Detailed Guide to Primary Skeletal Muscle Cell Culture
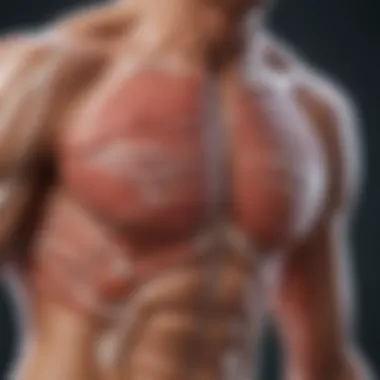
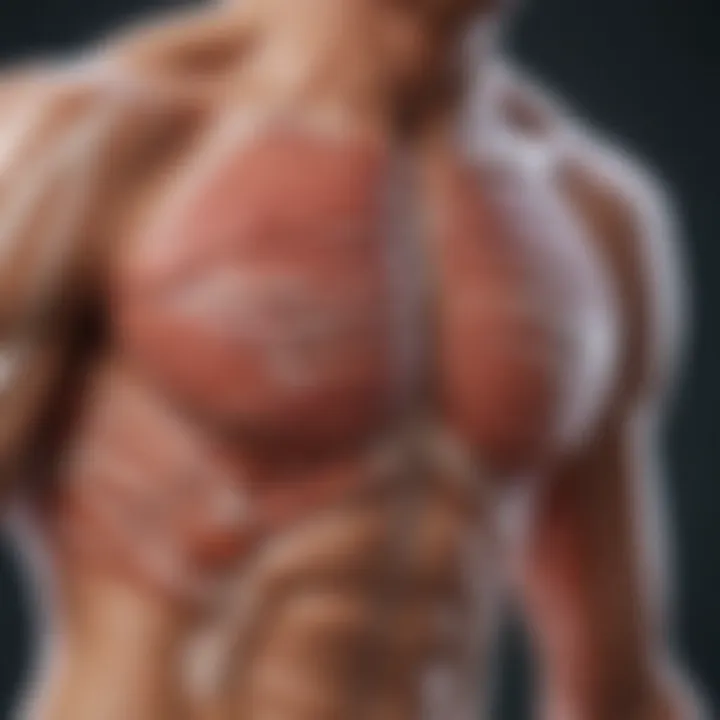
Intro
When it comes to unraveling the complexities of skeletal muscle biology, primary muscle cell culture stands as a foundational technique. This process not only provides researchers a window into the unique characteristics of muscle cells but also reinforces our understanding of muscle development, regeneration, and related therapeutic applications. Delivering a dependable and reproducible protocol is essential for anyone looking to explore this field further, whether they are seasoned scientists or new enthusiasts.
In this article, we will outline an extensive protocol for primary skeletal muscle cell culture. Our aim is to equip you with the knowledge and information necessary to navigate each step, from the initial isolation of tissues right through to the nuances of culture conditions and what to do when things don’t go as planned. Alongside highlighting practical techniques, we will delve into the broader implications of skeletal muscle research, offering insights that encourage a holistic understanding of muscle cell biology.
This journey will not just be about the steps involved but also about understanding the importance of each phase within the protocol. The applications of this research are manifold, spanning regenerative medicine and beyond, which is why we emphasize an approach driven by rigor and reproducibility.
Methodologies
Description of Research Techniques
The process of culturing primary skeletal muscle cells starts with careful tissue isolation, often sourced from animal models like mice or rats. Once the tissue is procured, several methods such as enzymatic digestion using collagenase or trypsin are employed to dissociate muscle fibers into individual cells. The choice of enzyme can significantly affect cell viability and characteristic, which is a crucial factor to consider.
Following isolation, cell plating in the appropriate culture medium is essential. The medium should closely mimic the muscle environment, often supplemented with growth factors to support cell proliferation. For example, the addition of insulin and basic fibroblast growth factor (bFGF) has shown to enhance myoblast growth.
A typical approach follows this sequence:
- Acquire muscle tissue
- Enzymatically digest the tissue
- Isolate and wash the cells
- Plate the cells in a growth medium
Tools and Technologies Used
To execute this protocol effectively, a few key pieces of equipment come into play. Basic lab tools like pipettes, culture dishes, and laminar flow hoods are a given. However, advanced technologies can significantly enhance the culture process:
- Microincubators offer precise control over temperature and gas compositions, which are vital for maintaining necessary culture conditions.
- Automated cell counters help determine cell density and viability efficiently.
- Flow cytometry can be used to analyze cell populations with high accuracy, an important aspect when examining muscle cell types.
Utilizing these tools not only streamlines the process but also can improve the reproducibility of results which is paramount in scientific research.
"A good protocol is like a map; it guides you step by step through what could otherwise be a confusing and unpredictable journey."
Discussion
Comparison with Previous Research
The evolution of primary muscle cell culture techniques has been significant over the years. Previous studies often relied on more rudimentary methods with limited understanding of optimal growth conditions. Current methodologies integrate findings from earlier research and build on them, refining various steps to enhance yield and purity of myoblast cultures. For instance, understanding the role of nutrient supplementation has evolved dramatically, allowing for richer culture environments that support better cell growth.
