Exploring the Silicon Probe: In-Depth Insights
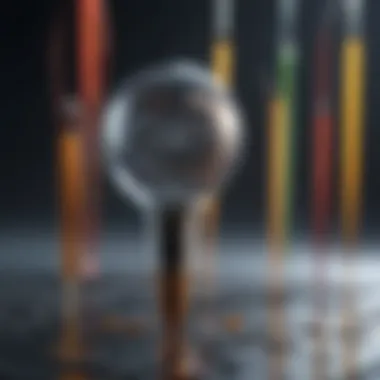
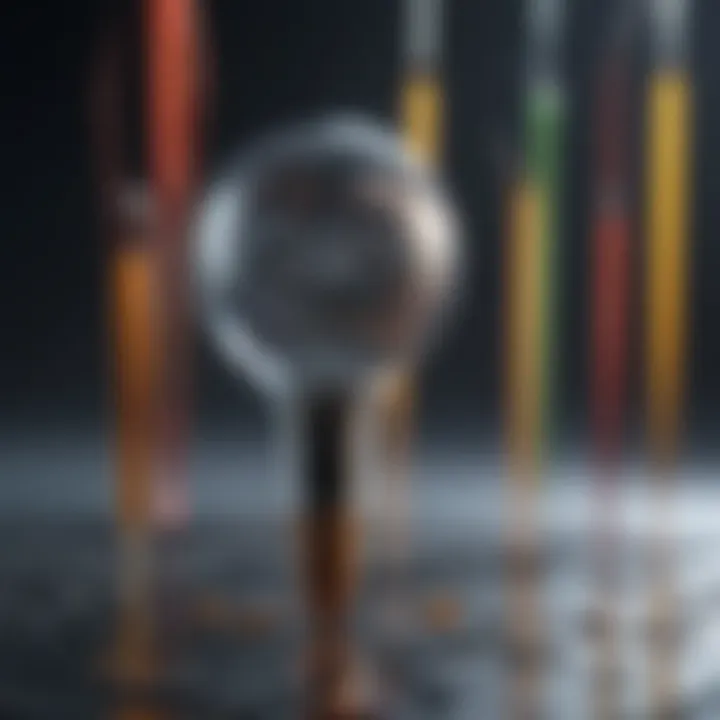
Intro
Silicon probes have emerged as a crucial tool in neuroscience and engineering. These devices provide researchers with precise data collection capabilities, facilitating in-depth exploration of neural activity. Understanding silicon probes requires a grasp of their design, function, and application. This guide aims to present a clear overview of these instruments, highlighting key advancements and practical relevancy in scientific research.
The evolution of silicon probes reflects broader trends in both neuroscience and engineering. As technology progresses, the methods to gather and analyze data have also advanced. This article will delve into the methodologies employed in the study of silicon probes, drawing connections between historical and contemporary techniques.
Furthermore, the discussion will explore how silicon probes compare to earlier research methodologies, providing insight into their unique contributions and theoretical implications. By engaging with both past research and future potential, we highlight the trajectory of this field and the implications for various disciplines, including biology and medical sciences.
In this exploration, we aim to address the intricacies of silicon probes while considering their impact on current and future scientific endeavors.
Understanding Silicon Probes
Silicon probes serve as an essential tool in the domains of neuroscience and biomedical research. By enabling precise electrical recording of neuronal activity, these instruments facilitate a deeper understanding of brain functions. In examining the design, material composition, and functionalities of silicon probes, one can appreciate their pivotal role in advancing both academic study and clinical practice.
Silicon probes open a gateway to intricate brain networks by providing high-resolution data collection methods. This aids researchers in mapping neural circuits, studying cellular interactions, and developing therapeutic strategies. Through this overview, a comprehensive foundation will be established to understand both their historical genesis and contemporary significance.
Definition and Basic Principles
A silicon probe is a device made primarily from silicon, engineered to penetrate biological tissues and record electrical signals from neurons. These probes can vary greatly in shape, size, and configuration, depending on their intended use. The fundamental principle of operation is based on the transduction of ionic currents into electrical signals. Essentially, when neurons fire, they generate ionic currents that silicon probes capture. The conversion of these signals leads to valuable data concerning neuronal behavior and interactions.
