Exploring the Complexities of Plasmid Vector Maps
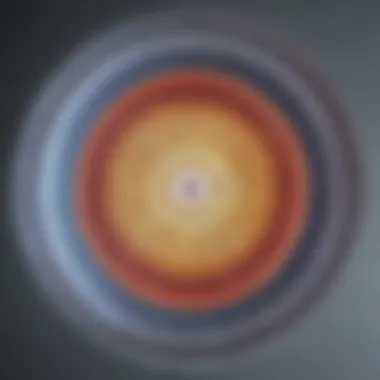
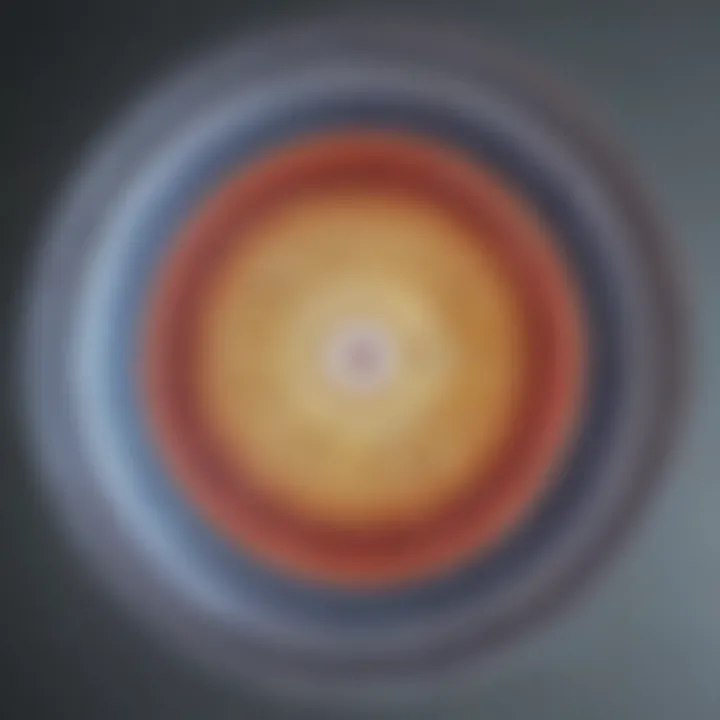
Intro
Plasmid vector maps serve as crucial tools in the fields of molecular biology and genetic engineering. These visual representations encapsulate vital information about plasmid DNA, including its structure, function, and the essential components that allow for genetic manipulation. Comprehending plasmid vector maps is an essential step for those involved in research, biotechnology, and genetic engineering, as they provide clarity on how these vectors function in various applications.
Overview of Plasmid Vectors
Plasmids are small, circular DNA molecules that are separate from the chromosomal DNA in bacterial cells. They have the ability to replicate independently and often carry genes that provide some advantageous traits, such as antibiotic resistance. The plasmid vector is a modified version of these plasmids and is designed for specific purposes, such as cloning, gene expression, or DNA manipulation.
Plasmid vector maps visually depict the arrangement of various components within the plasmid, making it easier for researchers to understand how to implement these vectors in laboratory techniques. Key features, such as the origin of replication, selectable markers, and restriction enzyme sites, are prominently displayed in these maps, allowing scientists to select appropriate conditions for their experiments.
As we delve into this comprehensive examination of plasmid vector maps, we will explore the methodologies that have been developed for their creation and use. We will also consider the critical discussions surrounding their application, including implications in both theoretical and practical settings.
Foreword to Plasmids
Plasmids are small, circular, double-stranded DNA molecules found in many bacteria and some eukaryotic organisms. They exist independently of chromosomal DNA and can replicate autonomously. This characteristic makes them invaluable tools in genetic engineering and molecular biology. Understanding what plasmids are and how they function is critical for students, researchers, and professionals in the field.
The importance of plasmids lies in their role as vectors for genetic material. They can carry genes of interest into host cells, allowing for the manipulation and study of those genes. This ability enables a myriad of applications ranging from gene cloning to the production of proteins for pharmaceuticals. Because of plasmids, scientists can explore genetic functions and interactions that would be challenging to examine using chromosomal DNA alone.
