mRNA): Carries the genetic code from DNA to the ribosome, where proteins are synthesized.
- Transfer RNA (tRNA): Functions as the adaptor that translates the mRNA code into amino acids, the building blocks of proteins.
- Ribosomal RNA (rRNA): A crucial component of ribosomes, which assemble proteins.
Understanding the roles of DNA and RNA helps clarify how genetic information flows in living organisms. The transcription of DNA into mRNA, and subsequently the translation into proteins, is essential for cellular function and overall organismal development.
Chromosomes: Organization of Genetic Material
Chromosomes are structures within cells that organize and package DNA. In humans, 46 chromosomes are present in pairs, with 23 inherited from each parent. These chromosomes house genes, and their structure plays a vital role in ensuring proper gene expression, DNA replication, and cell division.
Chromosomes can be classified into two main types:
- Autosomes: Non-sex chromosomes that are universal across genders.
- Sex chromosomes: Determine an individual's sex—XX for females and XY for males.
Each chromosome consists of a centromere, a constricted region that divides it into two arms. The structure is further supported by proteins called histones, which help in condensing DNA into a compact shape. Effective organization within these chromosomes is critical for the correct distribution of genetic material during cell division.
"The orderly structure of chromosomes not only preserves genetic integrity but also facilitates the dynamic processes of life that depend on precise gene regulation."
In summary, the structural aspects of genes, including the complexity of DNA and RNA, as well as the organization of chromosomes, are fundamental to our understanding of heredity and genetic function. These structures form the basis for the dynamic interplay between genetics and biology, highlighting their importance in the study of life itself.
Gene Function and Expression
Understanding gene function and expression is crucial to grasping the fundamental principles of biology. Genes, which are segments of DNA, serve as templates for producing proteins and play vital roles in numerous cellular processes. The mechanisms that dictate how and when genes are expressed can significantly affect an organism's phenotype and adaptability. This section will explore the intricacies of transcription and translation, highlighting their importance in gene expression, and examine the regulatory elements that modulate this complex process.
Transcription and Translation: Gene Expression Process
Transcription is the first step in the gene expression process. During transcription, a specific segment of DNA is copied into messenger RNA (mRNA) by the enzyme RNA polymerase. This process begins when RNA polymerase binds to a promoter region of a gene, unwinding the DNA strand and synthesizing the mRNA based on the DNA template. The resulting mRNA strand is a complementary copy of the gene, with uracil replacing thymine.
After transcription, the mRNA undergoes several modifications before it can be translated into a protein. These modifications include splicing, where introns are removed and exons are joined together, and the addition of a 5' cap and a poly-A tail to protect the mRNA from degradation. Once processed, the mRNA exits the nucleus and enters the cytoplasm, where it encounters ribosomes.
Translation is the next step, where the ribosome reads the mRNA sequence and translates it into a polypeptide chain, which later folds into a functional protein. This process involves transfer RNA (tRNA) that brings the correct amino acids to the ribosome, matching them with the corresponding codons on the mRNA. The ribosome moves along the mRNA, facilitating the formation of peptide bonds between amino acids. This intricate process underlines how genes, through transcription and translation, ultimately dictate the synthesis of proteins that perform various functions in an organism.
Regulatory Elements: Enhancers and Promoters
Gene expression is not a straightforward process; it is finely regulated by various elements that control when, where, and how much a gene is expressed. Enhancers and promoters are key regulatory components that play a significant role in this process.
A promoter is a specific DNA sequence located near the start of a gene. It is essential for the initiation of transcription. RNA polymerase binds to the promoter region, which is associated with various transcription factors that help to regulate the transcription process. The presence of such factors can either enhance or suppress gene activity, providing a level of control over gene expression.
Enhancers, on the other hand, are distant elements that can increase the likelihood of transcription. They can be located thousands of nucleotides away from the gene they regulate. By looping the DNA, enhancers can interact with the promoter region, forming a complex that enhances the transcription process. This interaction is crucial because it allows for a nuanced response to environmental signals and cellular needs. Moreover, the interplay between promoters, enhancers, and various other regulatory sequences underlines the adaptability of organisms in response to internal and external changes.