The construction of silicon probes requires an understanding of semiconductor physics. By harnessing the electrical properties of silicon, researchers can design probes that offer high sensitivity and selectivity. Factors such as electrode spacing, material properties, and surface topology play critical roles in determining performance.
Historical Development
The development of silicon probes traces back to advancements in materials science and microfabrication techniques. Early iterations emerged from a blend of traditional electrode technology and innovations from the semiconductor industry. The 1970s and 1980s saw the advent of microelectrode arrays, which laid the groundwork for silicon probes as we know them.
As researchers sought to enhance spatial resolution and target specificity, more sophisticated designs evolved. The integration of silicon carbide and various coatings improved biocompatibility and reduced tissue damage during implantation. The evolution of silicon probe technology has not only influenced research methodologies but also revolutionized areas such as brain-machine interfaces. Each advancement represents a leap forward in both capability and application, paving the way for future exploration in this fascinating field.
"Silicon probe technology represents a convergence of neurology, engineering, and materials science, providing researchers with unmatched insights into the functioning of the brain."
The Design of Silicon Probes
The design of silicon probes is a critical factor that influences their performance and application in various scientific fields. Probes must be engineered to achieve specific objectives such as high sensitivity, minimal invasiveness, and the ability to facilitate complex data acquisition processes. This section discusses the fundamental elements of silicon probe design, emphasizing material composition, geometric configurations, and size and scalability considerations.
Material Composition
Silicon probes are primarily composed of silicon, a material that is favored for its semiconductor properties. Silicon allows for the integration of electronic components, making it a versatile choice for neuroprosthetics and other applications. Additionally, the doping of silicon can enhance its electrical characteristics, which is crucial for establishing reliable electrical connections with biological tissues.
Other materials, such as polymers and metals, may be incorporated to improve the mechanical properties and biocompatibility of the probes. Consideration of biocompatibility is vital, as it determines the potential for irritation or rejection by the host organism's immune system. Probing materials must therefore be selected based on their chemical stability and compatibility with biological environments. The balance between electronic performance and biological safety is a key consideration in the design process.
Geometric Configurations
The geometric layout of silicon probes plays a significant role in their effectiveness. These configurations can vary widely based on the intended applications. For instance, tapered designs allow for easier insertion into tissues, while branched configurations enable multiple sites of data collection simultaneously. As more intricate arrangements are designed, probes can accommodate dense arrays of electrodes, which improves the spatial resolution of recordings made from neural tissues.
Achieving an optimal geometry not only enhances performance but also impacts the ability to reach targeted brain areas. Research indicates that specific geometries can help reduce tissue damage and inflammation at the insertion site, aiming to create a more favorable environment for long-term recordings. Therefore, it is essential to analyze the trade-offs between different shapes and layouts during the design phase.