Additionally, plasmids can confer selective advantages to bacteria, such as antibiotic resistance. This functionality highlights their evolutionary significance and the potential consequences of their transfer between organisms. The insights gained from studying plasmids contribute to our understanding in various disciplines, including biotechnology, medicine, and microbiology.
In summary, plasmids are essential components of genetic manipulation tools, enabling innovative research and applications in numerous fields. Grasping their characteristics, behaviors, and uses is foundational for anyone involved in modern biological sciences. This section sets the stage for a deeper exploration of plasmid vector maps, offering a stepping stone into more complex concepts that shape current molecular techniques.
Understanding Vector Maps
Vector maps are foundational tools in molecular biology, especially in the field of genetic engineering. They serve as detailed representations of plasmid vectors, illustrating their important structural components. By understanding vector maps, researchers can efficiently design and manipulate plasmids for various applications.
Importance of Vector Maps
Vector maps offer crucial insights into the arrangement and function of different elements within a plasmid. Each component plays a specific role, and the clarity provided by the vector map is invaluable for both novice and experienced scientists. The benefits include:
- Visual Reference: Vector maps provide a graphical representation that simplifies complex information. Researchers can quickly identify features such as origins of replication, selectable markers, and cloning sites, aiding in experimental planning.
- Error Reduction: Detailed vector maps help in minimizing errors during plasmid construction and manipulation. A well-labeled map can guide the selection of appropriate restriction enzymes, ensuring that cloning steps are executed correctly.
- Communication: Vector maps facilitate discussion and collaboration among researchers. Sharing a common visual tool allows for easier communication of ideas and findings within the scientific community.
Specific Elements in Vector Maps
The comprehension of vector maps requires attention to certain essential components:
- Origin of replication: This sequence is crucial for the plasmid’s replication within a host cell. Without it, the plasmid cannot propagate, making it a key feature in vector design.
- Selectable markers: These are often antibiotic resistance genes that allow for the identification of successfully transformed cells. This feature is particularly important in gene cloning and transformation experiments.
- Multiple cloning sites (MCS): The MCS is a short region containing several restriction enzyme sites, allowing researchers to insert foreign DNA into the plasmid easily. The design of this site is critical for flexibility in cloning procedures.
- Promoter regions: These regions control the expression of downstream genes. They are essential for ensuring that the desired protein is produced at the right levels once the plasmid is introduced into cells.
Understanding these components enhances the ability to create effective plasmids tailored for specific research objectives. Knowing how to read and interpret vector maps not only promotes successful experimental outcomes but also advances knowledge in genetic manipulation.
"A well-understood vector map transforms the daunting task of plasmid design into a systematic and manageable process."
Considerations
When working with vector maps, researchers must consider factors such as:
- Compatibility with host systems: The plasmid must be appropriate for the host organism, ensuring that the genetic elements function as intended.
- Construction techniques: Techniques like restriction enzyme cloning or Gibson assembly are influenced by vector map design, impacting the efficiency and success of plasmid creation.
By prioritizing an understanding of vector maps, researchers enhance their capability to innovate within the fields of genetic engineering and synthetic biology. The significance of accurately interpreting these maps cannot be overstated, as it informs critical decisions throughout the experimental workflow.
Key Features of Plasmid Vector Maps
Plasmid vector maps are indispensable tools in the realms of molecular biology and genetic engineering. Understanding their key features allows researchers and students to optimize plasmid design and execution in various applications. Each component within a plasmid vector map plays a crucial role in ensuring the successful cloning and expression of genes. Here, we delve into the essential elements that characterize these influential maps.
Origin of Replication
The origin of replication (ori) is a fundamental feature of plasmid vector maps. It determines the starting point for DNA replication within the host cell. By defining this region, researchers can control the copy number of the plasmid in the bacterial host. High copy number plasmids are often desirable for cloning where abundant DNA is required. Conversely, low copy number plasmids can be beneficial for maintaining the stability of inserted sequences.
