Exploring the TEM Technique in Scientific Research
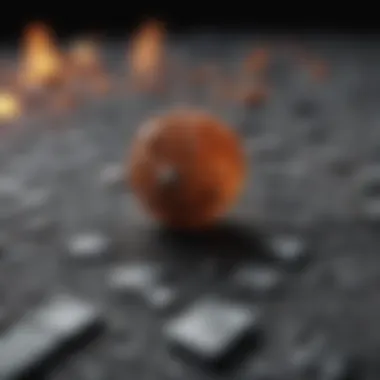
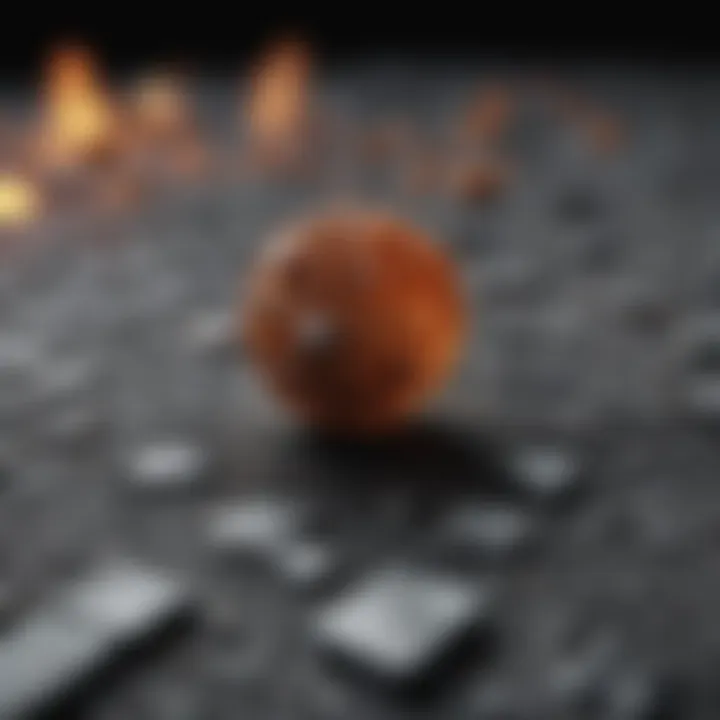
Intro
The transmission electron microscopy (TEM) technique has played a fundamental role in advancing scientific research, especially in the fields of materials science, biology, and nanotechnology. By utilizing the principles of electron optics, TEM allows researchers to visualize and study structures at the atomic level. This capability opens doors for elaborate analysis that reveals intricate details about micro and nanostructures.
The aim of this article is to provide a thorough examination of the TEM technique, exploring its principles, applications, advantages, and advancements. The following sections will guide you through the methodologies of TEM, presenting vital knowledge that will enhance your understanding of its significance in various scientific inquiries.
Methodologies
Description of Research Techniques
While the basic principle of transmission electron microscopy may seem straightforward, it encompasses a range of intricate techniques. The most common TEM processes include:
- Conventional TEM: This involves standard imaging to provide two-dimensional projections of samples.
- High-Resolution TEM (HRTEM): HRTEM is aimed at observing lattice structures, which offers insights into crystallography.
- Selected Area Electron Diffraction (SAED): SAED applies to phase identification and crystallinity analysis by obtaining diffraction patterns from selected areas.
- Scanning TEM (STEM): This technique combines scanning and imaging, creating a deep understanding of sample composition and morphology.
Each technique serves specific objectives and provides distinct information, thus broadening the scope of scientific research.
Tools and Technologies Used
To utilize the TEM technique effectively, a careful selection of tools and technologies is essential. Key components include:
- Electron Sources: Common sources are thermionic emitters and field emission sources. These allow for the generation of high-energy electrons required for imaging.
- Lenses and Optical Systems: Spherical and chromatic aberration-corrected lenses greatly enhance image quality, minimizing distortions in observed samples.
- Detectors: Modern systems often include charge-coupled devices (CCD) or direct electron detectors for efficient capture of data during imaging.
- Sample Preparation Equipment: Techniques such as ultramicrotomy and focused ion beam (FIB) milling are used to prepare samples to appropriate thickness for TEM analysis.
These technological advancements facilitate better imaging capabilities and provide insights that would be unattainable through other methods.