Size and Scalability Considerations
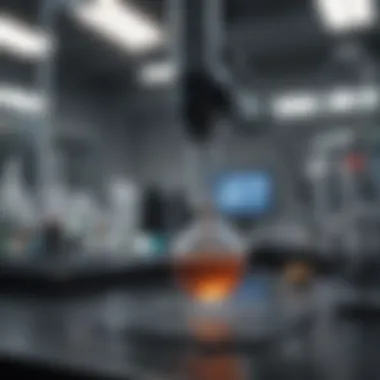
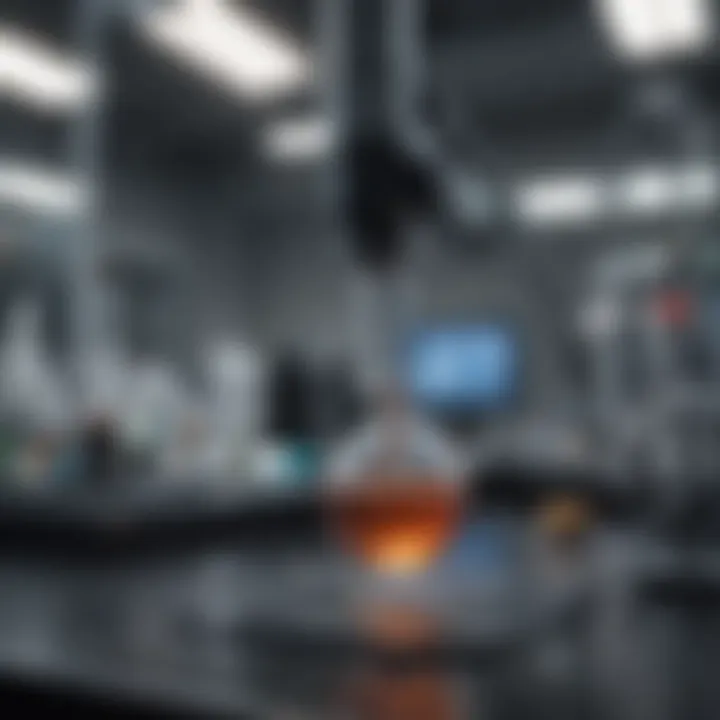
Size is another essential aspect of silicon probe design. Probes must be small enough to minimize tissue damage but large enough to house the required electronic components. The development of nanoscale technologies has made it possible to create smaller probes that can provide high-resolution recordings without significant invasiveness. Conversely, larger probes may offer more capabilities for complex data acquisition but may also present challenges, such as increased tissue trauma.
Scalability is particularly pertinent when designing probes for large-scale applications, like multielectrode arrays. The ability to produce larger numbers of probes consistently remains a critical factor for researchers looking to conduct large-scale studies in neuroscience. This emphasizes the need for manufacturing processes that maintain high yields and reliability across a wide range of sizes. A collaborative approach between engineers and neuroscientists can help ensure that designs are responsive to the requirements of specific research objectives.
"The advancement of silicon probe technology depends significantly on innovative design practices that address both electronic performance and biological compatibility."
In summary, the design of silicon probes involves multiple considerations that interplay in complex ways. Material composition, geometry, and size are interdependent factors that can influence the efficiency and effectiveness of these devices in real-world applications. Thorough understanding and meticulous planning are crucial for developing silicon probes capable of meeting the rigorous demands of modern neuroscience research.
Functionality of Silicon Probes
The functionality of silicon probes forms a critical pillar in understanding their role in neuroscience and engineering. These devices are designed to facilitate the acquisition, processing, and analysis of neural data, enabling researchers to decode and interpret complex biological signals. Their sophisticated design allows for high-resolution measurements, essential for detailed insights into neuronal activity. Employing silicon probes can significantly enhance the capabilities of neuroscience research, offering distinct advantages alongside some considerations that must be addressed.
Data Acquisition Techniques
Data acquisition techniques are fundamental in capturing the neural signals that silicon probes encounter. The primary technique employed is multi-electrode recording, where multiple electrodes within a single probe can simultaneously monitor neuronal activity.
This ability allows for:
- High temporal resolution: Researchers can track fast changes in neural signals, providing insights into dynamics of brain activity.
- Spatial resolution: The arrangement of electrodes enables detailed mapping of activation across various neuronal populations.
- Real-time data collection: Continuous recording of neural signals facilitates dynamic studies in live subjects.
Silicon probes also leverage advanced technologies such as integrated circuits for efficient signal amplification. This integration minimizes the noise typically associated with electrical signals, improving the quality of data captured. Researchers employ various methods, including spike sorting algorithms, to analyze these signals, discerning significant events from background noise.
Signal Processing Methods
After data acquisition, effective signal processing is vital to extract meaningful information from the recorded signals. The processing techniques used with silicon probes can significantly influence the interpretation of neuronal activity. Common methods include:
- Filtering: This initial step reduces noise and maximizes the clarity of the data. Bands of interest can be isolated to focus on specific signal types.