Implications for cloning:
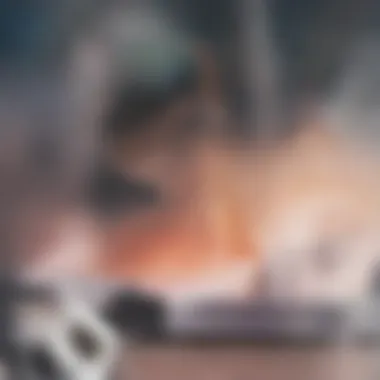
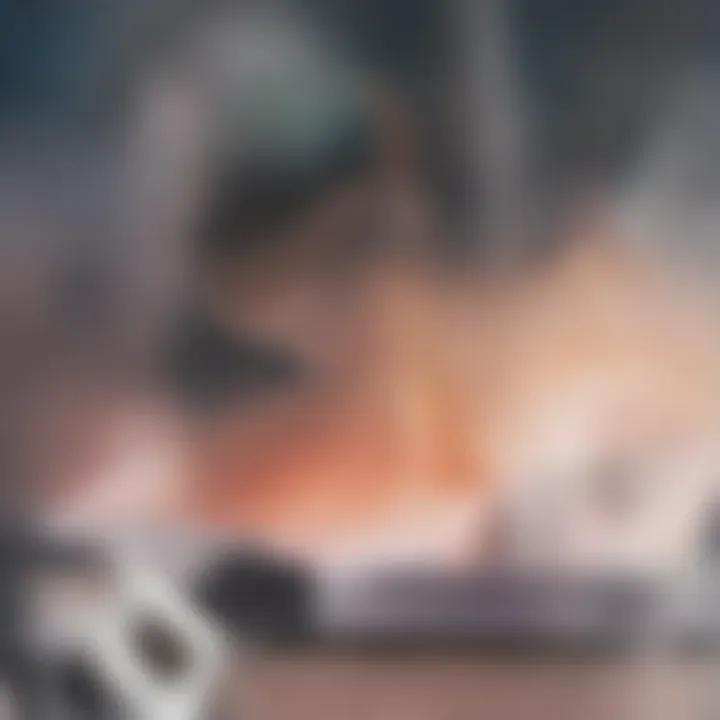
- High copy number leads to increased gene product yield.
- Low copy number can minimize potential toxic effects of overexpression.
Presence and choice of ori depend on the target organism. For E. coli, the pBR322 ori is common, while the ColE1 ori can be ideal for applications needing lower plasmid yields.
Selectable Markers
Selectable markers are crucial for filtering transformed cells. These genes confer resistance to specific antibiotics or allow the identification of successfully transformed cells. Their importance lies in streamlining the identification process of cells that incorporated the plasmid. Common selectable markers include the Ampicillin resistance gene (bla) and Kanamycin resistance gene (kan).
Using a selectable marker, researchers can:
- Ensure only cells that have taken up the plasmid survive in the presence of the antibiotic.
- Quickly determine successful transformations by observing growth patterns.
The choice of selective markers must align with the experimental conditions and cannot cross-react with the antibiotic used.
Multiple Cloning Sites
Multiple cloning sites (MCS), also known as polylinkers, are short segments within the plasmid vector that contain several unique restriction sites. These sites facilitate the insertion of foreign DNA. The MCS allows for flexible insertion of different genetic sequences, thereby enhancing the utility of the plasmid across various applications.
Benefits of having MCS include:
- Versatility: Different enzymes can be used based on the DNA to be cloned.
- Increased ease of cloning: Researchers can choose the best restriction sites for their sequence insert.
It is essential that the chosen restriction enzymes do not cut within essential plasmid features such as the ori or selectable markers, to maintain plasmid functionality after the insertion.
Promoter Regions
Promoter regions are integral for driving gene expression. These regions dictate the transcription of the downstream gene, influencing when and how much of the gene product is produced. In plasmid vector maps, promoters can be either constitutive or inducible, providing flexibility in experimental design.
Considerations regarding promoter choice include:
- Constitutive promoters lead to constant expression, suitable for genes that need to be expressed continuously.