"The regulation of gene expression is complex and reflects the intricate balance of promoting and suppressing factors that govern how our genes function in a dynamic environment."
In summary, the gene function and expression mechanisms—including transcription, translation, and regulatory elements—play an essential role in biological systems. By understanding these processes, researchers can glean insights into genetics, evolution, and potential therapeutic interventions for genetic disorders. The depth of regulation and expression not only determines how life forms develop but also how they adapt to changing environments.
Genetics and Heredity
Genetics and heredity are foundational concepts in biology, essential for understanding how traits are passed from one generation to the next. The importance of these topics cannot be overstated, as they provide insight into the mechanisms of inherited characteristics and their implications for evolution, biology, and medicine. This section will explore the fundamental principles of genetics, focusing on aspects that influence individuality, disease susceptibility, and biodiversity.
Understanding genetics enriches our comprehension of biological diversity and evolutionary processes. Each organism has a unique genetic make-up, formed through a combination of parental traits and environmental interactions. This genetic individuality is essential, not only for an organism's survival but also for the adaptability of species over time. Genetic variation, therefore, plays a crucial role in evolution, enabling populations to adapt to changing environments.
Moreover, genetics is vital in the context of health and disease. Many disorders have a genetic basis, and understanding heredity aids in identifying predispositions to conditions like cystic fibrosis or sickle cell anemia. Clinicians can better address these health issues through genetic counseling when they consider family history and inheritance patterns. This helps in making informed decisions based on an individual's genetic risks.
In summary, genetics and heredity offer insights not just into personal health but also into broader biological principles, making them indispensable in various fields such as medicine, anthropology, and conservation biology.
Mendelian Genetics: Principles of Inheritance
Mendelian genetics, established by Gregor Mendel in the 19th century, provides a framework for understanding the basic principles of inheritance. Mendel's experiments with pea plants led to the formulation of several key laws that continue to underpin genetic studies today. These include the Law of Segregation and the Law of Independent Assortment.
- Law of Segregation: This law states that during gamete formation, the alleles for a trait segregate from each other. Thus, offspring inherit one allele from each parent, defining traits through dominant and recessive variations.
- Law of Independent Assortment: This principle asserts that alleles for different traits segregate independently during gamete formation, leading to a variety of combinations in offspring.
Mendel's work laid the groundwork for classical genetics, demonstrating that traits are inherited in predictable ways. His use of pea plants allowed him to deduce patterns of inheritance, which are applicable across many organisms.
Non-Mendelian Inheritance: Complex Patterns
Non-Mendelian inheritance encompasses various inheritance patterns that do not follow Mendel's laws. This allows a more nuanced understanding of heredity, as genetics can be influenced by multiple factors. Examples of non-Mendelian inheritance include:
- Incomplete Dominance: In this pattern, neither allele is completely dominant, resulting in a heterozygous phenotype that is intermediate between the two homozygous phenotypes. An example is the flower color in snapdragons, where crossing a red flower with a white flower produces pink flowers.
- Codominance: Here, both alleles express themselves fully in the phenotype of the organism. A classic example is in blood types, where individuals with type AB blood display features of both A and B alleles.
- Polygenic Inheritance: Many traits are influenced by multiple genes, leading to a range of phenotypes. Human height, for example, is determined by several genes, highlighting the complexity of inheritance.
- Epigenetics: This field studies how environmental factors can affect gene expression without altering the underlying DNA sequence. Factors such as nutrition, stress, and exposure to toxins can lead to epigenetic changes that may influence traits across generations.
Non-Mendelian inheritance reveals the intricate nature of genetics, showing that the interplay between genes and external factors results in complex patterns of heredity that go beyond simple dominant and recessive traits. Understanding these patterns is essential for comprehending the full spectrum of genetic influences on evolution, health, and behavior.