Theoretical Implications
Engaging in primary skeletal muscle cell culture holds not just practical implications but also theoretical ones. Each protocol contributes to a broader understanding of muscle physiology. Insights gathered from these cell cultures deepen our grasp on typical muscle regeneration mechanisms, which can lead to improvements in therapies for degenerative muscle diseases.
The take-home message here is that by diving into the methodologies and outcomes of skeletal muscle cell culture, researchers lay the groundwork for innovative approaches in regenerative medicine, contributing to important advancements in the field.
As we unfold this comprehensive guide, we invite you to dive deeper into each segment. There’s much to be learned from everything from technique to applications that could very well alter the landscape of muscle biology.
Intro to Skeletal Muscle Cell Culture
Understanding skeletal muscle cell culture is fundamental for various applications in biological research and medicine. These primary cells not only serve as a model for studying muscle development and pathology but also play a crucial role in regenerative medicine. Researchers are keen on muscle cell cultures because they enable experiments that can elucidate cellular mechanisms underlying muscle diseases, injuries, and responses to various treatments.
Importance of Primary Skeletal Muscle Cells
Primary skeletal muscle cells hold immense value due to their natural state and functional integrity, which are instrumental in obtaining accurate experimental results. Unlike cell lines that may undergo extensive genetic modifications over time, primary cells maintain their physiological characteristics. For instance, when looking to study muscle regeneration, the use of primary myoblasts—muscle progenitor cells—provides a clear view into the regeneration process, making findings more applicable in clinical settings.
Here are several reasons why primary skeletal muscle cells are especially important:
- Realistic Responses: They reflect natural responses to stimuli, making experiments more relevant.
- Disease Modeling: Useful in simulating muscle diseases, which aids in discovering potential therapeutic strategies.
- Regenerative Insights: Integral for understanding how muscles heal and regenerate post-injury.
- Drug Testing and Toxicology: Serve as an effective model for evaluating drug effects, especially concerning muscle-specific compounds.
Historical Context of Muscle Cell Culturing
The journey of muscle cell culture has evolved significantly over decades. Early attempts date back to the mid-20th century when researchers primarily relied on animal models. Techniques were rudimentary, often yielding low success rates and questioning the efficacy of muscle cell cultures. It wasn't until the advent of more sophisticated techniques, like enzymatic digestion and serum supplementation, that primary cultures began to thrive.
In the late 1970s, scientists managed to isolate primary skeletal muscle cells using these improved culturing methods. This breakthrough expanded the horizon of muscle biology research. During the 1980s and 1990s, advancements in molecular biology prompted an intensified interest in genetic manipulation, enabling more targeted muscle cell studies. Today, researchers can delve into various aspects of muscle cell biology, contributing to a better understanding of physiology and pathology.
"The evolution of muscle cell culture illuminates the balance between scientific rigor and innovative exploration, opening unprecedented doors to discovery."
The historical backdrop lends crucial insight into how skeletal muscle cell culture protocols have emerged, ensuring that current methodologies are rooted in past learnings. As we navigate contemporary applications, it's important to reflect on these developmental milestones that have shaped the field into what it is today.
Materials Required for Cell Culture
The area of materials necessary for cell culture acts as the backbone of any successful research endeavor involving primary skeletal muscle cells. Without the right materials, even the most innovative protocols can become futile. By ensuring quality in the materials used, researchers can improve reproducibility and reliability of results, which are essential in scientific investigations. This section delves into two primary aspects: Essential Equipment and Cell Culture Media and Reagents.
Essential Equipment
Setting up a cell culture laboratory correctly is vital. The right equipment provides a clean, controlled environment needed for growing cells effectively. Here’s a rundown of the essential tools:
- Laminar Flow Hood: A must-have for preventing contamination, this hood creates a sterile working area.
- Incubator: Regulating temperature and CO2 levels, it mimics the conditions found in the body, which is crucial for cell proliferation.
- Biosafety Cabinet: This offers additional protection, especially when handling potentially hazardous biological materials.
- Centrifuge: This is essential for separating cells based on density and for washing cells to remove impurities.
- Cryopreservation Equipment: For long-term storage, it’s important to have equipment like liquid nitrogen tanks to preserve cell lines.
Having the right equipment isn’t just about having shiny new tools. It’s about durability and reliability. You want your gear to withstand the rigors of frequent use without faltering. Simply put, if it breaks down mid-experiment, you could be chasing your tail trying to fix things, or worse, you might waste valuable time and materials.