- Inducible promoters allow researchers to control gene expression through specific conditions, optimizing experimental timing.
Some common promoter sequences include the T7 promoter and lac promoter, which are widely used in various expression systems. Understanding the role of promoter regions enhances the ability to tailor plasmid functionality for specific applications.
The key features of plasmid vector maps are critical in defining their utility in genetic engineering and research.
The Role of Plasmids in Genetic Engineering
Plasmids play a crucial role in genetic engineering, acting as versatile tools for manipulating genetic material. These small, circular, double-stranded DNA molecules can replicate independently of chromosomal DNA within a host cell. This unique characteristic enables scientists to insert foreign genes into plasmids, facilitating the study and alteration of genetic functions in various organisms.
One major benefit of using plasmids is their ability to introduce specific genes into host cells, which can lead to the expression of desired traits. For instance, researchers can use plasmids to create genetically modified organisms (GMOs) that possess advantageous characteristics such as pest resistance or enhanced nutritional content. Moreover, plasmids allow for the replication and expression of therapeutic proteins, which has profound implications for medicine, including in the production of insulin for diabetes treatment and monoclonal antibodies for various diseases.
Here are some specific elements regarding the role of plasmids in genetic engineering:
- Gene Delivery Systems: Plasmids are often employed in gene delivery systems, providing a method for introducing engineered genes into various cell types. This can be done in different organisms such as bacteria, plants, and animal cells.
- Marker Genes: Many plasmids carry selectable marker genes that enable researchers to identify and isolate cells that have successfully taken up the plasmid. This is crucial for confirming successful genetic modifications.
- Versatility in Applications: Plasmids are employed in diverse applications, including cloning, gene therapy, and vaccine development. Their adaptability makes them invaluable in both research and commercial biotechnology.
However, the use of plasmids is not without its considerations. For example, retaining the stability of the plasmid within the host can be challenging, and loss of the plasmid may occur, especially if selective pressure is not maintained. Furthermore, the introduction of plasmids can lead to undesired effects in host organisms if not carefully controlled.
In summary, plasmids are fundamental components in the toolbox of genetic engineering. They facilitate the introduction of new genetic material into organisms, enabling significant advancements in fields such as agriculture and medicine. As our understanding of plasmid technology continues to evolve, the potential for innovation in genetic research and applications remains significant.
Diversity of Plasmid Vectors
The diversity of plasmid vectors plays a crucial role in the field of molecular biology and biotechnologe. By understanding various types of plasmids, researchers can select the appropriate vector for their specific applications. Each type of plasmid has unique characteristics that can enhance the efficiency of gene delivery, expression, and manipulation within various host systems.
When discussing diversity, it's important to consider several factors:
- Functionality: Different plasmid vectors serve distinct purposes, such as gene cloning, expression, or even gene silencing.
- Compatibility: Not all plasmids are suitable for every host organism. The selection of a plasmid must consider the host's characteristics to maximize the success of transformation.
- Ease of Use: Some plasmids provide greater ease of use for specific applications, making them more desirable for researchers.
Exploring the three major categories of plasmid vectors can provide insight into their applications across the scientific spectrum: bacterial plasmids, viral vectors, and yeast plasmids.
