Exploring the Mechanics of Plant Systems
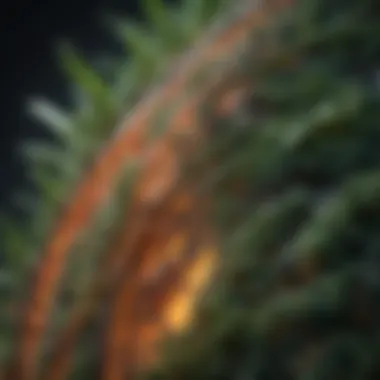
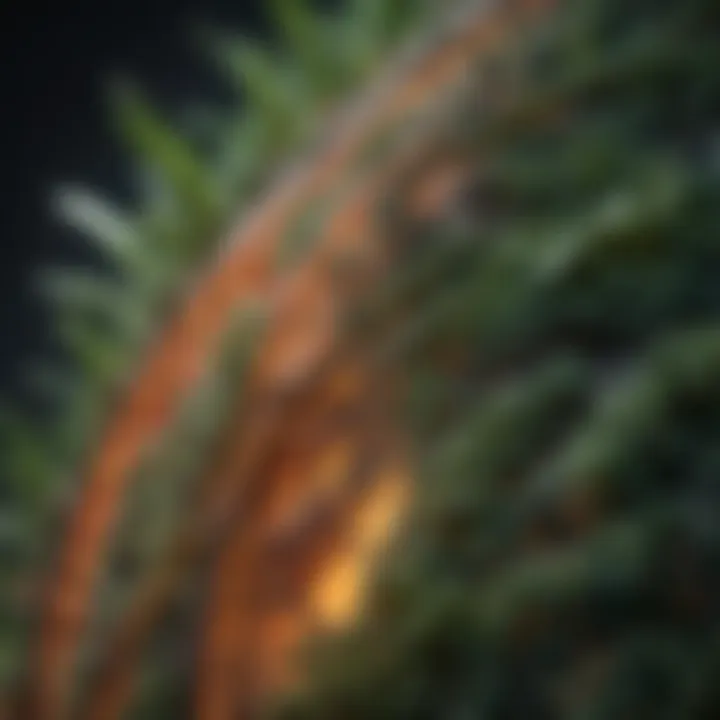
Intro
The study of plant mechanical systems is a multi-faceted field that combines aspects of biology, physics, and engineering. Plants, unlike animals, do not move to respond to their environments; instead, they rely on their internal mechanical systems for stability, support, and adaptability. The intricacies of these systems are essential for understanding plant growth and resilience. As plants encounter varying environmental stimuli, their mechanical systems must respond in a manner that facilitates optimal function and survival. This article aims to dissect these components thoroughly, from cellular structures to broader biomechanical principles.
Methodologies
To explore plant mechanical systems, a variety of methodologies are applied. This encompasses both experimental and theoretical approaches, enabling a comprehensive understanding of the subject.
Description of Research Techniques
Research techniques include:
- Microscopy: High-resolution imaging, particularly scanning electron microscopy, allows for detailed examination of plant cell structures. This is crucial for identifying the architecture of tissues that contribute to mechanical stability.
- Biomechanical Testing: Methods such as tensile and compression tests provide insights into the strength and flexibility of plant materials. This data is important for understanding how plants withstand external forces.
- Finite Element Analysis: This computational method simulates physical interactions and mechanical properties of plant structures. It helps predict how plants react under various stresses.
Tools and Technologies Used
The successful implementation of plant mechanical studies relies on specific tools:
- Scanning Electron Microscopes: These advanced instruments provide high-resolution images needed for cellular analysis.
- Load Cells: Used in biomechanical testing, these devices measure the force applied to plant structures.
- Software for Modeling: Programs like ANSYS and COMSOL Multiphysics are crucial for finite element analysis. They allow researchers to simulate various mechanical behaviors in plants under different conditions.
Discussion
Comparison with Previous Research
A review of existing literature reveals significant advancements in understanding plant mechanics. Earlier studies primarily focused on individual components, such as cell wall properties and material strength. Recent research integrates these findings into a more comprehensive framework. This shift has led to a better understanding of how mechanical systems contribute to overall plant resilience.