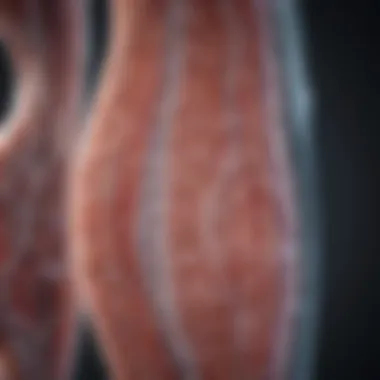
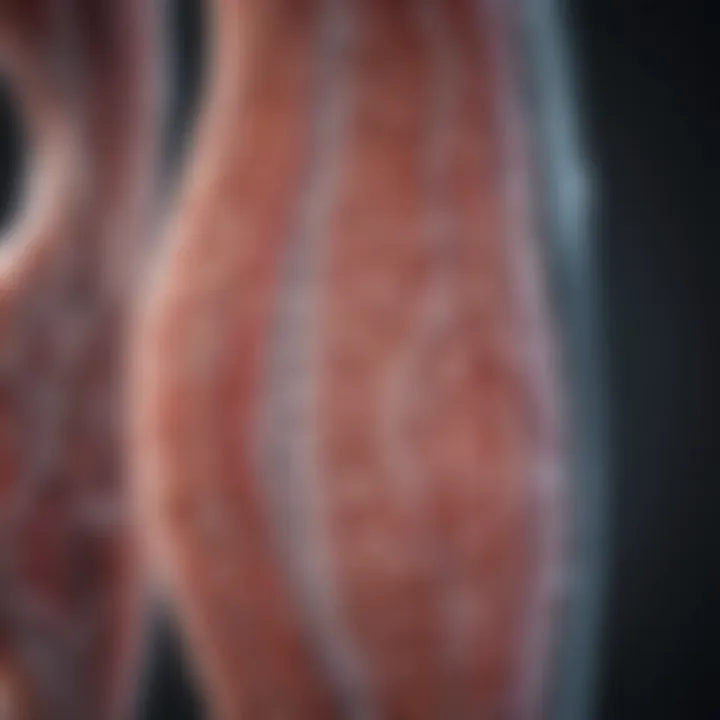
Cell Culture Media and Reagents
Equally as important as the equipment is the media and reagents that support cell growth. These are the lifeblood that keeps cells alive in culture. Without nutrient-rich media, muscle cells would simply wither away, not unlike a plant without water. Here are the key elements to consider:
- Basal Media: Common options include Dulbecco's Modified Eagle Medium (DMEM) or Roswell Park Memorial Institute Medium (RPMI-1640). These forms deliver essential nutrients and amino acids.
- Serum Additives: Fetal Bovine Serum (FBS) often serves as a supplement, providing growth factors that are particularly essential for muscle cells.
- Antibiotics: Using penicillin-streptomycin can help in reducing contamination from bacteria and fungi, which is a constant threat in cell cultures.
- Trypsin: This enzyme is used during cell passaging to detach adherent cultures. It’s a critical reagent for maintaining healthy cell lines.
"The choice of media and reagents can significantly affect the outcomes of your experiments, so don’t skimp on quality."
Choosing the right media isn't a trivial detail; it's akin to selecting the right recipe for brewing a well-crafted beer. Ingredients matter. Using subpar or outdated reagents may lead to reduced cell viability or inconsistent results, leaving researchers wrestling with errors that could have been avoided.
In summary, the materials used in your primary skeletal muscle cell culture setup are crucial for ensuring a robust and effective research environment. From the right equipment working hand-in-hand with quality media, every component must be aligned with the ultimate aim of achieving successful cell culture.
Tissue Isolation Techniques
Tissue isolation is a cornerstone of skeletal muscle cell culture, ensuring the integrity and viability of primary cells for subsequent experimental processes. The significance of this section cannot be overstated; it directly influences the outcomes and reproducibility of future analyses. Proper isolation sets the stage for successful cell cultivation, allowing researchers to delve into muscle pathophysiology, drug responses, and more, with precision.
Selection of Muscle Tissue Source
The selection of the muscle tissue source is vital for ensuring a robust cell culture setup. It's essential to choose a muscle type that best aligns with your research goals. Commonly utilized sources include:
- Skeletal muscle from mice or rats: This is favored due to its well-characterized genetics and ease of access.
- Human skeletal muscle: While more challenging to obtain, it provides insight into human biology, particularly in disease contexts.
- Aquatic or avian muscle: These alternatives may be necessary for specific physiological inquiries but come with their unique challenges.
Each muscle type harbors inherent cellular characteristics, such as growth rates and differentiation capabilities. For example, selecting the gastrocnemius muscle may yield more progenitor cells, while quadriceps is often richer in mature fibers. Thus, the decision should be guided by both availability and the nature of your experimental design.
Dissection Methodology
Once the tissue source is selected, dissection becomes the next step in the process. It’s crucial to conduct this step under sterile conditions to prevent contamination. The following are the key considerations:
- Preparation: Equip your workstation with sharp surgical tools, sterile dissection trays, and disinfectants. Sterile gloves are a must.
- Technique: Carefully remove the muscle from the carcass. Aim to carefully separate the muscle bundles without damaging surrounding tissues. Short, precise incisions often work best.
- Minimizing Thermal Stress: Remember to keep the tissue cool; exposure to temperatures above physiological levels can lead to cell death. An ice bath can be particularly helpful during this stage.
Once the muscle is extracted, it’s essential to handle the tissue delicately, maintaining its structure as much as possible. An effective dissection can greatly affect the subsequent yield of viable cells.
Prepping Tissue for Culture
With the tissue now secured, the next phase involves prepping it for cell culture. This step is crucial for ensuring that the muscle cells can flourish once placed in culture conditions:
- Washing: Thoroughly rinse the muscle tissue with sterile saline solution to remove any residual blood and contaminants.
- Mechanical Separation: Using scissors and forceps, chop the muscle into smaller pieces. This increases the surface area available for enzymatic digestion.