Discussion
Comparison with Previous Research
The evolution of the TEM technique has seen significant improvements over decades. One notable advancement has been the transition from bulk imaging to atomic resolution. Earlier TEM models, while effective, had limitations in resolution, which hindered detailed studies at the atomic scale. The introduction of aberration-corrected TEM systems has dramatically improved imaging ability, enabling detailed observation of materials with atomic precision.
Theoretical Implications
On a theoretical level, the TEM technique enhances our understanding of the underlying physics of materials. By allowing researchers to visualize materials at their microscopic level, it contributes to fundamental knowledge concerning electron interactions with matter. This bridging of theories with empirical data is essential for advancing fields such as nanotechnology and materials engineering.
The study of micro and nanostructures using TEM has become a cornerstone of modern science, providing insight into the properties and behaviors of materials that were previously out of reach.
Preamble to the Tem Technique
The transmission electron microscopy (TEM) technique holds a significant position in the field of scientific research, especially when it concerns investigating micro and nanostructures. Its widespread use across various disciplines emphasizes its crucial role in enhancing our understanding of material properties and biological systems. This section will provide a comprehensive overview of the TEM technique's definition, importance, and historical context. By grasping these foundational aspects, one sets the stage for deeper exploration of the operational mechanics, applications, and implications of TEM in scientific inquiry.
Definition and Importance
Transmission electron microscopy is defined as a technique that uses electrons to create high-resolution images of thin samples. It operates differently than light microscopy, as it relies on electrons’ shorter wavelength to achieve better resolution. One of the most critical features of TEM is its capability to visualize structures at the atomic level, which is essential in understanding the material science and biological processes.
The importance of the TEM technique cannot be overstated. It plays a pivotal role in both academic and industrial research. By enabling scientists to analyze materials with unprecedented detail, TEM allows for innovations in various fields, from nanotechnology to pharmaceuticals. The insights gained from TEM studies can lead to the development of improved materials, better drug delivery systems, and a greater understanding of biological mechanisms.
Historical Development
The historical trajectory of the TEM technique is marked by significant milestones. The concept traces back to the early 20th century when physicists first proposed using an electron beam to image samples. However, practical implementation began in the 1930s, thanks to developments in electron optics. The construction of the first transmission electron microscope occurred in 1931 by Ernst Ruska and Max Knoll. This invention was revolutionary, laying the groundwork for future advancements in microscopy.
Throughout the decades, the development of the TEM technique continued rapidly. Innovations such as the introduction of high-voltage electron sources in the 1950s enabled higher resolution imaging. The late 20th century saw the emergence of software that allowed for advanced image analysis, further enhancing the utility of TEM. Today, TEM has evolved to include various specialized forms, such as scanning transmission electron microscopy (STEM), which has expanded its applications significantly.
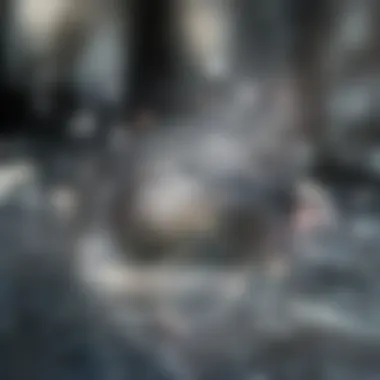
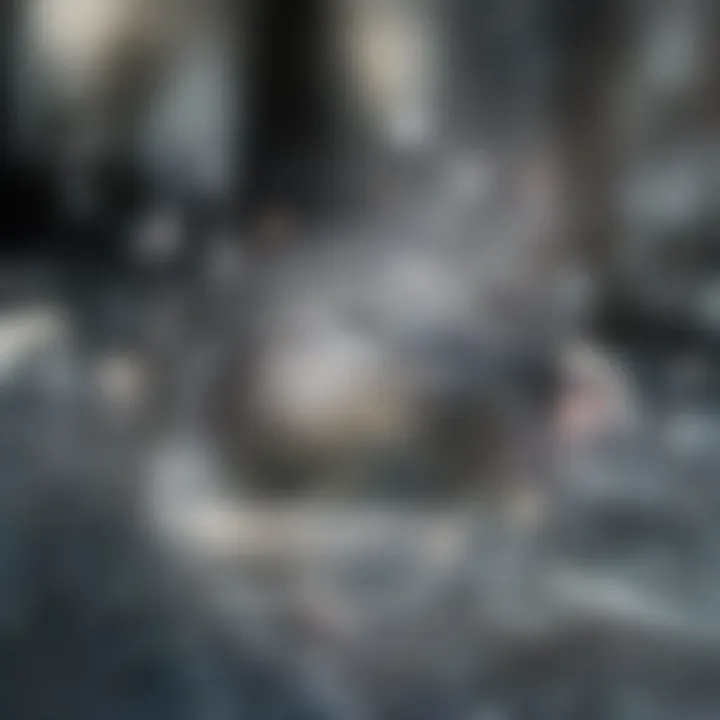
Understanding the definition and historical context of the TEM technique provides essential insight into its relevance and impact. As the following sections unfold, they will delve deeper into the operational mechanics, applications across scientific disciplines, and how this powerful tool continues to shape research and discovery.