- Spike detection algorithms: These are crucial in identifying action potentials, helping to transform continuous voltage signals into discrete neuronal events for further analysis.
- Feature extraction: Key characteristics of the spikes, such as amplitude and temporal patterns, are quantified, enriching the dataset and assisting in classification efforts.
- Statistical analysis: Advanced techniques, such as machine learning, can be applied in some instances to model complex relationships within the data. This can reveal patterns that are not immediately obvious, facilitating deeper insights.
"The efficiency of signal processing methods is about maximizing the extraction of relevant data while minimizing disruption from noise."
Applications in Neuroscience
Silicon probes have become a significant tool in neuroscience, providing insights that were previously difficult to obtain. The importance of these applications lies in their ability to capture complex neural activity with precision. Various specific elements come into play, including the technology's adaptability to different experimental setups, its scalability, and the accuracy of data collection. Thus, understanding these applications can lead to advances in both fundamental research and clinical practices.
Single-Cell Recording
Single-cell recording is a technique that utilizes silicon probes to measure the electrical activity of individual neurons. This method is vital for studying the mechanisms underlying neural signaling. By placing a silicon probe close to a neuron, researchers can detect action potentials and synaptic events. The high temporal resolution provisions enabled by silicon probes allow for monitoring rapid physiological changes. This precision is crucial when exploring individual neuronal responses under various conditions.
Moreover, single-cell recording facilitates in-depth analysis of cellular properties and neuron interactivity. This can help in understanding disease mechanisms, providing a pathway for targeted therapies in conditions like epilepsy or neurodegenerative disorders.
Multielectrode Arrays and Neural Networks
Multielectrode arrays (MEAs) represent another pivotal application of silicon probes in neuroscience. These arrays contain multiple recording sites that can monitor the activity of many neurons simultaneously. This capacity is instrumental for studies focused on neural networks and their intricate connections.
The primary advantage of MEAs lies in their ability to offer a comprehensive view of neural dynamics. By analyzing large datasets collected from these arrays, researchers can identify patterns that dictate how networks respond to stimuli, learn, and adapt. This can be particularly useful in illuminating the pathways involved in learning processes or in investigating the neuronal basis of behavior.
Brain-Machine Interfaces
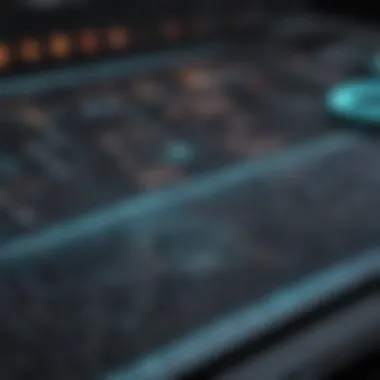
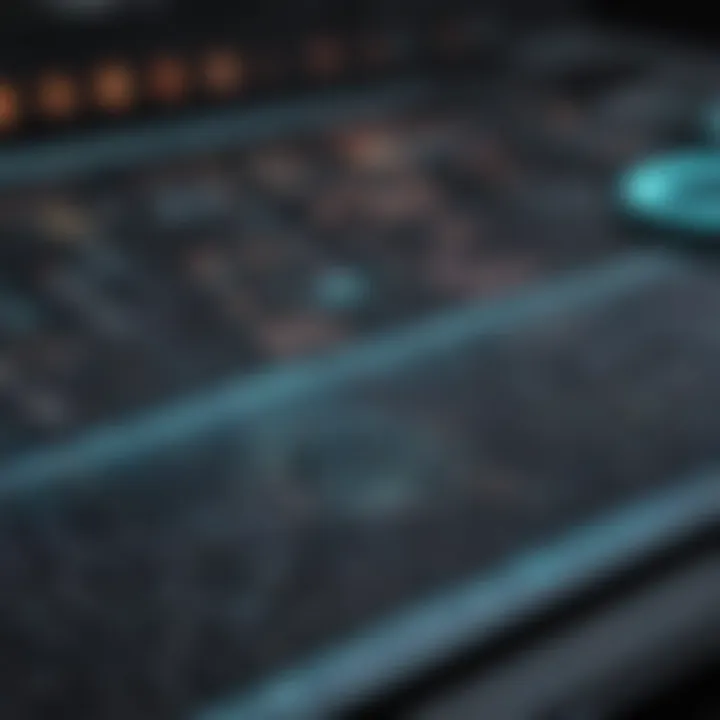
Brain-machine interfaces (BMIs) symbolize one of the most groundbreaking applications of silicon probes. These technologies aim to establish direct communication pathways between the brain and external devices. By using silicon probes to record neuronal activity, BMIs can interpret brain signals and translate them into commands for machines, such as prosthetics or computer interfaces.