- Enzymatic Digestion: Treatment with enzymes such as collagenase or trypsin is necessary to dissociate the fibers into individual cells. The duration and concentration of the enzyme should be optimized based on preliminary trials. Careful monitoring is crucial to prevent over-digestion, which can lead to cell death.
- Filtering: After digestion, pass the cellular suspension through a sterile mesh to remove larger debris. This ensures a cleaner culture environment allowing for better cell growth.
- Centrifugation: Finally, centrifuge the cell suspension to pellet cells and prepare them for resuspension in appropriate culture media.
Before proceeding to actual culturing, always verify cell viability using techniques like trypan blue exclusion. This preparatory work pays dividends in ensuring a healthy and productive cell culture in the long run.
"Successful tissue isolation not only enhances the yield of muscle cells but ensures their functionality, paving the way for groundbreaking research."
Cell Culture Techniques
Cell culture is the backbone of any skeletal muscle research, providing a controlled environment to study muscle cell behaviors, improve experimental results and push the bounds of our understanding. Utilizing these techniques allows researchers not only to dissect the physiological, biochemical, and molecular aspects of muscle biology but also to create innovative solutions for various medical challenges.
Engaging with cell culture techniques requires stretching far beyond the mere act of maintaining cells in a dish; it is an intricate dance of science and art. Each adjustment—be it environmental parameters, feeding schedules, or the fine-tuning of growth supplements—can have cascading effects on the muscle cells. Therefore, mastering these techniques is critical for anyone involved in muscle biology, regenerative medicine, or related fields.
Cell Seed and Growth Factors
The initial step in culturing skeletal muscle cells is determining the cell seeding density and the applicable growth factors. This phase presents a nuanced balancing act; failing to achieve the right cell density can lead to either over-confluence or under-utilization of the available substrate. Research shows that skeletal muscle cells, especially myoblasts, require specific growth factors like insulin-like growth factor-1 (IGF-1) and fibroblast growth factor (FGF) for optimal growth.
These growth factors are elevators in the world of muscle cell culture, as they provide important signals for differentiation and proliferation. When applying growth factors, it's important to tailor the concentrations to achieve successful outcomes. For example, a common mistake is assuming a one-size-fits-all approach.
- Insulin (10 μg/mL)
- Beef Extract (0.2%)
- Fetal Bovine Serum (FBS) (10-20%)
All these components together act as fuel, pushing the cells towards differentiation and maturation, influencing everything from size to metabolic activity. The ideal concentrations can vary based on cell type and its specific needs, requiring constant optimization through monitoring and vivacious experimentation.
"The success of muscle cell cultures hinges on the tireless pursuit of understanding what each cell type desires. The cells can almost tell if you're not giving them what they need, and they won't be shy about it!"
Culturing Conditions and Environment
Moving on to culturing conditions, the ambiance of the culture dishes can make or break your experiment. Factors such as temperature, pH, and gas composition are pivotal because muscle cells are extraordinarily sensitive to their surroundings.
Muscle cells usually thrive best at the physiological temperature of 37°C and require a CO2 concentration of 5% to maintain optimal pH levels—this is where a CO2 incubator comes in handy. Additionally, the osmolarity and ionic composition of the culture media are things that should not be shrugged off.
A great culture environment is one where:
- Nutrient media is refreshed regularly.
- Contaminants are kept at bay with sterile techniques.
- Conditions are checked against physiological relevance.
Caring for cultured muscle cells extends to recognizing their need for a balanced, rich environment. Regular monitoring under a microscope will let you keep an eye on the cell morphology, which is crucial for assessing health and the viability of the culture. Lastly, passing the cells at just the right density and timing ensures they aren’t choked with overgrowth, nor left starving.
In essence, the culturing process is more than simple operations; it’s about anticipating the needs of your muscle cells at each step, ensuring they grow as robust and functional as their in-vivo counterparts.
Monitoring and Maintenance of Cultures
An essential facet of any cell culture protocol is the systematic monitoring and maintenance of cultures. This step cannot be glossed over when engaging with primary skeletal muscle cells; it maximizes the efficacy of your research and contributes vastly to the reliability of your results. Monitoring setups don't merely keep an eye on the experiment; they help in crafting a conducive environment for healthy cellular activity. This segment will delve into the crucial elements involved, the benefits derived, and the necessary considerations when keeping your muscle cells in tip-top shape.