Fundamental Principles of the Tem Technique
Understanding the fundamental principles of the Transmission Electron Microscopy (TEM) technique is essential for anyone engaged in the study or application of this powerful imaging method. This section aims to provide a clear foundation that outlines the operational mechanics and underlying theoretical frameworks of TEM. The significance lies in appreciating how these principles contribute to the technique's ability to investigate materials at atomic scales, the versatility it offers across various scientific fields, and the implications of its use for future advancements in research.
Operational Mechanics
The operational mechanics of the TEM technique revolve around its sophisticated imaging processes. In essence, it uses high-energy electrons instead of light to create images of samples. This shift from optical to electron-based imaging is crucial, as electrons possess much shorter wavelengths than visible light, allowing for significantly greater resolution.
The key components of the operating mechanics include:
- Electron Source: The process begins with an electron gun, which emits a stream of electrons. This electrons are accelerated to high energies, typically between 100 to 300 keV.
- Lenses and Condenser System: Lenses are used to focus the electron beam onto the sample. Unlike traditional optical systems, electromagnetic lenses manipulate the path of electrons.
- Sample Interaction: As electrons interact with the sample, they undergo scattering—this can reveal various structures and phases within a material.
- Detector: The transmitted and scattered electrons are collected by a detector, allowing for image formation through digital processing.
TEM can be utilized in several imaging modes, such as bright-field, dark-field, and high-angle annular dark field (HAADF), each serving specific analytical goals, such as identifying crystal structures or evaluating material defects.
Underlying Theoretical Frameworks
The theoretical frameworks that underlie the TEM technique are based upon principles from quantum mechanics and wave physics. Key aspects of these frameworks include how electrons function as both particles and waves, a concept central to the image formation in TEM.
Several theoretical aspects are noteworthy:
- Wave-Particle Duality: Electrons can be treated as waves, allowing for diffraction phenomena that contribute to imaging capabilities. This duality extends usage to electron diffraction analysis, which aids in determining crystallographic information.
- Interaction of Electrons with Matter: When high-energy electrons collide with a sample, they can either be absorbed, transmitted, or scattered. This interaction forms the basis of contrast in the images produced, where different materials respond to electron exposure based on their atomic number and density.
- Fourier Transform: In the context of lens systems, Fourier transforms are used to manipulate images in real-time. This mathematical framework helps in reconstructing images from electron diffraction data, enhancing the clarity and resolution of observations.
Applications Across Scientific Disciplines
The Transmission Electron Microscopy (TEM) technique has become indispensable across a variety of scientific fields. Its importance lies in its ability to provide high-resolution images and detailed information about the micro and nanostructures. This depth of analysis enables researchers to glean insights that are often unattainable through other imaging methods. Understanding the application of TEM across different disciplines can enhance not only the comprehension of structural properties but also the manipulation of materials for various purposes. This section will highlight its significance in biological research, material science, and nanotechnology.
Biological Research
The application of the TEM technique in biological research serves as a powerful tool for visualizing cellular structures at unprecedented resolutions. Researchers utilize TEM to investigate the intricate details of cellular components, such as organelles, membranes, and proteins. For instance, examining the morphology of viruses or the structure of cell membranes can reveal critical insights into function and pathology.
Using TEM, scientists can improve their understanding of disease mechanisms, leading to advances in diagnostics and treatment strategies. The high-resolution imaging allows for the observation of the interactions between biomolecules and their environments in real time. Consequently, TEM establishes itself as a cornerstone in molecular biology studies.
Material Science
In the realm of material science, the TEM technique provides essential insights into the microstructural properties of materials. Industries focused on developing new materials or enhancing existing ones depend on this technique for evaluation purposes. The analysis can include crystal structures, defects, and phase transformations that govern material behavior.