The advancements in silicon probe technology have significantly enhanced BMI performance, particularly regarding signal fidelity and processing speed. However, challenges remain in ensuring biocompatibility and minimizing the immune response to implanted devices. Research continues to focus on improving these interfaces to allow for seamless integration between the brain and technology, ultimately aiming to restore lost functionalities in patients.
"Silicon probes are essential for unlocking the communication between the brain and technology, offering new avenues for rehabilitation and enhancement of human capabilities."
Advancements and Innovations
Advancements in silicon probe technology are crucial for enhancing their capabilities in neuroscience and related fields. As researchers unravel the complexities of the brain, the integration of silicon probes with various technologies plays a pivotal role in acquiring high-quality data. Progress made in this domain not only improves the effectiveness of experiments but also facilitates new discoveries. Through innovative techniques and applications, silicon probes are becoming indispensable tools in modern neural research.
Integration with Emerging Technologies
The integration of silicon probes with emerging technologies is reshaping the landscape of neuroscientific research.
- Optogenetics: This method allows researchers to control neurons with light, combining optical methods with silicon probes to stimulate and record neural activity simultaneously. The resulting data can show how neural circuits behave under specific conditions.
- 3D Printing: Custom probes can be fabricated using 3D printing techniques, providing tailored solutions for unique experimental setups. This flexibility leads to better fit and functionality in diverse biological tissues.
- Wearable Devices: The shift towards wearable technology is significant. Silicon probes are being integrated into wearable devices allowing real-time monitoring of neural activity in natural settings. This development paves the way for studies on behavior and neurological conditions in everyday life.
"Emerging technologies offer a new dimension to the capabilities of silicon probes, allowing for versatile applications across various research fields."
These integrations not only enhance data acquisition but also expand the scope for multidisciplinary research that combines neuroscience, engineering, and medicine.
Software Developments for Analysis
In parallel with hardware advancements, software developments are equally important for the utility of silicon probes. The analysis of large datasets generated from silicon probes requires sophisticated software tools.
- Machine Learning: Algorithms help in identifying patterns from the massive amounts of data collected. Machine learning models can classify neural signals, identifying correlations that might be missed by traditional analysis methods.
- Data Visualization Tools: Advancements in visualization software allow researchers to interpret complex data more effectively. Graphical representations of neural activity can provide clearer insights into brain function and behavior.
- Open Source Platforms: Tools such as Open Ephys and Neurophysiological Systems are contributing to an open-source revolution in data analysis. By providing accessible platforms, researchers can share findings and collaborate more efficiently.
By focusing on software development, researchers not only facilitate enhanced analytical capabilities but also foster an environment of shared knowledge and innovation.
Challenges in Silicon Probe Research
Research utilizing silicon probes is essential, but it is not without its share of challenges. These challenges influence the effectiveness and applicability of silicon probes in various environments. Understanding these issues is vital for researchers and practitioners in the field. Addressing these hurdles can open avenues for advancements in technology and better experimental outcomes. Below are some major challenges that researchers face today.
Limitations in Spatial Resolution
One of the foremost challenges in silicon probe research is the limitation in spatial resolution. Silicon probes must strike a balance between density and spatial precision. While increasingly dense arrays can record from far more neurons simultaneously, they can suffer from issues ensuring precise localization of individual neural signals. In high-density arrangements, signals may be mixed or overlapped, leading to ambiguous data interpretation.