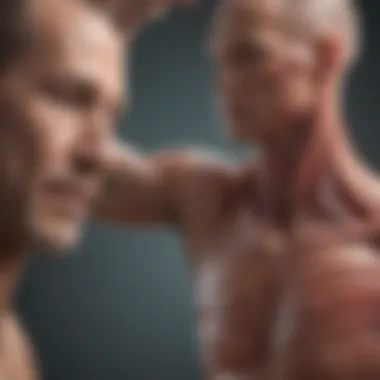
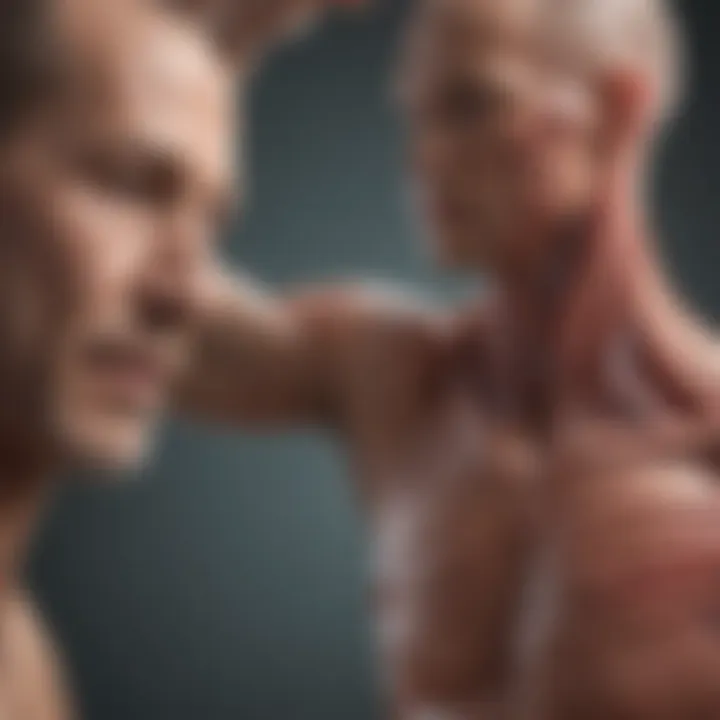
Observing Cell Morphology
One of the first tasks in monitoring is observing cell morphology. Morphology offers a visual cue that speaks volumes about cell health. You would want to look for characteristics such as cell shape, size, and density. Healthy skeletal muscle cells typically appear elongated with clear borders, while unhealthy or stressed cells may exhibit rounded shapes, clumping, or detachment from the culture substrate.
Regularly taking microscope snapshots captures these changes and may also serve as a historical record, depicting the cells’ journey from isolation to culture over time.
- Importance of Morphology:
- A well-structured morphology indicates cell differentiation and growth.
- Deviant morphology might signal contamination or nutrient deficiency.
Using phase contrast microscopy can be particularly effective since it enhances transparency and gives a better view of live cells. Seeing the muscle cell's normal structure functioning as expected reassures you that the culture process is going smoothly.
Adjusting Culture Conditions
Adjusting culture conditions is essential for keeping your cells thriving. It’s a bit like fine-tuning a musical instrument; even minor tweaks could lead to harmonious outcomes.
- Key Factors to Monitor:
- Benefits of Adjustments:
- Considerations When Adjusting:
- pH Levels: Over time, the media could become acidic or basic, impacting cell health. Aim for a pH of around 7.4.
- Temperature: Consistent incubation at 37°C is ideal, but fluctuations could spook your cultures.
- Nutrient Supply: Regularly check the concentrations of essential nutrients, especially glucose and amino acids.
- Timely adjustments can prevent cell death and increase viability rates.
- They allow you to identify needs before larger issues arise, thus saving time and resources.
- Changes should mesh with the specific experimental design you're working with. One size doesn’t fit all.
- Be conservative with alterations; sudden shifts in culture conditions might lead to shock for the cells.
"Monitoring is not just about identifying problems, but rather about enhancing cell growth and performance."
The key takeaway here is that maintaining an optimal environment for cultured skeletal muscle cells requires diligence and a keen eye. It’s not a set-and-forget situation but rather an ongoing process that can directly influence the success of your research outcomes.
Troubleshooting Common Issues
Troubleshooting common issues in skeletal muscle cell culture is a vital aspect of ensuring successful experiments and reproducible results. When embarking on this intricate journey, researchers often encounter a slew of hurdles, two of the most prevalent being contamination problems and low cell viability. Addressing these issues swiftly can save time, resources, and countless hours of stress. Thus, understanding how to troubleshoot these common bottlenecks is not just beneficial, but necessary for the integrity of the research.
Contamination Problems
Contamination can play havoc with experimental outcomes and is a concern that plagues many cell culture setups. This problem may arise from various sources: airborne particles, contaminated reagents, or even improper handling techniques. The effects of contamination are manifold, leading to skewed data and misleading conclusions. For instance, bacterial, fungal, or viral contaminants can alter cellular metabolism, and growth rates, or even induce apoptosis, severely distorting results.
To tackle contamination, consider the following:
- Sterile Techniques: Always practice strict aseptic conditions while working with cells. This includes using gloves, masks, and sterilized equipment.
- Regular Monitoring: Routinely check cell cultures for signs of contamination, like unusual turbidity or changes in pH. Implementing a schedule for microscopic examination can aid in early detection.
- Use of Antibiotics: While not a complete solution, the incorporation of antibiotics can act as a preventive measure against bacterial contamination. However, one must use them judiciously, as over-reliance can mask contamination problems rather than solve them.
"An ounce of prevention is worth a pound of cure." This adage definitely holds true in the realm of cell culture, where proactive steps can nip contamination issues in the bud.
Low Cell Viability
Cell viability is a crucial indicator of the health and functional capacity of cultured muscle cells. Low cell viability often reflects underlying issues that need to be identified and remedied. Several factors can contribute to this predicament, including suboptimal culture conditions, incorrect media formulation, or even over-confluence of the cells. Low viability not only complicates experimental outcomes but can also frustrate researchers who are striving for consistency and accuracy.