For example, in metallurgy, TEM aids in understanding the effects of temperature and composition on the properties of alloys. This information is fundamental for optimizing material performance in demanding applications, such as aerospace and automotive sectors. Furthermore, the ability to observe nanoscale features can lead to innovations in nanocomposite development, enabling the creation of superior materials.
Nanotechnology
Nanotechnology is another scientific field where the TEM technique plays a critical role. Researchers employ TEM to analyze the properties of nanomaterials to explore their potential applications. This technique is particularly valuable due to its capacity to provide detailed information about size, shape, and distribution of nanoparticles. In nanotechnology, the ability to manipulate materials at the atomic level offers opportunities in various sectors including electronics, medicine, and energy.
For instance, TEM allows scientists to investigate the structural details of carbon nanotubes or quantum dots, assessing their functionality in electronic components or drug delivery systems. As nanotechnology continues to evolve, TEM remains a crucial method in not only understanding nanoscale structures but also in advancing technological applications.
The adoption of the TEM technique across diverse scientific fields illustrates its versatility and effectiveness in enhancing our understanding of complex structures.
In summary, the applications of the TEM technique across biological research, material science, and nanotechnology underscore its significance in modern scientific inquiry. As researchers continue to exploit the capabilities of TEM, the technique will undoubtedly remain at the forefront of advancing knowledge in multiple disciplines.
Benefits of the Tem Technique
The transmission electron microscopy (TEM) technique offers significant advantages within scientific research. Its precise imaging capabilities and extensive applications contribute to its integral role in understanding material properties at microscopic and nanoscopic scales. This section explores the core benefits of the TEM technique and highlights its weaknesses and strengths.
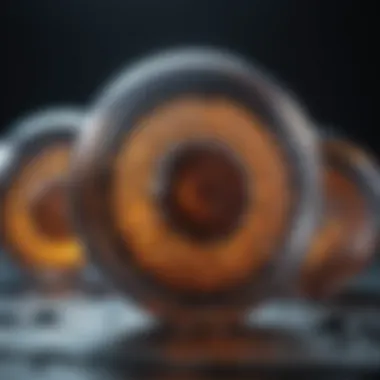
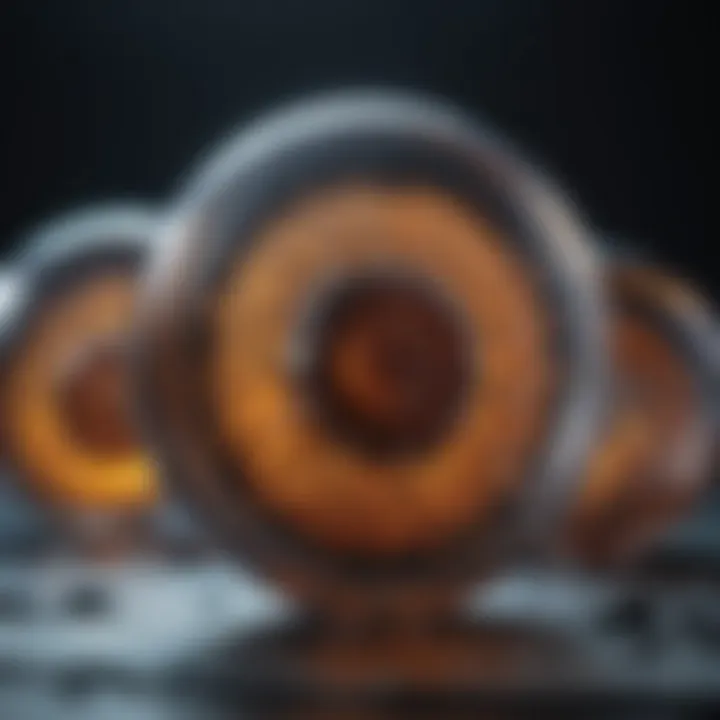
High Resolution Imaging
A primary advantage of the TEM technique is the capability for high resolution imaging. This technology allows researchers to visualize structures at resolutions below one nanometer. The ability to discern fine details in samples is essential in diverse fields, including materials science, biology, and nanotechnology.