Theoretical Implications
The knowledge gained from examining plant mechanical systems has broad implications, including:
- Evolutionary Biology: Understanding how mechanical properties evolved can provide insights into plant evolution in response to environmental pressures.
- Agricultural Innovation: Insights into plant mechanics can inform breeding programs aimed at enhancing crop resilience to climate change.
"Thorough understandings of plant mechanics are critical to innovations in agriculture and ecology."
This narrative not only highlights the significance of studying mechanical systems in plants but also positions it as a foundational element for future research and applications. By synthesizing information from various disciplines, we can create a comprehensive understanding of how these systems function and their relevance to plant biology.
Preface to Plant Mechanics
In the study of plant biology, the understanding of plant mechanics is fundamental. This discipline focuses on the mechanical properties and systems that ensure plants can thrive in diverse environments. Every aspect from growth patterns to structural stability is shaped by mechanical considerations. Recognizing these factors can significantly deepen our appreciation of plant life.
Defining Plant Mechanics
Plant mechanics refers to the physical principles that govern the structure and behavior of plants. This includes the study of materials that make up plant tissues such as cellulose, lignin, and other polymers. These materials provide essential support and elasticity.
Moreover, plant mechanics examines how these structures respond to forces, such as wind or the weight of fruits and flowers. Through understanding plant mechanics, researchers can explain how plants maintain integrity and function under various stressors.
Importance of Studying Plant Mechanics
Studying plant mechanics is crucial for several reasons. First, it helps in understanding how plants adapt to their environments. When plants face challenges like drought or high winds, their mechanical properties enable them to withstand these phenomena. This knowledge is substantial for agricultural practices and ecosystem management.
Additionally, insights from plant mechanics can inspire innovations in biomimicry. Engineers and designers often look to nature for solutions to structural problems.
"The mechanical properties of plants offer a treasure trove of information that can be applied in engineering and material sciences."
In summary, the exploration of plant mechanics is not just about understanding plants. It paves the way for advancements in technology, sustainability, and environmental conservation.
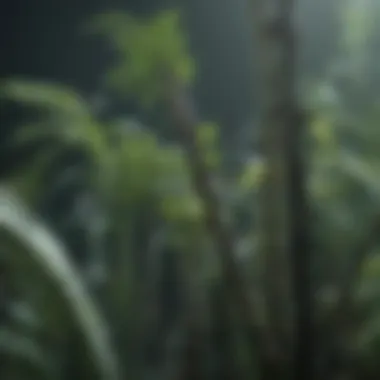
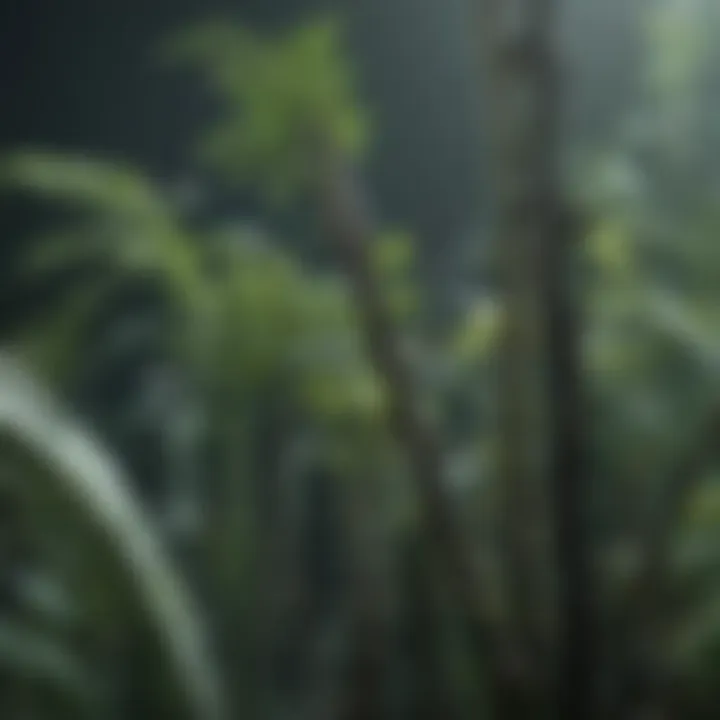
Cellular Structures in Plants
Cellular structures are foundational to understanding plant mechanics. Plant structures, ranging from cell walls to chloroplasts, play crucial roles in maintaining the integrity and function of plants. In this section, we will thoroughly examine these cellular elements and how they contribute to overall plant mechanics.