Bacterial Plasmids
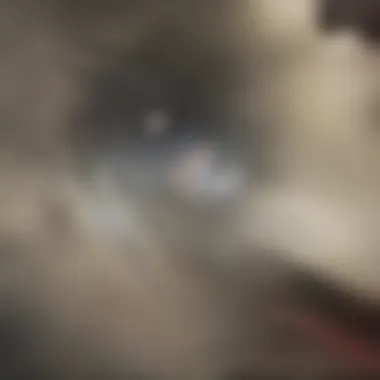
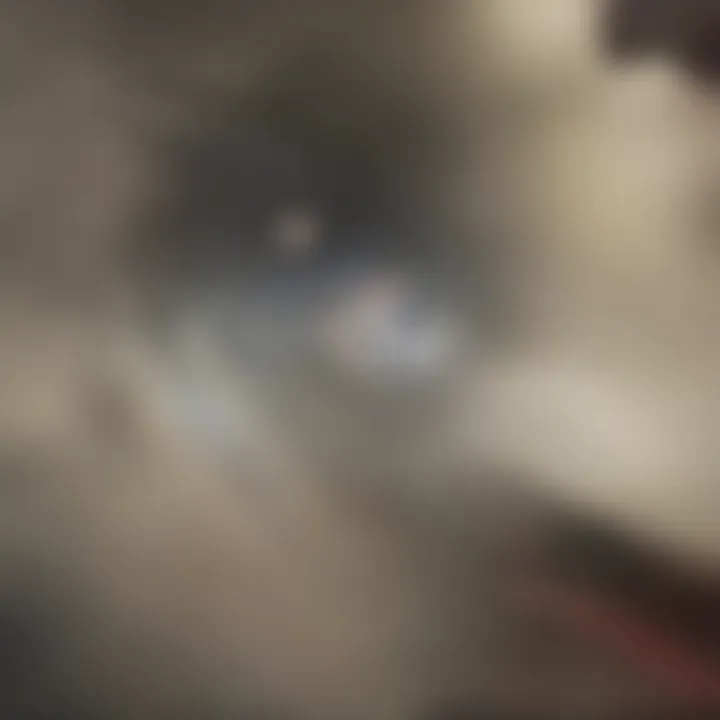
Bacterial plasmids are some of the most commonly used vectors in molecular biology. Their small size, high replicative ability, and intrinsic capabilities for transformation make them ideal for genetic manipulation. These plasmids often carry a replication origin, selectable markers, and multiple cloning sites, facilitating gene insertion and positive selection of transformed cells.
There are several notable benefits associated with bacterial plasmids:
- High transformation efficiency in bacterial cells, often reaching close to 100%.
- Rapid replication time, allowing quick analysis of genetic constructs.
- The availability of a vast array of well-characterized vectors, simplifying the experimental design.
However, researchers must also consider limitations with bacterial plasmids, such as their inability to express eukaryotic genes adequately due to post-translational modifications.
Viral Vectors
Viral vectors provide an intriguing alternative for gene delivery, particularly in eukaryotic systems. Their natural capability to infect cells facilitates the introduction of foreign genetic material. Commonly used viral vectors include lentiviral, adenoviral, and adeno-associated viral vectors. Each type possess its own advantages, which include:
- High efficiency in gene delivery to a variety of cell types.
- Potential for stable integration into the host genome, allowing for long-term expression of the inserted gene.
- The ability to introduce large inserts of genetic material, which is often beneficial for certain applications.
Despite their advantages, the use of viral vectors raises safety concerns regarding potential immunogenicity and the risk of insertional mutagenesis, which may lead to unintended effects in the host organism.
Yeast Plasmids
Yeast plasmids are particularly appealing when studying eukaryotic gene expression and metabolism. These vectors possess unique elements that leverage the yeast's cellular machinery for post-translational modifications. This characteristic is valuable for eukaryotic gene studies and protein production. Some specific features of yeast plasmids include:
- Autonomously Replicating Sequences (ARS): Allowing for replication and maintenance in yeast cells.
- Selectable markers, enabling efficient identification of transformants.
- The ability to handle large inserts compared to bacterial plasmids.
While yeast plasmids offer many benefits, researchers must be cautious about the growth conditions and transformation methods, as these can impact results significantly.
Understanding the diversity of plasmid vectors expands researcher's toolkits, enabling them to make informed decisions in the design of their experiments.
In summary, the diversity of plasmid vectors encompasses bacterial plasmids, viral vectors, and yeast plasmids, each with unique advantages and considerations. This understanding can enhance the efficacy of genetic engineering and molecular biology applications.
Constructing a Plasmid Vector Map
The construction of a plasmid vector map is a pivotal process in molecular biology and genetic engineering. It serves as a blueprint for understanding how different elements of the plasmid fit together. This process starts with selecting vital features, which will aid in the plasmid's desired functionality. The importance of constructing a plasmid vector map lies not only in theoretical knowledge but also in practical applications. By creating a detailed map, researchers can streamline their experiments and ensure that the plasmid will function as expected in various applications, such as gene cloning and expression studies.