"Genetics is not only about how we resemble our parents but also how we come to be who we are through an intricate dance of heritage and environment."
Understanding both Mendelian and non-Mendelian genetics equips researchers, healthcare professionals, and students to analyze genetic data and pursue advancements that could change the way we approach genetic disorders, healthcare, and evolutionary biology.
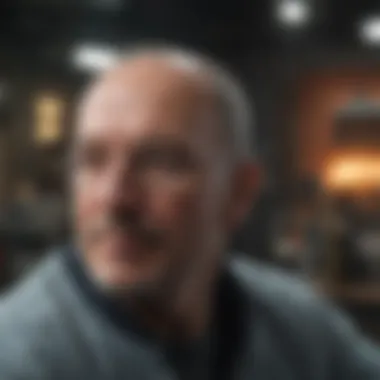
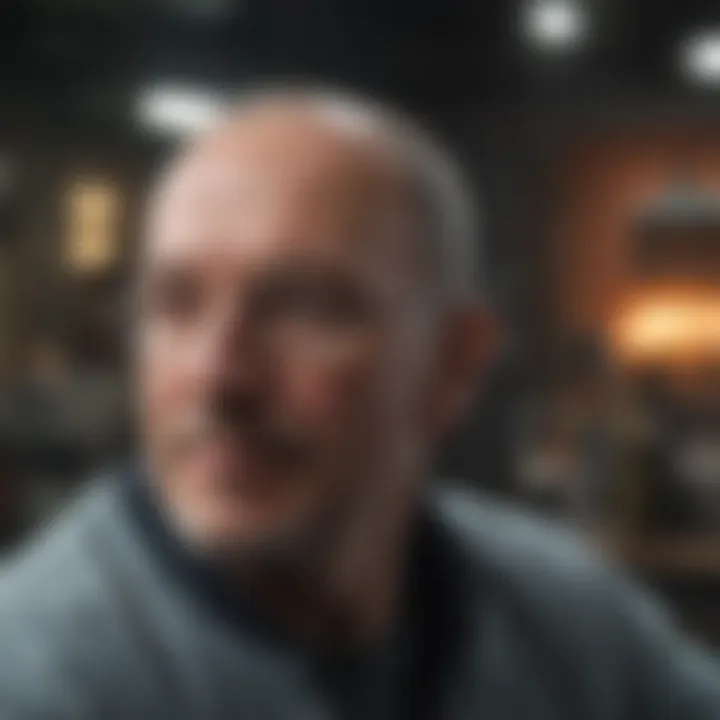
The Role of Genes in Evolution
Understanding the role of genes in evolution is essential for grasping how species adapt and change over time. Genes are the fundamental units of heredity and serve as blueprints for the traits described in organisms. Evolution is the process by which these traits are modified across generations, influenced by genetic variation and environmental factors.
By studying the mechanisms underlying genetic change, one can see how evolution does not merely involve random changes, but rather a complex interaction of genes, natural pressures, and environmental contexts. This understanding illuminates the pathways through which species evolve and diversify.
Natural Selection and Genetic Variation
Natural selection is a key mechanism of evolution, operating on the premise that individuals with advantageous traits have a greater chance of surviving and reproducing. Genetic variation, arising from mutations, recombination, and other forms of genetic change, is what fuels natural selection. Without this variation, evolution cannot occur.
- Adaptation: Beneficial genetic variations leads to adaptations, which improve an organism’s chances in its environment. For example, the peppered moth became darker during the industrial era in England, as darker moths were less visible to predators against soot-darkened trees.
- Survival: Individuals with traits that confer survival advantages continue to pass those genes to their offspring, gradually altering the frequency of these traits in a population over generations.
The study of genetics and evolution has shown that the capacity for change is built into the genetic landscape of every organism. The interplay between environmental pressures and genetic diversity creates a continuous loop that drives evolution forward.
Gene Flow and Genetic Drift
Gene flow refers to the transfer of genetic material between populations. This can occur when organisms migrate and interbreed with members of another population, leading to changes in allele frequencies in both groups. Gene flow can introduce new alleles into a population, increasing genetic diversity.