Cell Wall Composition and Function
The cell wall is a crucial component of plant cells. It provides structural support and protection. Cell walls are primarily composed of cellulose, hemicellulose, and lignin.
- Cellulose is a polysaccharide that forms the backbone of the cell wall, offering tensile strength.
- Hemicellulose contributes to the rigidity and helps to bind cellulose fibers together.
- Lignin adds compressive strength, especially in woody plants.
The composition of the cell wall varies among different plant species. This variability illustrates the adaptation of plants to diverse environments. For instance, plants in arid regions tend to have thicker cell walls to retain water. Also, the cell wall is involved in growth regulation and can respond to various environmental stimuli. This responsiveness is vital for the plantโs ability to adapt to its surroundings. To sum up, the composition and function of the cell wall are central to understanding plant resilience and mechanical stability.
Role of Chloroplasts in Mechanical Properties
Chloroplasts are well-known for their role in photosynthesis, but they also contribute to the mechanical properties of plants. Situated within plant cells, chloroplasts contain chlorophyll, which plays a critical role in capturing light energy.
- Turgor Pressure: Chloroplasts help maintain turgor pressure within cells. Turgor pressure is essential for keeping plant cells firm and maintaining overall structure. When chloroplasts perform photosynthesis, they produce sugars that contribute to osmotic balance, influencing the water retention of cells.
- Growth and Development: Chloroplasts influence growth patterns too. The energy they produce allows for cellular activities that support growth and mechanical strength. A healthy chloroplast population is crucial for a plant's structural integrity. Thus, while their primary function is energy production, their role in mechanical support cannot be ignored.
Chloroplasts are not just the powerhouses of the cell; they also play an important role in maintaining the physical structure of the plant.
Biomechanics of Plant Structures
Biomechanics of plant structures is a crucial aspect of understanding how plants function and thrive in diverse environments. This area of study intersects biology, physics, and engineering, offering insights into the intricate mechanical principles that govern plant growth and development. By examining these principles, we can appreciate how plants utilize mechanical advantages to optimize their form and function. This section will explore two fundamental concepts: the tensile strength of plant fibers and the compressibility and stability of roots.
Tensile Strength of Plant Fibers
Tensile strength refers to a material's ability to withstand tension without breaking. In plants, this characteristic is primarily rooted in their fibrous components, such as cellulose and lignin. These materials provide structural integrity and support, allowing plants to grow tall and withstand various physical stresses.
The significance of tensile strength lies in its role in determining how effectively a plant can resist bending, breaking, or deforming under load. For instance, the fibers in bamboo exemplify high tensile strength, enabling it to reach significant heights while remaining flexible. This is essential for survival in natural environments where wind or heavy rainfall may pose challenges.
Several factors affect the tensile strength of plant fibers:
- Cell Wall Composition: Different plants have varying ratios of cellulose, lignin, and hemicellulose, all contributing to the overall strength of the fiber.
- Orientation of Fibers: The arrangement of fibers influences their load-bearing capacity. For example, vertical fibers can better resist upward forces compared to horizontally oriented ones.
- Environmental Factors: Conditions such as humidity and temperature can impact the structural properties of plant fibers.
Understanding these mechanics can inform applications in materials science, such as developing sustainable building materials or optimizing agricultural practices to enhance crop resilience.
Compressibility and Stability in Roots
Roots play an essential role in plant biomechanics, primarily through their ability to provide stability and anchorage while absorbing water and nutrients. Compressibility is a vital factor in ensuring that roots can adapt to their surrounding soil conditions without compromising their structural integrity.
Root systems display remarkable adaptability. When subjected to varying pressure from soil or water, roots can compress and expand to maintain contact with the surrounding environment. This characteristic not only aids in stability but also allows roots to navigate through compacted soils or uneven surfaces.
Key factors affecting root compressibility and stability include:
- Soil Composition: The type of soil, its moisture content, and texture influence root behavior. Loosely packed soils allow for easier navigation compared to clay-heavy soils, which may inhibit growth.