Here are some strategies to improve low cell viability:
- Optimize Culture Conditions: Ensure that environmental factors such as temperature, CO2 levels, and humidity are set correctly. These variables can significantly influence cell growth and survival rates.
- Check Media Composition: Sometimes, the cell culture media might lack essential nutrients or growth factors. Make sure the chosen media match what skeletal muscle cells require to thrive.
- Subculture Routinely: Avoid overcrowding your cultures, which can lead to decreased cellular health. Regularly subculture cells to maintain optimal density and condition.
Ultimately, paying attention to detail can greatly enhance the viability of muscle cells in culture, paving the way for more reliable and reproducible research results.
By mastering the steps of troubleshooting common issues like contamination and low cell viability, researchers can better navigate the complexities involved in primary skeletal muscle cell culture, ensuring that their findings contribute valuable insights into the field.
Advanced Techniques in Muscle Cell Culture
Advanced techniques in muscle cell culture are crucial for pushing the boundaries of our current understanding and manipulation of skeletal muscle cells. These sophisticated methods not only enhance our ability to grow and maintain muscle tissue in vitro, but they also create a foundation for groundbreaking research in regenerative medicine and beyond. Here, two major innovative strategies catch the eye: genetic manipulation and three-dimensional culturing systems.
Research in muscle biology increasingly relies on these advanced techniques to unlock new pathways and applications while dealing with the inherent challenges of culturing muscle cells. These innovations yield a level of precision and complexity that traditional methods often struggle to achieve, thus significantly amplifying the potential for real-world applications.
Genetic Manipulation of Muscle Cells
Genetic manipulation is a powerful tool in the arsenal of researchers focused on muscle cell biology. Altering genes within muscle cells can help unveil roles of specific genes in muscle fiber development, metabolism, and repair processes. Through techniques like CRISPR-Cas9, scientists can easily knock out or insert target genes, which provides insight into the biological pathways influencing muscle cellular functions.
For instance, modifications in genes related to myogenesis can lead to the development of muscle fibers that better mimic natural physiology. This holds promise for creating tissue-engineered constructs for therapies aimed at muscle wasting diseases or injuries. Furthermore, gene editing allows researchers to study muscle cell responses to various treatments and environmental stimuli under controlled conditions. The implications are immense:
- Regenerative therapies: Enhancing muscle repair mechanisms through targeted gene delivery
- Modeling diseases: Investigating genetic disorders such as muscular dystrophy with patient-derived cells
- Pharmacological testing: Evaluating drug responses on genetically modified muscle cells
While genetic manipulation opens up unparalleled avenues, it hammers home the need for careful consideration of ethics and safety, ensuring any modifications align with regulatory close-outs. Therefore, researchers must continuously engage in discussions regarding responsible handling of genetic technologies.
Three-Dimensional Culturing Systems
Three-dimensional culturing systems represent another leap forward. Unlike traditional two-dimensional cultures, which often lead to uncharacteristic cellular responses, 3D systems more accurately replicate the natural extracellular matrix and cellular environments found in vivo. Utilizing scaffolds made from biocompatible materials, these systems can better mimic the architecture of muscle tissue.
The benefits from 3D cultures include:
- Enhanced cell interactions: Promoting more natural signaling pathways among muscle cells
- Improved functionality: Yielding muscle networks that contract, simulating physiological activity
- Increased viability: Preventing the senescence that often plagues 2D cultures, allowing cells to thrive longer
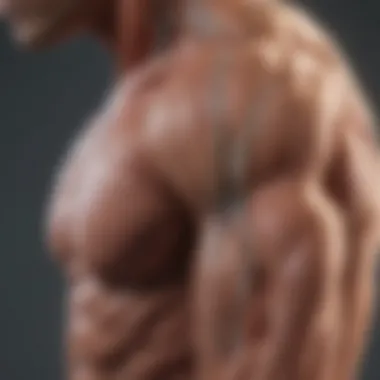
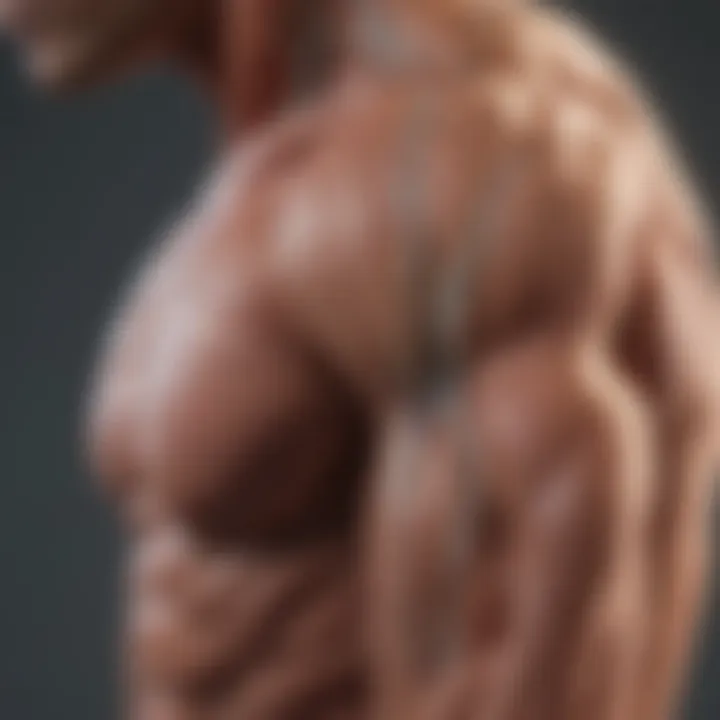
Applications of 3D systems are vast. In drug development, they offer more reliable predictive models for human responses, helping streamline the process of bringing new therapies to market. In regenerative medicine, they serve as platforms for evaluating novel graft materials for treating muscle injuries or atrophy.