- Impact on Evolution: Increased genetic diversity often enhances a population's ability to adapt to changing environments. For example, a bee population experiencing gene flow from a neighboring more resilient population may improve its overall robustness against diseases.
In contrast, genetic drift is a mechanism that causes random changes in allele frequencies, especially in small populations. Events such as natural disasters can eliminate individuals regardless of their genetic value, leading to reduced variation and potentially limiting a population's adaptability.
- Bottleneck Effect: This occurs when a population's size is dramatically reduced, leading to a loss of genetic diversity. For instance, the Northern elephant seal was hunted to near extinction. Now, despite its recovery, its genetic diversity is significantly lower than that of its ancestors, impacting its long-term adaptability.
"Genetic drift can play a decisive role in shaping the evolutionary outcomes of populations, particularly in small, isolated groups where chance events can drastically alter genetic composition."
In summary, genes are the threads through which the fabric of evolution is woven. Natural selection, gene flow, and genetic drift all contribute to the dynamic process of evolution, guiding the development of biodiversity. Understanding these processes is crucial for both the study of genetics and the implications for conservation and health in present-day ecosystems.
Emerging Technologies in Genetics
Emerging technologies in genetics represent a significant frontier in molecular biology and biotechnology. They transform our understanding of genetics, offering solutions to complex problems in health care, agriculture, and environmental science. The advancements in genetic research do not only enhance our ability to edit genes but also improve how we analyze and interpret genetic information. This section will explore two pivotal technologies: CRISPR and gene editing as well as genomic sequencing technologies, emphasizing their underlying principles, benefits, and implications.
CRISPR and Gene Editing
CRISPR, an abbreviation for Clustered Regularly Interspaced Short Palindromic Repeats, has revolutionized the field of genetics. It functions as a precise tool for gene editing, allowing scientists to modify DNA at specific locations easily. This technology employs a guide RNA that is designed to match a specific sequence of DNA. The associated enzyme, Cas9, acts as a molecular scissors that cuts the DNA at the targeted site.
The importance of CRISPR lies in its versatility and efficiency compared to previous gene-editing methods. The implications of this technology are profound:
- Therapeutic Potential: CRISPR has opened avenues for treatments of genetic disorders such as cystic fibrosis and sickle cell anemia. Researchers are investigating ways to correct mutations directly in patient cells.
- Agricultural Advances: Scientists are using CRISPR to enhance crop resilience to diseases and environmental stress, potentially leading to a new era of sustainable farming.
- Research Applications: It serves as an invaluable tool for fundamental research, allowing for functional studies of genes by creating knockout organisms.
Still, these benefits come with ethical considerations. Issues around off-target effects, where unintended genes may be altered, as well as the implications of germline editing, raise significant discussions within scientific and public circles.
Genomic Sequencing Technologies
Genomic sequencing technologies are another crucial component of modern genetics. They allow for the determination of the complete DNA sequence of an organism's genome. The insights provided by genomic sequencing hold the key to understanding the complexity of genetic information. Techniques like Sanger sequencing and Next-Generation Sequencing (NGS) have expanded our capacity to analyze genomes comprehensively.
The significance of genomic sequencing includes:
- Personalized Medicine: By analyzing an individual's genetic makeup, healthcare providers can tailor treatments specific to the genetic mutations present in a patient, increasing the effectiveness of therapies.
- Disease Diagnosis: Genomic sequencing plays a crucial role in diagnosing diseases, including cancers, where the identification of specific genetic alterations can inform treatment plans.
- Evolutionary Studies: The technology enhances our ability to study evolutionary relationships among species, providing clarity on genetic variation across populations.
Genetic Disorders and Health Implications
Genetic disorders are conditions caused by abnormalities in genes or chromosomes. Understanding these disorders is essential as they have significant implications for health and disease management. They can impact various aspects of personal well-being and health care strategies. By studying these genetic disorders, researchers can gain insights into the biological mechanisms of diseases and identify potential interventions. Moreover, awareness of genetic disorders aids individuals in making informed health decisions and understanding the risks associated with inherited conditions.