- Root Architecture: The branching patterns and depth of roots can dictate how well a plant withstands forces acting upon it, such as those from wind or heavy rains.
- Hydration Status: Water content impacts root rigidity; hydrated roots are generally more sturdy than their dry counterparts.
A better grasp of root biomechanics informs agricultural practices and environmental management, enhancing crop yields while promoting sustainable land use.
"The intricate mechanics of plant structures reveal not only the beauty of nature but also the profound innovations that can be derived from them."
Mechanical Responses to Environmental Stimuli
Understanding mechanical responses to environmental stimuli is significant in plant biology. This section provides insights into how plants interact with their surroundings, adapting to various conditions through specialized growth responses. Plants face numerous environmental challenges, including light, gravity, touch, and moisture. Their ability to adapt is pivotal for survival and optimization of resources.
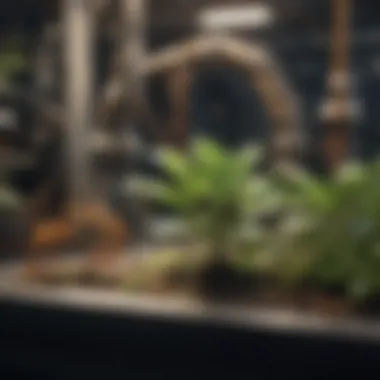
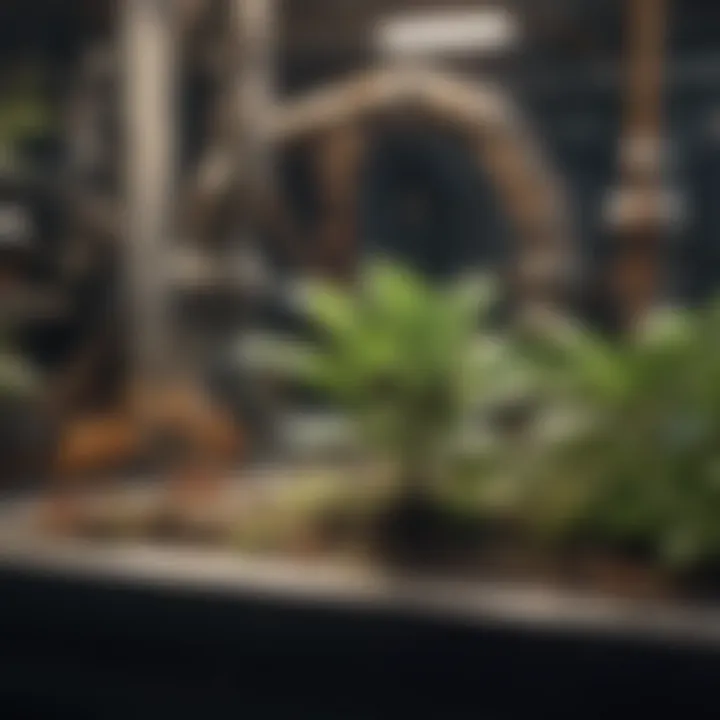
Tropisms: Growth Responses to Stimuli
Tropisms refer to directional growth responses based on stimuli. Plants exhibit positive or negative tropisms, where they grow towards or away from a stimulus, respectively. One common form of tropism is phototropism, where plants bend towards light sources. This adaptation maximizes photosynthesis, allowing plants to harness energy more efficiently.
In addition to phototropism, gravitropism affects plant orientation concerning gravity direction. Roots usually demonstrate positive gravitropism by growing downward, anchoring the plant while searching for water and nutrients. Alternatively, stems exhibit negative gravitropism, growing upward towards light. This dual response highlights how crucial environmental stimuli are for growth.
Mechanisms like auxins, a class of plant hormones, facilitate these tropic responses. They regulate cell elongation and promote directional growth. The concentration of auxins varies depending on the light and gravity, allowing the plant to adapt accordingly. As a direct consequence, plants can ensure their survival by optimizing light intake and stabilizing their position.
Nastic Movements: Non-directional Growth
In contrast to tropisms, nastic movements are non-directional responses to stimuli that do not depend on the direction of the stimulus. A classic example is the opening and closing of flowers or leaves in response to environmental conditions like light and temperature. These movements are crucial for protecting the plant from harsh conditions or maximizing reproductive success.