As researchers continue to refine these advanced techniques, the possibilities for muscle cell culture expand exponentially. Greater understanding of muscle biology not only enlightens ongoing studies but also lays the groundwork for future developments in therapies and applications.
These advanced techniques underscore an ever-complex relationship between muscle cells and their environments, spotlighting the intrinsic intricacies of cell behavior that demand sophisticated approaches to effectively translate findings into functional applications.
Applications of Cultured Skeletal Muscle Cells
The realm of skeletal muscle cell culture holds a treasure trove of applications that play a significant role in various fields of biology and medicine. These applications not only showcase the capabilities of cultured muscle cells but also highlight their potential to contribute to advancements in regenerative therapies, drug testing, and understanding muscle physiology.
Understanding how skeletal muscle cells behave in a controlled environment allows researchers to dissect complex processes that occur in the body. As we delve deeper into specific applications, one can appreciate the impact these cultures have not just in the lab, but also in broadening our understanding of human health.
Regenerative Medicine
Regenerative medicine stands at the forefront of medical innovation, and skeletal muscle cells are pivotal in this field. With conditions like muscular dystrophy or injuries leading to muscle loss, utilizing cultured muscle cells for therapeutic purposes has gained momentum. There are a few key areas to consider when discussing the application in this domain:
- Cell Replacement Therapy: Cultured muscle cells can be engineered to replace dead or damaged tissues. This technique opens avenues for treating patients who have suffered accidents or diseases that compromised skeletal muscle integrity.
- Tissue Engineering: Beyond just replacing cells, researchers are exploring techniques to engineer entire muscle tissues. This involves scaffolding that supports muscle-cell growth in three-dimensional structures. For instance, using bio-ink in 3D printing technologies provides an elegant solution to create muscle constructs that can mimic natural behavior effectively.
- Studying Muscle Regeneration: Cultured muscle cells allow for direct observation of muscle regeneration processes. Researchers can manipulate conditions to understand how muscle fibers grow and repair themselves after injury. This knowledge is essential in developing treatments that can kickstart or enhance regeneration in vivo.
"Skeletal muscle regeneration is not merely a biological repair process, it's an intricate dance of signaling, cellular behavior, and environmental cues that can be studied effectively through dedicated cell cultures."
Additionally, skeletal muscle cell culture facilitates gene therapy approaches, which can be used to correct genetic defects at the cellular level. Techniques such as CRISPR are often applied within cultured cells, allowing scientists to probe and potentially fix mutations before applying these therapies to whole organisms.
Drug Development and Toxicology Testing
The pharmaceutical industry heavily relies on in vitro testing systems for evaluating drug effects. Skeletal muscle cells provide a robust platform for assessing drug safety and efficacy. Here are some pertinent factors that underline their relevance in drug development:
- Toxicity Assessments: One of the essential applications of muscle cell cultures is in toxicity testing. By exposing cultured muscle cells to new compounds, researchers can observe cellular responses that might indicate potential adverse effects before clinical trials. This helps in minimizing risks associated with drug toxicity.
- Pharmacodynamic Studies: The study of how drugs affect muscle cells can lead to a better understanding of drug action and interaction. Analyzing muscle cells in a controlled environment allows for the identification of optimal dosages for drugs targeting muscle-related diseases.
- High-Throughput Screenings: Cultured skeletal muscle cells can be used in high-throughput screening systems to quickly evaluate compound libraries. This process accelerates drug discovery by identifying promising candidates that effectively interact with muscle tissue.
As drug development becomes increasingly sophisticated, the role of cultured muscle cells will undoubtedly grow. Their ability to mimic human biology in vitro makes them indispensable for creating effective therapies tailored to muscle diseases.
In summary, the applications of cultured skeletal muscle cells are expansive and diverse. From regenerative medicine, which harnesses the unique properties of these cells to restore function, to drug development, where they serve as critical models for assessing the safety and efficacy of therapeutic agents, these cultures embody a vital resource for current and future scientific endeavors.
As the landscape of muscle cell research evolves, its impacts may ripple through multiple domains of health science, leading to breakthroughs that enhance human life.
Future Directions in Muscle Cell Research
Research in skeletal muscle cell culture is paving the way for innovative solutions to various health issues. As scientists unlock the mysteries of muscle function, the implications are far-reaching, impacting everything from injury recovery to chronic disease management. Future directions in this field not only address existing challenges but also open doors to new avenues of exploration that can substantially enhance our understanding and treatment of muscular disorders.