High resolution imaging in TEM relies on a focused electron beam that passes through thin specimen slices. This process produces exceptional contrast and detail, revealing the internal structures of materials and biological samples. Unlike optical microscopes, which are limited by the wavelength of visible light, TEM can overcome these constraints due to the shorter wavelength of electrons. Consequently, material scientists and biologists can investigate crystal structures, defect sites, and cell organelles more effectively.
Benefits of High Resolution Imaging:
- Enables structural analysis of materials at atomic levels.
- Provides insights into the arrangement of atoms and defects in crystals.
- Facilitates the observation of cellular components, enhancing biological understanding.
"The high resolution of the TEM technique has opened doors to discoveries in nanotechnology and materials science that were previously unattainable."
Versatility in Applications
The versatility of the TEM technique is another notable benefit. Its applications span various scientific disciplines, making it invaluable in modern research. Researchers utilize TEM in biological studies, materials characterization, and nanotechnology endeavors. The unique adaptability of this technique allows it to tackle diverse scientific questions.
For instance, in biological research, TEM assists in examining cellular structures. The ability to analyze cells at such a detailed resolution aids in understanding complex biological processes and disease mechanisms. In materials science, TEM is used to investigate the properties of new materials, contributing to advancements in engineering and electronics. Nanotechnology also benefits significantly, as TEM provides insights into the characteristics of nanoparticles and nanomaterials.
Key Points on Versatility in Applications:
- Biological Research: Helps in studying organelles, viruses, and bacteria.
- Material Science: Analyzes crystal structures, grain boundaries, and phase transitions.
- Nanotechnology: Characterizes nanostructures, enhancing the understanding of their properties and behavior.
Limitations and Challenges
While the Transmission Electron Microscopy (TEM) technique provides numerous advantages, acknowledging its limitations and challenges is equally important for a balanced understanding. Researchers must navigate various constraints that can affect both the applicability and the outcomes when utilizing TEM in their studies. This section will discuss these limitations, focusing on technical constraints and the intricacies involved in sample preparation, which are crucial for achieving optimal results.
Technical Constraints
The operational efficacy of the TEM technique hinges on specific technical requirements. First and foremost, the high-voltage electron source is essential for achieving the desired resolution. However, this requirement can introduce limits on the types of specimens that can be examined. Samples must be electron transparent, typically less than 100 nanometers thick. As a result, thicker materials—common in bulk samples—often cannot be analyzed directly through TEM. This limitation poses a challenge for researchers aiming to study certain materials without extensive modification.
Moreover, the resolution of TEM imaging depends significantly on the stability of the electron beam and the alignment of optical components. Minor misalignments or instability can lead to distorted images, compromising the quality of data obtained. The requirement for meticulous calibration might dissuade some researchers from utilizing TEM for their studies, especially those who are less experienced with the equipment.
Key Points on Technical Constraints:
- High-voltage requirements restrict sample thickness.
- Sample pre-treatment might not always reflect the natural state.
- Alignment and calibration issues can impair imaging quality.
Sample Preparation Difficulties
Sample preparation is a critical step in the TEM workflow. Preparing a specimen for TEM analysis can be labor-intensive and requires specific techniques to maintain sample integrity. The process often involves cutting, grinding, or polishing the material, each step introducing potential artifacts that can misrepresent the original structure. For example, mechanical preparation can introduce strain or defects, which may not be present in the unprepared sample.
Furthermore, it is crucial to handle biological samples with care, as they can be quite sensitive to the preparatory methods used. Chemical fixation, dehydration, and embedding can alter the fine details of a biological structure. This alteration could result in a misunderstanding of the sample's features and impact subsequent analyses.
In summary, while the TEM technique remains a powerful tool for scientific research, its limitations pose challenges that must be carefully addressed. Researchers must be prepared to invest time and resources into ensuring that their samples are adequately prepared and that their equipment is aligned to achieve the best possible results.
Case Studies and Notable Research
The exploration of case studies and notable research in the context of the TEM technique is vital for understanding its impact and practical applications. These case studies serve as concrete examples, showcasing the diverse ways in which the TEM technique can be harnessed. They illustrate the real-world benefits of this powerful tool, while also highlighting the challenges faced by researchers across various disciplines. The insights gained from these studies contribute to the ongoing development and evolution of the technique, enabling further advancements in scientific inquiry.
Innovative Applications in Biology
The application of the TEM technique in biological research has revolutionized many aspects of life sciences. It allows scientists to examine cellular structures at unprecedented resolutions. For instance, researchers have used the TEM technique to visualize the intricate details of viruses, providing crucial information on their morphology and structure. This leads to better understanding of pathogenesis and aids in vaccine development.