Selection of Features
Selecting the correct features for a plasmid vector map is fundamental. The chosen elements determine the efficiency and utility of the plasmid. A well-constructed vector map often includes the following features:
- Origin of replication: Essential for plasmid propagation within host cells.
- Selectable markers: Assist in identifying successfully transformed cells.
- Multiple cloning sites: Enable the insertion of foreign DNA at specific locations.
- Promoter regions: Control the expression of the target gene.
Each element must be evaluated for its role and compatibility with the overall vector design. Researchers must consider factors like host compatibility, ease of use, and the expression levels of the target gene. This selection process can significantly impact the success rate of subsequent experiments.
Design Software Tools
The advancement of technology has led to the development of various software tools for designing plasmid vector maps. These tools significantly enhance the efficiency of the construction process. Popular options include SnapGene, Vector NTI, and ApE (A Plasmid Editor). Each of these programs offers unique capabilities, such as:
- Visual representation: Simplify the design of complex maps.
- Feature annotations: Automatically label essential plasmid components.
- Sequence alignment: Ensure that sequences are correctly integrated before synthesis.
Using these tools allows researchers to simulate cloning strategies and anticipate potential complications before actual experimentation. It ultimately increases the accuracy of the plasmid design and reduces the likelihood of errors in practical settings.
"Constructing a plasmid vector map with precision can enhance the success of genetic engineering projects, saving time and resources."
Applications of Plasmid Vector Maps
Plasmid vector maps play a significant role in molecular biology and genetic engineering. Understanding their applications is crucial for scholars and professionals in these fields. This section highlights the multifaceted applications of plasmid vector maps, their benefits, and various considerations.
Gene Cloning
Gene cloning is one of the most prominent applications of plasmid vector maps. In this process, specific DNA segments are inserted into plasmid vectors to create recombinant DNA. The primary goal is to produce copies of a particular gene or DNA sequence. The cloned gene can be analyzed, modified, or expressed.
The steps involved in gene cloning require precision. Plasmid vector maps provide a clear layout of essential features needed for successful cloning. This includes the origin of replication, which ensures that the plasmid is replicated within the host cell. Selectable markers are equally important as they allow researchers to identify successfully transformed cells. Through these processes, scientists can clone genes from various organisms, leading to advances in biotechnology and medicine.
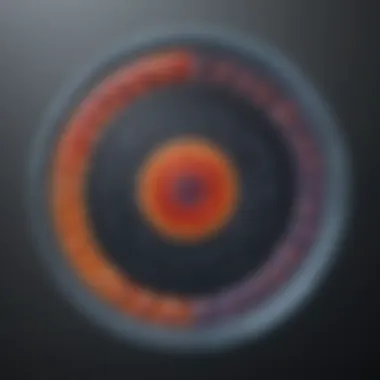
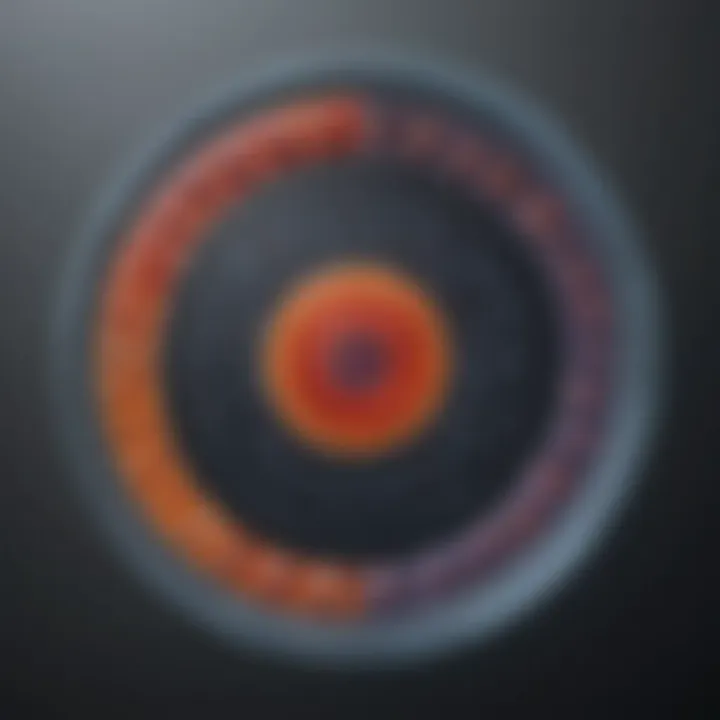
"Gene cloning using plasmid vectors has paved the way for significant breakthroughs in genetic research, including the production of insulin and other therapeutic proteins."