In this section, we will delve into two critical aspects: the types of genetic disorders and the role of genetics in disease susceptibility.
Types of Genetic Disorders
Genetic disorders are categorized based on their underlying causes, genetic inheritance, and manifestations. Below are key types of genetic disorders:
- Single-gene Disorders: These are caused by mutations in a single gene. Examples include Cystic Fibrosis and Sickle Cell Anemia. These disorders follow predictable patterns of inheritance, such as autosomal dominant or recessive.
- Chromosomal Disorders: These arise from abnormal numbers or structures of chromosomes. Down syndrome is one of the most well-known chromosomal disorders, resulting from an extra copy of chromosome 21.
- Multifactorial Disorders: These conditions result from a combination of multiple genetic factors and environmental influences. Heart disease and diabetes are examples. They do not follow simple inheritance patterns, making them more complex to study.
- Mitochondrial Disorders: These result from mutations in the DNA of mitochondria, often affecting energy production within cells. Conditions like Leber's Hereditary Optic Neuropathy fall into this category.
It is crucial to recognize these classifications, as they govern treatment options and patient management strategies.
The Role of Genetics in Disease Susceptibility
Genetics plays a significant role in determining an individual's susceptibility to various diseases. While environmental factors like diet and lifestyle contribute considerably, genetic predisposition can make some individuals more vulnerable to specific conditions. This relationship is often studied in the context of:
- Family History: A family background of certain genetic disorders suggests a higher risk for relatives. For instance, having a family member with breast cancer can increase the risk due to shared genetic factors.
- Gene-Environment Interactions: Often, genetic risk factors interact with environmental triggers to initiate disease. For instance, an individual with a genetic predisposition to lung cancer who smokes is at greater risk compared to a non-smoker.
- Genetic Testing: Advances in technology allow for precise genetic testing, helping identify individuals at risk for certain disorders. These tests can provide crucial information for preventative measures and personalized treatment plans.
Understanding the relationship between genetics and disease susceptibility informs both research and clinical practices, offering pathways for prevention and targeted interventions.
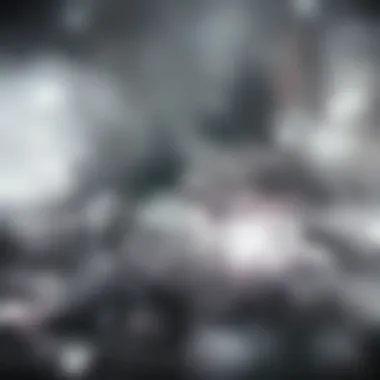
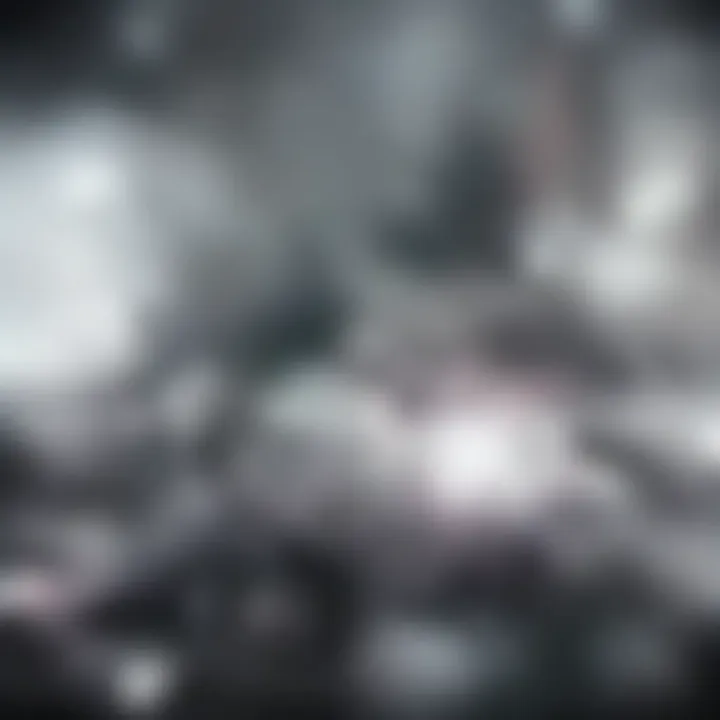
Ethical Considerations in Genetic Research
The topic of ethical considerations in genetic research is crucial in a world increasingly influenced by genetic technology. Advancements in genetics offer significant benefits, but they also introduce a range of ethical dilemmas that researchers must navigate. These considerations help guide the responsible application of genetic knowledge while protecting individuals and society from potential harms.