The mechanism behind nastic movements involves turgor pressure changes within the cells. When conditions are favorable, cells may increase their water content, leading to expansion. Conversely, dehydration results in cell contraction. Such adjustments not only affect a plant's appearance but also enhance its resilience. For instance, the touch-me-not plant (Mimosa pudica) swiftly folds its leaves when disturbed, a defense mechanism against herbivores.
To summarize, both tropisms and nastic movements illustrate the diverse ways plants respond to environmental stimuli. By understanding these mechanisms, researchers can gain insights into plant adaptability and resilience. The implications of these adaptations extend beyond botany, impacting agriculture and horticultural practices.
Mechanical Adaptations in Various Environments
Understanding mechanical adaptations in various environments is critical for comprehending how plants thrive under diverse conditions. These adaptations are not merely structural; they represent a concerted effort by plants to optimize resource use and ensure survival. By evolving specific traits, plants respond to their ecosystems, displaying resilience against external challenges such as water availability, mechanical stress, and light conditions. The study of these adaptations sheds light on the evolutionary processes that favor certain structural features, directly impacting plant success and biodiversity.
Adaptations in Aquatic Plants
Aquatic plants exhibit unique mechanical adaptations that allow them to thrive in water. Unlike terrestrial plants, which experience forces like gravity, aquatic plants predominantly deal with buoyancy. These adaptations include:
- Flexible Stems: Many aquatic species, such as water lilies, have soft and flexible stems. This flexibility allows them to sway with water currents, reducing the risk of breakage.
- Reduced Lignin: The structural component lignin, which provides strength to terrestrial plants, is less prominent in aquatic plants. This reduction optimizes buoyancy and leads to lighter structures, facilitating floatation.
- Wider Leaves: Leaves in aquatic plants tend to be broader and flatter. This design maximizes surface area for photosynthesis while allowing them to float effectively on the water's surface.
Moreover, adaptations like aerenchyma (air-filled cavities within plant tissues) enable efficient gas exchange even under submerged conditions. This adaptation is evident in species like Eichhornia crassipes, commonly known as water hyacinth, which maintains buoyancy and optimizes photosynthesis.
Desert Plants and Structural Resilience
Desert environments pose significant challenges due to extreme temperatures and limited water supply. Plants in these regions have evolved several structural adaptations to ensure survival, including:
- Thickened Cuticles: Desert plants like the saguaro cactus have thick cuticles that minimize water loss through evaporation. This feature is crucial for maintaining hydration.
- Modified Leaves: Many desert plants have modified leaves that take the form of spines or scales. This modification reduces the surface area, thus decreasing transpiration.
- Deep Root Systems: To access deeper water sources, desert plants develop extensive root systems. For instance, Creosote bush (Larrea tridentata) can spread roots widely to absorb moisture efficiently, even from distant sources.
These adaptations enhance structural resilience, enabling desert plants to withstand both mechanical stress and climatic extremes. Their unique strategies not only ensure survival but also contribute to the ecological balance in arid environments.
"Adaptations in plant structure are vital for survival in extreme environments, allowing plants to optimize resource use and maintain ecological balance."
In summary, mechanical adaptations are paramount for plants as they navigate the complexities of various habitats. From buoyant structures in aquatic species to drought-resistant features in desert plants, these adaptations showcase the remarkable resilience of plant life.
Plant Growth Dynamics
Understanding the mechanisms behind plant growth is essential for grasping how plants interact with their environment. Plant growth dynamics encompass the various processes through which plants increase in size and develop structures vital for their survival. These dynamics highlight the adaptability of plants in response to external forces, confirming their resilience.
- Importance of Plant Growth Dynamics
The study of plant growth dynamics is crucial for several reasons:
- It offers insights into how plants utilize resources efficiently.
- Knowledge of growth responses aids in agricultural advancements and crop improvement.
- Understanding these mechanisms can inform conservation strategies for threatened ecosystems.
- It also allows researchers to explore the implications of environmental changes on plant behavior.
"Plants exhibit remarkable flexibility in response to physical forces, revealing the sophistication of their growth dynamics."