Moreover, TEM is also utilized in the field of neurobiology. By imaging synaptic structures, scientists can investigate how neurons communicate. This knowledge is essential in exploring various neurodegenerative diseases. The detailed images obtained through TEM techniques allow for the identification of abnormalities that would otherwise go unnoticed under traditional imaging methods.
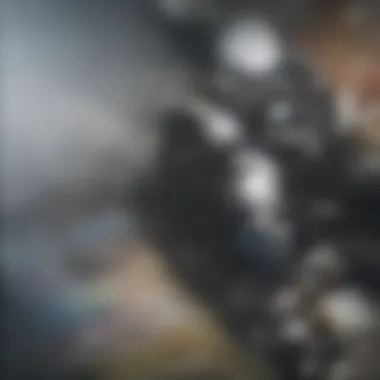
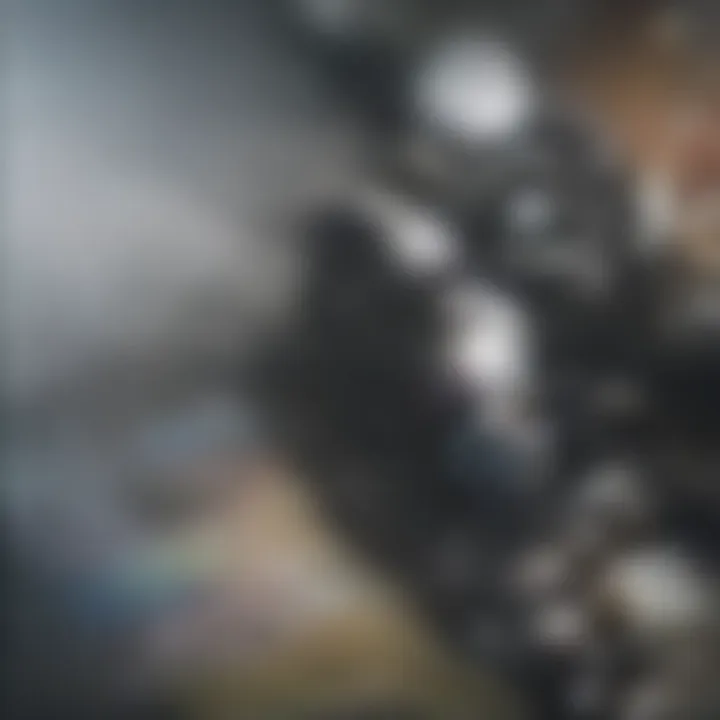
Some notable case studies include:
- Viral Structures: Investigations on the structure of HIV have benefited greatly from TEM, allowing for the visualization of viral envelopes and proteins, leading to insights essential for antiviral drug design.
- Cellular Imaging: The application of TEM to study the ultrastructure of cancer cells has unveiled new targets for therapy. This understanding is crucial for developing effective treatments and enhancing patient outcomes.
Material Characterization Techniques
In material science, the TEM technique plays a crucial role in characterization and analysis of materials at the nanoscale. TEM provides essential information on the crystalline structure, morphology, and composition of materials. For instance, it is commonly used to study semiconductor materials, which are critical in the electronics industry. Understanding defects at the atomic level can help improve the performance and efficiency of electronic devices.
The following aspects are significant when using TEM for material characterization:
- Phase Identification: TEM aids in determining different phases within a material, which is fundamental in tailoring materials for specific applications.
- Elemental Analysis: The technique can identify the distribution of elements at nanoscale, which is vital for advanced material development.
- Nanostructures: The characterization of nanoparticles using TEM helps in understanding their behavior and interactions, informing their use in various applications including drug delivery and catalysis.
In summary, the case studies and notable research surrounding the TEM technique underline its critical role across various scientific fields. The innovative applications in biology showcase how researchers utilize TEM to solve complex biological questions, while the material characterization techniques elucidate fundamental properties of materials at the nanoscale. Collectively, these studies emphasize the necessity and potential of the TEM technique in advancing scientific knowledge.
Future Directions in Tem Research
The exploration of Future Directions in Tem Research is essential to understand where this pivotal technique is headed. As technology and scientific inquiry evolve, the Transmission Electron Microscopy method is poised to adapt and integrate with new advancements, offering even deeper insights into micro and nanostructures. This section outlines emerging technologies and interdisciplinary approaches that promise to reshape TEM applications in the coming years.