One of the most pressing ethical issues is informed consent in genetic testing. Individuals undergoing genetic testing should have a clear understanding of what the testing entails, including the implications of the results. This means they should be fully educated about potential outcomes, risks, and the emotional impact that genetic information may have on their lives. Researchers must ensure that consent is not only obtained but that it is informed, respecting the autonomy of participants. Transparent communication is key in this process.
"Informed consent is the foundation of ethical research practice. Without it, individuals may not fully appreciate the significance of their genetic data."
Informed Consent in Genetic Testing
Informed consent plays a vital role in the integrity of genetic testing. Researchers must provide comprehensive information about the testing process, including how the data will be used and who will have access to it. Participants should be aware of their right to withdraw consent at any time.
The complexity of genetic data adds another layer of difficulty. Genetic information can reveal not just personal health risks but also family histories, which might affect relatives who have not consented to the testing. This situation raises ethical concerns regarding familial rights and privacy.
Furthermore, the language used to explain genetic testing must be accessible. Scientific jargon should be minimized so that participants can understand fully what they are consenting to. Researchers need to adopt an empathetic approach, guiding participants through the process while answering their questions.
The Genetic Privacy Debate
The genetic privacy debate is another essential aspect of ethical considerations in genetic research. As genetic testing becomes more common, there are rising concerns about how genetic data is stored and shared. The possibility of data breaches or misuse of genetic information by third parties, such as insurance companies or employers, poses significant risks to individuals.
Privacy laws, such as the Genetic Information Nondiscrimination Act (GINA) in the United States, aim to protect individuals from discrimination based on genetic information. However, these laws have limitations, and not all countries have robust frameworks to ensure genetic data is protected. Thus, understanding where one's genetic data goes and how it can be used is critical for anyone considering genetic testing.
In addition, societal implications must be reflected on. If certain genetic traits are deemed 'favorable,' this may lead to societal pressure to undergo testing or even modify one's genetic makeup. These pressures raise important questions about societal values and individual rights.
In summary, addressing ethical considerations in genetic research is necessary for ensuring public trust in science. The principles of informed consent and data privacy must be upheld to maintain integrity. Future innovations in genetics demand ongoing ethical dialogue among researchers, ethicists, and the community to navigate the potential benefits and pitfalls of these technologies.
Future Directions in Genetic Research
Genetic research is advancing rapidly, and this brings both promise and challenges. Understanding future directions is essential for development in this field. As researchers delve deeper into the complexities of genes, several areas stand out. The evolving landscape includes innovative technologies, ethical implications, and prospective health applications. These directions provide insights into how genetics can transform various sectors.
The Promise of Synthetic Biology
Synthetic biology aims to manipulate biological systems for various applications. This field combines biology and engineering to design new biological parts and systems. The potential benefits are substantial. For instance, synthetic biology could lead to the creation of organisms that produce biofuels efficiently or synthesize medicines at a lower cost.
Synthetic enhancement allows for more tailored solutions to complex problems. Here are key elements:
- Targeted Therapeutics: Custom-built organisms can be designed to produce specific drugs, minimizing side effects and maximizing efficacy.
- Sustainable Production: Using engineered microbes to create materials like plastics can reduce dependence on fossil fuels.