Emerging Technologies
The advent of novel technologies is reshaping how we think about and utilize skeletal muscle cells. One of the most promising developments is the use of bioprinting, which allows for the creation of complex three-dimensional muscle tissue structures. Researchers can precisely control the arrangement of cells, thereby mimicking the natural architecture found in the human body. This opens up new possibilities for in vitro studies and regenerative therapies.
Additionally, CRISPR-Cas9 technology is making waves in genetic manipulation, allowing scientists to manipulate muscle cell genes with greater precision than ever before. This capability provides insights into gene function in muscle cells, potentially revealing targets for treating genetic muscle disorders.
Another exciting frontier is organ-on-a-chip technology, which integrates living cells onto microchips to emulate human physiological conditions. These models can be instrumental in studying drug effects on muscle cells, reducing the reliance on animal models and optimizing drug testing processes for safety and efficacy.
"The future of muscle cell research lies in our ability to adapt and innovate with the tools at our disposal."
Through these emerging technologies, researchers can develop better models for studying diseases like muscular dystrophy or ischemia. This can lead to tailored treatments that are more effective and mitigate adverse effects on patients.
Potential for Personalized Medicine
The push towards personalized medicine is another key future direction in muscle cell research. By understanding the specific genetic makeup and environmental influences on an individual's muscle cells, treatments can be customized to suit their unique profiles. For instance, muscle cell cultures derived from patients can be used to test drug responses in a controlled environment, ultimately allowing practitioners to determine the most effective treatment plans.
Moreover, advancements in single-cell sequencing techniques provide insights into the heterogeneity of muscle cells. This can unveil variations in muscle regeneration among individuals, leading to more nuanced approaches in therapeutics. Knowing how specific individuals respond to treatments can optimize physical rehabilitation strategies, ensuring a better return to function after injury.
In summary, the future of muscle cell research is not just about understanding muscle biology. It encompasses a broader vision aimed at integrating cutting-edge technology with personalized treatment strategies. As scientists push the envelope, muscle cell research stands at the brink of transformative changes that promise improved patient outcomes in various medical fields.
Culmination and Summary
The conclusion and summary section is a vital component of this article on primary skeletal muscle cell culture. It encapsulates the core findings and insights presented throughout, providing a streamlined understanding of complex methodologies and their real-world applications. This is particularly significant as it reinforces the knowledge gained, aiding students, researchers, and educators in grasping key takeaways effectively.
One of the most important elements discussed is the critical nature of rigor in experimental design. Understanding how variations in methods, such as tissue isolation or cell culture conditions, can influence outcomes is essential for reproducibility. It serves as a reminder that meticulous planning and execution can bolster the reliability of results.
Additionally, recapitulating the various protocols and considerations involved emphasizes the intricacy of working with primary skeletal muscle cells. Here, the balance of practical techniques with theoretical knowledge becomes clear, laying the foundation for further exploration and innovation in the field.
In summary, this section solidifies the importance of thorough preparation and deep comprehension in the journey from basic muscle cell culture to groundbreaking applications in fields like regenerative medicine and drug development.
Recapitulation of Key Points
- Importance of Primary Skeletal Muscle Cells: These cells play a crucial role in muscle biology and in application areas such as disease modeling and drug testing.
- Tissue Isolation Techniques: Detailed strategies for selecting and dissecting muscle tissue underscore the foundational steps necessary for successful cell culture.
- Culturing Conditions: Highlighting the specific growth factors and environmental controls needed to maintain cell viability and promote growth.
- Monitoring and Maintenance: The significance of regular observation of cell morphologies helps catch early signs of issues, ensuring cultures remain healthy.
- Troubleshooting Common Issues: Understanding potential hindrances, such as contamination or low viability, equips researchers to better handle challenges in the lab.
- Applications of Cultured Skeletal Muscle Cells: Their applications in regenerative medicine and toxicity testing exemplify the practical impacts of this research, fueling future innovations.
Importance of Rigor in Experimental Design
Maintaining rigor in experimental design is not just about following protocols; it’s a mindset that ensures the integrity of scientific inquiry. By adhering to stringent methods and documentation practices, researchers pave the way for reproducible and valid results.
- Statistical Significance: Proper sample sizes and controls are crucial. A small oversight in this area can lead to data that may misrepresent findings.
- Standardized Procedures: Establishing protocols that can be replicated across labs enhances collaboration and knowledge sharing, ultimately driving progress in muscle biology.
- Robust Record Keeping: Every step taken in the experiment must be precisely documented. This provides a roadmap for others to follow and can assist in identifying where things went awry if results are not as expected.
Ultimately, the emphasis on rigorous methodologies fosters a culture of excellence in research, encouraging a new generation of scientists to innovate while paying respect to well-established practices and findings.
"Good science is built on solid foundations—when the basics are strong, the future can be limitless."
This tribute to foundation echoes the sentiments throughout the article. It encourages ongoing growth, while keeping the critical principles at the forefront.
In essence, the intersection of detailed protocol with methodological rigor serves as a guidepost for emerging researchers and seasoned professionals alike, ensuring that work in the realm of skeletal muscle cell culture remains impactful and forward-thinking.