Mechanisms of Growth in Response to Forces
Plants respond actively to mechanical forces, which affect their growth patterns. This response is often known as mechanotransduction. When plants are subjected to external stresses, such as wind or touch, specific signaling pathways are activated. This leads to adaptations that ensure their structural integrity and overall fitness.
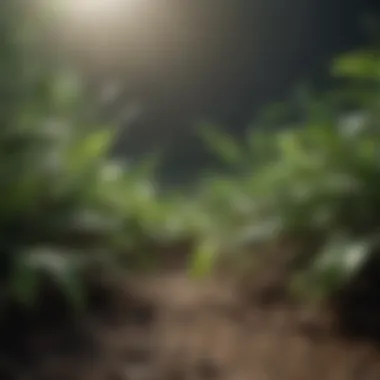
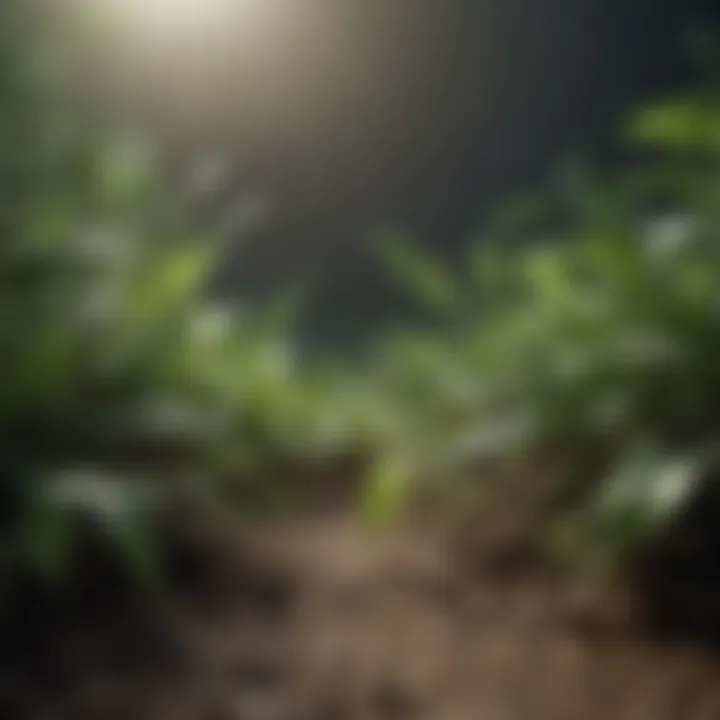
- Thigmotropism is a type of growth response where plants respond to physical contact. For example, climbing plants like Phaseolus vulgaris (common bean) tend to adapt their growth direction when they encounter obstacles, allowing them to optimize sunlight exposure.
- Gravitropism, another crucial response, allows roots to grow downward while shoots grow upward, ensuring proper anchorage and resource acquisition.
Key Factors Influencing Growth in Response to Forces
- Tension and Compression: Plants experience both tensile and compressive forces that impact their growth direction and structural formation.
- Wind Stress: Exposure to wind can lead to thicker stems as plants adapt to withstand these forces.
This dynamic adjustment in growth mechanisms emphasizes the intelligent design of plant structures, reinforcing their survival and adaptability.
Lateral Growth and Support Structures
Lateral growth is fundamental to plant architecture, contributing to stability and nutrient absorption. This growth is often referred to as secondary growth, which enhances the plant's girth and thereby its support.
- Cortex and Vascular Cambium:
The formation of the cortex and vascular cambium supports lateral growth. The vascular cambium produces new xylem and phloem cells, increasing the plantโs efficiency in transporting water and nutrients. - Root Structure: Furthermore, lateral roots extend outwards, improving stability and nutrient access in the soil. For instance, trees have extensive root systems that not only support their massive structure but also stabilize their position in the ground during adverse weather conditions.
In summary, lateral growth and the development of support structures are pivotal in ensuring plants maintain their upright position and effectively gather resources, demonstrating the intricate balance plants maintain in their quest for survival.
Technological Applications of Plant Mechanics
The realm of technological applications of plant mechanics indicates a marriage between nature and human ingenuity. Understanding how plants operate mechanically is crucial for advancements in various fields. By studying the methods that plants employ to grow, adapt, and withstand environmental stresses, we can derive innovative solutions to pressing engineering problems. This section will delve into specific elements that highlight the benefits of applying plant mechanics to technology, such as sustainability, efficiency, and inspiration for design.