- Environmental Solutions: Bacteria can be modified to clean pollutants from soils or waterways.
However, these advancements raise ethical questions. The manipulation of life forms must be approached carefully, ensuring safety and ecological balance. Balancing innovation with responsibility is crucial.
Innovations in Gene Therapy
Gene therapy involves altering genes within an individual’s cells to treat or prevent diseases. Recent innovations have made gene therapy more effective than ever. Techniques such as viral vectors allow for precise delivery of therapeutic genes. This method has shown promise in treating genetic disorders, cancers, and viral infections.
Significant advancements include:
- CRISPR-Cas9: This gene-editing technology allows for precise modifications. It has the potential to correct genetic mutations at their source.
- Adeno-Associated Virus (AAV): AAV vectors are being used for safer and more targeted gene delivery. This method reduces immune response, enhancing treatment efficacy.
- Long-Term Durability: New approaches aim to ensure that the effects of gene therapies last longer, reducing the need for repeated treatments.
Gene therapy’s ongoing evolution signifies a major leap in medicine, changing how we approach disease management.
The future of gene therapy hinges on ethical practices and regulatory oversight. Researchers must continue to navigate these waters carefully to ensure patient safety while delivering groundbreaking treatments.
Concluding Remarks
In discussing the role and function of genes throughout this article, it is essential to reflect on the intricate nature and significance of genetic research. Genes serve as the fundamental units of heredity and are crucial in determining the biological traits of organisms. Understanding genes provides insights into various biological processes, molecular mechanisms, and the interconnectedness of all living organisms. It forms the basis for comprehension in fields like genetics, medicine, and evolutionary biology.
The exploration of genes leads to numerous benefits in terms of health and technology. For instance, advancements in gene editing and therapy are reshaping medical treatments, allowing for therapies that target specific genetic disorders. Additionally, knowledge of gene function fosters a greater understanding of disease susceptibility and prevention strategies, impacting public health.
"Genetic research is not just about understanding life; it’s about improving it."
Ethical considerations, such as informed consent and genetic privacy, also arise when discussing the implications of genetic knowledge. As we push boundaries in genomics, awareness and dialogue regarding these matters become critical. By engaging with these topics, researchers, students, and professionals can ensure that the understanding of genetics is applied responsibly and beneficially.
Finally, looking ahead, ongoing advancements in synthetic biology and genomic technologies promise to enrich our understanding of life, offering new avenues for exploration. The future of genomics holds limitless potential, inviting curiosity and innovation to tackle the challenges we face in health and sustainability.
Summarizing Key Insights on Genes
Throughout this article, several key insights about genes have emerged. First, it is clear that genes are not isolated entities but are part of a complex system that involves interaction with environmental factors.
- Genes as Units of Inheritance: They dictate biological traits passed from one generation to the next.
- Molecular Mechanisms of Gene Expression: Transcription and translation are vital processes that convert genetic information into functional products.
- Impact on Health: An understanding of genetics is crucial in deciphering diseases and developing therapies.
- Ethical Considerations: Responsible handling of genetic information is fundamental in research and application.
These insights underscore the essential role genes play in shaping life as we know it. As science progresses, maintaining a holistic perspective on genetics is vital to grasp its implications for society and the environment.
Looking Ahead: The Future of Genomics
The future of genomics is promising and filled with opportunities for breakthroughs. The advancements seen in recent years will likely continue to expand our knowledge and capabilities. Some anticipated trends in genetic research include:
- Synthetic Biology: This emerging field focuses on designing and constructing new biological parts, devices, and systems. Innovations in synthetic biology could lead to significant advancements in biotechnology and medicine.
- Gene Therapy Innovations: Research in gene therapy is developing rapidly, with potential to treat genetic disorders at their source through techniques like CRISPR-Cas9.
- Personalized Medicine: The future may hold a movement towards treatments tailored to an individual’s genetic profile, improving efficacy and minimizing side effects.
- Public Engagement and Education: Raising awareness about genetics and its implications will foster informed conversations about its applications in society.