The demand for enhanced spatial resolution drives research towards higher-density silicon probes. However, there exist physical constraints within the material and design. Researchers continuously look for methods to refine spatial resolution while maintaining the ability to capture multiple signals.
Biocompatibility Issues
Another significant concern is biocompatibility. When silicon probes are introduced into living tissue, they must not provoke an adverse immune response. The body might react negatively to the foreign material, which can lead to inflammation and tissue damage. Consequently, this can inhibit accurate data acquisition.
To enhance biocompatibility, ongoing research focuses on materials and coatings that reduce immune responses. These adaptations are crucial for facilitating long-term implantation and recordings. Innovations in coating technologies and alternative materials provide potential solutions to this challenge. A more extensive focus on natural and synthetic polymers may help in making probes more bihoconpatible.
Data Overload and Processing Concerns
Silicon probes generate large volumes of data, resulting in possible data overload. The ability to record from multiple sites may yield significant amounts of information, which can be overwhelming. This data management issue complicates further analysis. Often, the challenge lies not just in collecting the data but also in effectively processing and interpreting the vast troves of information.
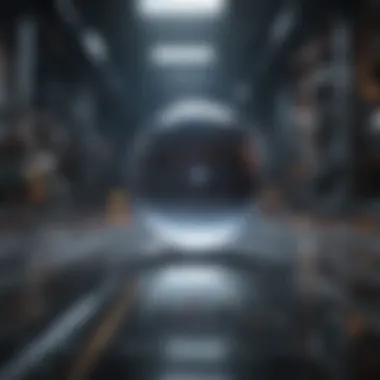
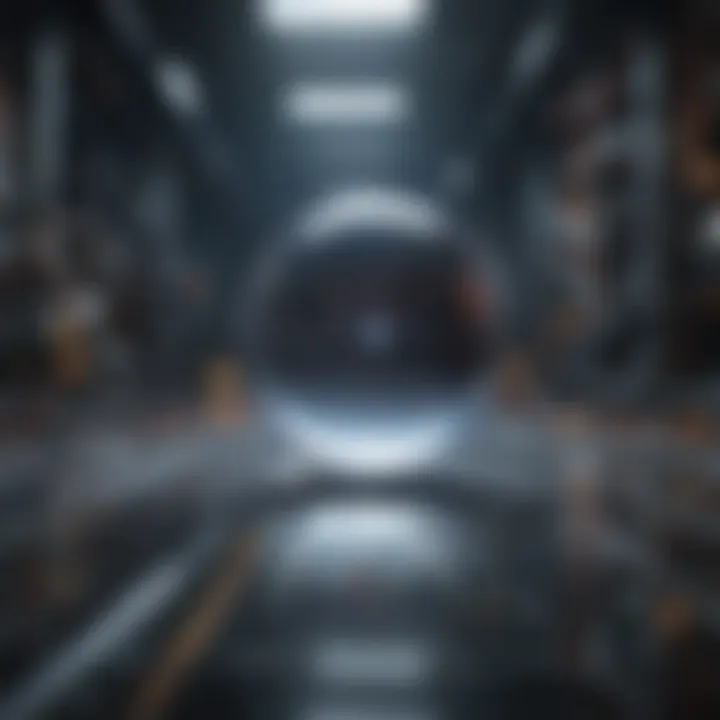
Researchers need to develop algorithms for real-time data processing and analyses. Tools that can distill essential information without losing critical details are of utmost importance. While advanced machine learning techniques show promise, challenges remain in their implementation and efficacy in varied research environments.
The future of silicon probe applications largely depends on overcoming these challenges, as well as further enhancing their capabilities.
Future Directions
In the field of silicon probes, exploring the future directions will be essential to understanding their evolving role in scientific research. The advancements in silicon probe technology open new avenues not only for neuroscience but also for regenerative medicine. This section discusses potential applications, ethical considerations, and the implications for future research and development.