Gene Expression Studies
Gene expression studies are essential for understanding cellular functions and responses. Plasmid vector maps facilitate these studies by enabling researchers to analyze how genes are expressed in different conditions. With plasmids that contain proper promoter regions, it is possible to drive the expression of target genes in various host organisms.
The flexibility in choosing different promoters and regulatory elements allows scientists to fine-tune gene expression. During experiments, researchers can monitor proteins' production levels, allowing for the assessment of gene activity in real-time. This information can lead to breakthroughs in understanding diseases, discovering drug targets, or developing novel therapeutic strategies. Thus, plasmid vector maps serve as indispensable tools in the toolkit of molecular biologists.
Transgenic Organisms
Another vital application of plasmid vector maps is in the creation of transgenic organisms. These organisms incorporate foreign genes into their genomes, resulting in new traits or characteristics. Plasmids, when designed correctly, can facilitate the transfer of these foreign genes into host organisms, both plants and animals.
For example, in agriculture, transgenic plants have been developed to express resistance to pests or diseases. In animal research, transgenic mice are often used to study gene functions and model human diseases. The detailed understanding of plasmid vector maps allows researchers to select appropriate genetic constructs for effective incorporation into the target organisms. This application underscores the transformative impact of plasmid technology on modern agriculture and biomedical research.
Challenges in Plasmid Vector Use
The use of plasmid vectors in genetic manipulation presents several challenges that researchers must navigate. These challenges are crucial to understand, as they can significantly impact the efficiency and reliability of experiments involving plasmid technology. Addressing these issues is imperative for improving the outcomes of research and applications in biotechnology.
Stability Issues
Stability of plasmid vectors is a fundamental concern. Plasmids often undergo genetic recombination or degradation, which can lead to loss of the desired functional elements. Factors affecting stability include the host organism, environmental conditions, and the plasmid design itself.
- Host organism: The choice of bacterial strain can influence plasmid retention. Some strains may integrate plasmids into their own genomic DNA, reducing the number of functional plasmids.
- Environmental conditions: Fluctuations in temperature, pH, and nutrient availability can also affect stability.
- Plasmid construction: The incorporation of certain elements, such as repetitive sequences, can predispose plasmids to instability.
Researchers must implement strategies to enhance plasmid stability, such as designing plasmids with robust replication origins and using appropriate host strains that exhibit high plasmid retention rates.
Contaminant Risks
Contaminant risks pose another significant challenge in the use of plasmid vectors. Unintended microbial contaminants or foreign DNA can compromise experimental integrity and lead to erroneous results. Common sources of contamination include:
- Laboratory environment: Airborne particles, water supply, and even reagents can harbor contaminating organisms.
- Improper handling techniques: Cross-contamination during the manipulation of plasmids can introduce unwanted genetic material.
To mitigate these risks, strict laboratory protocols and regular monitoring of materials are essential. This includes maintaining clean environments, using sterilization techniques, and implementing proper waste disposal methods.
"Understanding the challenges in plasmid vector use is vital for advancing genetic engineering and ensuring the reliability of research outcomes."
Future Perspectives in Plasmid Technology
The landscape of plasmid technology is continually evolving, driven by advancements in genetic engineering and molecular biology. Future perspectives in this field reveal new opportunities and challenges, shaping how researchers approach genetic manipulation. Considering these trends is essential for anyone involved in biotechnology, genetic research, or education.