Emerging Technologies
Emerging technologies are critical for enhancing the capabilities of the TEM technique. As scientists continue to push the boundaries of what is possible, several key developments are notable:
- Automated Imaging and AI: The incorporation of artificial intelligence in image processing is transforming how electron microscopy images are interpreted. AI algorithms can quickly analyze and classify images, significantly increasing throughput and accuracy without compromising detail.
- In Situ Techniques: The ability to observe samples under operating conditions is invaluable. New in situ TEM capabilities allow researchers to study dynamic processes in real-time. This means observing reactions, phase changes, and other phenomena as they occur, yielding novel insights not available with traditional methods.
- Cryo-EM: Advancements in cryogenic electron microscopy (Cryo-EM) enable the observation of biological samples at near-liquid nitrogen temperatures. This approach allows for the preservation of samples and visualization of structures in their natural state, leading to breakthroughs in molecular biology and materials science.
"The ongoing integration of cutting-edge technologies into TEM will enhance our understanding of material properties at the atomic scale, fostering innovation across multiple disciplines."
By leveraging these emerging technologies, the TEM technique will not only expand its capabilities but will also enable researchers to explore areas previously thought impossible.
Interdisciplinary Approaches
The future of TEM research lies in embracing interdisciplinary approaches. Collaboration between different scientific fields can generate innovative solutions to complex problems. A few areas worth noting include:
- Material Science and Biology Collaboration: By merging insights from biology and material science, researchers can develop new biomaterials with enhanced properties. TEM can provide critical data on the structure and performance of these materials at the nanoscale, informing engineering designs.
- Chemistry and Nanotechnology: A partnership between chemistry and nanotechnology enhances the study of nanoparticles and their interactions. TEM’s ability to characterize these small structures is vital for developing drug delivery systems and catalysts.
- Physics and Electrical Engineering: Innovations in the field of nanoelectronics require precise material characterization methods to improve device performance. Collaborative research between physicists and electrical engineers using TEM can lead to advancements in semiconductor technologies.
Thus, embracing interdisciplinary research and collaboration can strengthen the application of the TEM technique. By pooling expertise and tools from various fields, scientists can tackle challenges more effectively, driving forward our collective understanding of material and biological systems.
End
The conclusion of this article provides a critical summary of the key insights into the Transmission Electron Microscopy (TEM) technique. It serves as a capstone to the discussions surrounding the fundamental principles, varied applications, benefits, limitations, and future directions of TEM research.
In scientific discourse, conclusions are vital. They not only encapsulate essential findings but also provide clarity on the practicality of the subject. This article has outlined that TEM stands out as a powerful tool for imaging and analyzing fine structures at a nanolevel, thus enabling advancements across multiple fields. The synthesis of its historical development with current practices underscores its evolution and relevance.
Summary of Key Points
- TEM provides unparalleled high-resolution imaging crucial for nanoscale analysis.
- Applications span diverse scientific fields including biology, material science, and nanotechnology.
- The versatility of TEM makes it suitable for various research endeavors, despite inherent limitations such as sample preparation challenges.
- Emerging technologies are reshaping the landscape of TEM, allowing for enhanced methodologies and techniques.
Overall, the comprehensive review of TEM highlights its significant role in modern scientific exploration.
The Impacts of the Tem Technique
The impacts of the TEM technique are vast and multi-dimensional. Firstly, it has revolutionized the way researchers visualize and understand materials at the atomic level. This capability has led to breakthroughs in various industries, from pharmaceuticals to electronics, showcasing the integral role TEM plays in advancing knowledge.
Moreover, the interdisciplinary approach observed in TEM research fosters collaboration between scientists from various fields, thereby enhancing innovation.
"The capability to examine structures at microscopic levels encourages deeper exploration beyond mere surface understanding, pushing the boundaries of scientific inquiry."
Importantly, as TEM technology progresses, so does its potential influence on future scientific discoveries. Researchers can anticipate not only improved resolution and speed but also expanded applicability in complex studies such as dynamic systems or molecular interactions. This ongoing evolution positions TEM as a key instrument in leading future advancements, both theoretically and practically.
By examining the profound effects of the TEM technique on scientific inquiry, one gains a deeper appreciation for its significance and the continuing innovations that arise from its application.