Biomimicry in Engineering Solutions
Biomimicry is the practice of drawing inspiration from nature's designs and processes. In terms of plant mechanics, it allows engineers to solve complex problems by mimicking the strategies plants use for growth and stability. For instance, studying how trees distribute mechanical stress can influence the design of buildings, making them more resilient to lateral forces like wind.
Key aspects of biomimicry include:
- Sustainable Materials: Materials that mimic the lightweight yet strong structure of plant fibers, such as those found in bamboo, are continuously researched to improve the longevity and performance of building materials.
- Adaptive Structures: Just like plants adapt their growth based on environmental signals, structures designed using biomimicry can adapt to dynamic conditions, improving safety and longevity.
- Energy Efficiency: Plants have evolved mechanisms for energy efficiency, such as using sunlight more effectively. Engineers can develop systems that optimize energy consumption by mimicking these natural processes.
Overall, biomimicry presents numerous opportunities to enhance human-made systems through lessons learned from plant mechanics, leading to holistic and sustainable engineering solutions.
Robotics Inspired by Plant Movement
Robotics inspired by plant movement is another area where the mechanical systems of plants have profound implications. Plants exhibit unique movement patterns, like tendrils wrapping around supports or leaves turning toward light, demonstrating a responsive capability that can be replicated in robotics. Understanding these movements can lead to the development of soft robotics and bio-inspired mechanisms that are versatile and efficient.
Relevant features of robotics designed around plant movement include:
- Soft Robotic Applications: These robots, inspired by the flexible and adaptive movements of plants, can navigate complex environments, performing tasks that traditional rigid robots struggle to accomplish.
- Environmental Interaction: Similar to how plants respond to external stimuli, robots can be designed with sensory feedback mechanisms, allowing them to adjust their movements based on changes in their surroundings.
- Natural Energy Utilization: Robotics inspired by the efficiency of plants in energy usage can improve battery life and reduce energy costs, leading to more sustainable technology.
"The exploration of plant mechanics not only enriches our understanding of biology but also paves the way for cutting-edge technological advancements that can reshape our future."
In summary, the technological applications of plant mechanics provide pathways to sustainable and innovative engineering solutions. The mechanisms in plants serve as blueprints that inform design principles across various disciplines. As researchers and engineers continue to explore these applications, the confluence of nature and technology promises to yield transformative results.
Epilogue
The analysis presented in this article illustrates the complexity of plant mechanical systems and emphasizes their integral role in the survival, growth, and adaptation of plant species. Understanding these systems provides valuable insights into how plants interact with their environments. The interplay of structural components, biochemical processes, and mechanical properties holds significant relevance not only for plant biology but also for fields like agriculture, bioengineering, and sustainable design.
Integrating Knowledge of Plant Mechanics
Integrating knowledge of plant mechanics means applying a multidisciplinary approach. This includes insights from biology, physics, and engineering. Such integration can enhance our understanding of how plants function mechanically. For instance, understanding the tensile strength of plant fibers can improve biomaterials in engineering. Furthermore, applies principles of biomechanics help in developing better agricultural practices and improving crop resilience.
In educational contexts, integrating knowledge strengthens curriculum designs in both high schools and universities. Students gain a multifaceted understanding of how plants work. This can spark interest in research and innovation, contributing to sustainable practices in various sectors.
Future Directions in Plant Mechanical Research
Future research in plant mechanics should focus on several key areas. One significant direction is the investigation of how climate change impacts plant mechanical systems. The shifts in environmental conditions affect growth patterns and structural stability in plants. Thus, understanding these links could advance conservation efforts.
Another promising area is the exploration of synthetic biology. Genetic engineering can enable researchers to manipulate plant structures for desired mechanical properties. This opens possibilities for creating new plant varieties that are more resilient to stressors.
In addition, researchers should harness advancements in technology, such as imaging and modeling techniques. These technologies can provide deeper insights into plant mechanics at both cellular and organism levels. Learning how plants function can also spark innovation in robotics and materials science.
Overall, understanding plant mechanical systems is essential for tackling many contemporary challenges. The insights gained will contribute to a sustainable future, advancing both knowledge and practical applications.