Potential in Regenerative Medicine
Silicon probes show promising potential in regenerative medicine. Their ability to interface seamlessly with neural tissue allows for better understanding of cellular behavior, which can lead to breakthroughs in treatment strategies. For example, they can be used for precise stimulation of neural cells, potentially aiding in nerve regeneration and recovery from injuries.
The integration of silicon probes in regenerative medicine could facilitate real-time monitoring of cellular responses and guide tissue engineering. This could improve the outcomes of therapies aimed at repairing damaged organs or tissues.
Innovative approaches, such as hybrid models combining biological materials with silicon probes, are gaining attention. Such models can provide insights into processes at the cellular level, accelerating the development of regenerative techniques.
It is important to consider that while silicon probes can significantly enhance regenerative medicine, research needs to solidify their effectiveness and reliability in clinical settings.
Ethical Considerations
As silicon probes become more integrated into biological systems, ethical considerations will arise. The implications of using silicon probes raise questions about the boundaries of scientific research and the moral responsibilities researchers hold toward living subjects.
Informed consent will be crucial for studies involving silicon probes. Participants must understand the risks, benefits, and purpose of experiments. Clarity and transparency in communication are essential to maintain trust in research.
Moreover, researchers must address concerns related to biocompatibility and long-term effects of implanting silicon probes in living organisms. Guidelines and regulations should be established to ensure safe and ethical use of this technology.
"The use of technology in medicine must be accompanied by a moral obligation to protect patient rights and well-being."
Lastly, interdisciplinary dialogue among ethicists, engineers, and medical professionals can foster responsible innovation. This collaborative approach can guide future studies, ensuring the responsible advancement of silicon probe technology.
In summary, future directions in silicon probe research are rich with potential both in regenerative medicine and ethical considerations. As technology progresses, researchers must navigate these realms thoughtfully to maximize benefits while minimizing ethical dilemmas.
Culmination
The conclusion serves as a significant part of any scholarly article, and in this case, it encapsulates the essence of exploring silicon probes. Silicon probes are not just tools; they represent a convergence of science and technology. Their carefully designed structure and versatility in functionality provide substantial contributions to the fields of neuroscience and engineering.
A few key elements stand out when we summarize the insights regarding silicon probes:
- Multifunctionality: Silicon probes excel in various applications from single-cell recording to intricate brain-machine interfaces. This versatility enhances their value in diverse research contexts.
- Innovation: Continuous advancements in material composition and data acquisition techniques promise to further refine their capabilities. Such innovations can drive new research avenues and expand their applications.
- Addressing Challenges: Highlighting the limitations and issues surrounding silicon probes underscores the importance of ongoing research to address these concerns. With every challenge identified, solutions emerge, paving the way for improved technology.
- Future Potential: The exploration of silicon probes does not stop with current applications. There is vast potential in regenerative medicine and ethical implications that need careful consideration, indicating that this field will continue to evolve.
- Impact on Research: By enabling unprecedented data collection and analysis, silicon probes are setting new standards in neuroscientific inquiry, influencing future studies and medical practices.
As discussed throughout the article, the journey into the world of silicon probes reveals their profound implications. Researchers, educators, and professionals in the respective fields should keep an eye on these developments. As the technology advances, its impact will be felt across various aspects of biological and medical research.
Ultimately, understanding silicon probes enriches our comprehension of the interplay between engineering and neuroscience and opens up possibilities for future discoveries.
"The future of silicon probes holds promise for revolutionizing how we understand and explore the brain's complexities."
Summary of Key Insights
In summary, the article highlights essential aspects of silicon probes, detailing their design, functionality, and diverse applications. Important points include:
- The historical context that has shaped the current state of silicon probes.
- The design considerations that ensure optimal performance in neural recordings.
- The various applications across neuroscience, facilitating significant advancements in understanding brain activities.
- Recent innovations that integrate advanced technologies and software developments, enhancing data interpretation.
- Ongoing challenges that need addressing to unlock further potential in this field.
- Future directions that include ethical considerations and potential use in regenerative medicine.
These insights not only consolidate the information provided throughout the article but also emphasize the critical role that silicon probes play in advancing our understanding of neuroscience and engineering.