One pivotal element shaping the future is the increasing demand for precision in genetic modifications. With the advent of CRISPR-Cas9 technology, the potential to develop targeted plasmid vectors has gained momentum. These refined vectors can minimize off-target effects and enhance the efficiency of gene editing. As researchers improve the accuracy of these tools, many expect a significant reduction in the unintended consequences typically encountered in genetic modifications.
Furthermore, ongoing innovations lead to the development of novel plasmid designs. For instance, advancements in synthetic biology are encouraging the design of plasmids with intricate control elements. This may allow for more sophisticated regulation of gene expression. Researchers may be able to create plasmids that respond dynamically to environmental signals. Such capabilities would significantly improve the effectiveness of gene therapy applications and transgenic organisms.
From a practical standpoint, scalability presents both opportunities and challenges in plasmid technology's future. The growth of personalized medicine emphasizes the need for efficient, large-scale production of plasmids. New methods of plasmid synthesis and purification could make it more viable to produce these vectors on a commercial scale while maintaining quality. For instance, bioreactor technologies might play a critical role in increasing yield and reducing time and costs associated with plasmid production.
The integration of bioinformatics into plasmid design is a promising avenue that cannot be overlooked. Computational tools can aid researchers in predicting the behavior of plasmid vectors in various biological systems. With more refined predictive models, scientists can better tailor plasmids for their intended functions. This may result in a more targeted approach to plasmid education and application.
"The integration of advanced technologies will allow for unprecedented customization and efficacy in plasmid applications across various fields."
Another essential consideration lies in regulatory frameworks. As plasmids become central to novel therapies, particularly in human subjects, oversight becomes crucial. Striking a balance between innovation and safety will be paramount. As regulatory bodies adapt, their guidelines will significantly impact how plasmid technologies are applied in real-world scenarios. Companies and researchers must stay informed of these evolving guidelines to navigate the complexities of compliance effectively.
Lastly, the globalized nature of scientific research fosters collaboration across disciplines and borders. Enhanced cooperation between researchers, industry, and regulatory agencies will likely fuel advancements in plasmid technology. Shared knowledge and expertise can address challenges in plasmid vector development, ultimately benefiting society by translating scientific discoveries into practical applications.
In summary, the future of plasmid technology holds great promise. Emphasizing precision, novel designs, scalability, bioinformatics integration, regulatory considerations, and global collaboration will significantly shape the next generation of plasmid vectors. As these advancements unfold, they will not only enhance our understanding of molecular biology but also provide robust tools to tackle various challenges across healthcare and biotechnology.
The End
In this exploration of plasmid vector maps, we arrive at a crucial reflection on their significance within molecular biology and genetic engineering. The understanding of plasmid vector maps is foundational for students, researchers, educators, and professionals engaged in scientific investigation. These maps provide a visual blueprint of genetic elements, facilitating an enhanced comprehension of how genes can be manipulated for various applications.
One major benefit of mastering plasmid vector maps is their direct influence on the efficiency of gene cloning processes. A well-constructed vector can streamline the isolation and amplification of specific DNA sequences, leading to more effective experimental outcomes. Moreover, precision in designing vector maps reduces the chances of misreading genetic codes or creating unintended mutations. This precision is essential when developing transgenic organisms or conducting gene expression studies, as any oversight could result in adverse or unexpected results.
The value of these maps extends to their utility in various fields, from biopharmaceutical production to agricultural enhancement. The applications are diverse and growing, demonstrating the adaptability of plasmid vectors in addressing real-world challenges. As we consider future advancements in this technology, it becomes imperative to remain cognizant of both the promise and potential challenges presented by plasmid vector utilization.
As the complexities surrounding genetic engineering deepen, the need for clear and accurate plasmid vector maps becomes ever more critical. This article has aimed to clarify those complexities and underline their relevance in today’s research practices. Ultimately, a strong grasp of plasmid vector maps not only enhances scientific understanding but also paves the way for innovative solutions to genetic manipulation needs.
"Understanding the architecture of plasmids is key to advancing genetic engineering techniques."