Exploring the Multifaceted Benefits of Hydrogen Energy
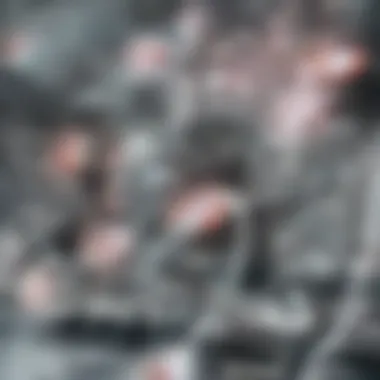
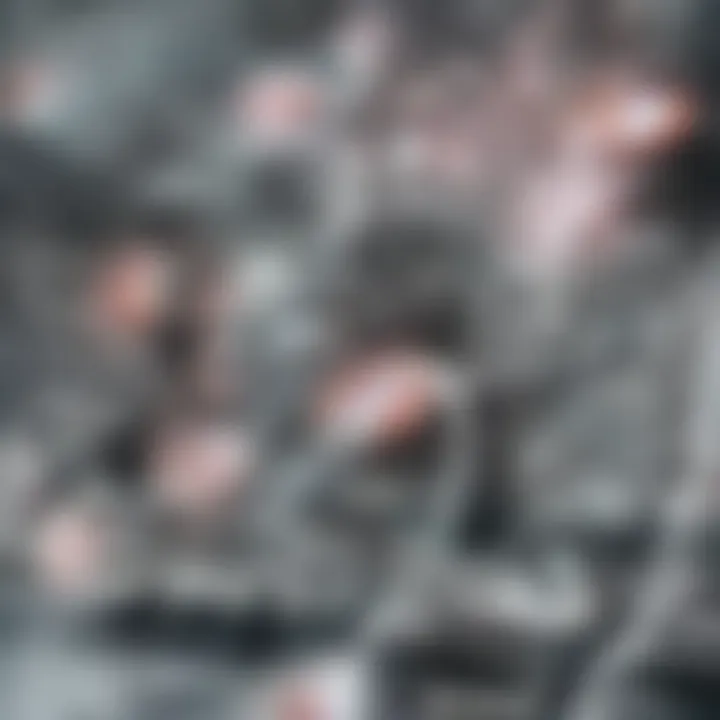
Intro
Hydrogen has emerged as a pivotal player in the global quest for sustainable energy solutions. Its potential as an energy carrier and as an essential raw material stretches across various sectors, including transportation, industry, and environmental sciences. People are realizing that in facing current energy challenges, hydrogen can serve as a beneficial alternative to conventional fossil fuels. This article aims to unpack the diverse advantages offered by hydrogen, establishing an understanding of its role in future energy systems.
Understanding hydrogen's multifaceted benefits involves diving into both theoretical and practical arenas. By examining its applications through various frameworks, and integrating insights from recent research, this discussion seeks to clarify hydrogen's economic implications and environmental advantages.
Methodologies
Description of Research Techniques
The assessment of hydrogen's benefits is not merely theoretical; it calls for a robust analysis of both qualitative and quantitative data. Various methodologies have been employed to understand its multifaceted nature:
- Literature Review: A comprehensive analysis of existing studies and papers illuminates the groundwork laid by scholars and researchers in the field of hydrogen energy.
- Case Studies: Real-world examples illustrate how hydrogen technologies have been implemented successfully across different sectors.
- Interviews and Expert Opinions: Insights from industry professionals and researchers contribute depth to the understanding of hydrogen's current and future applications.
This combination of methods aims to create a balanced perspective on hydrogen’s role in energy transitions.
Tools and Technologies Used
To further analyze hydrogen's benefits, various technological tools facilitate research:
- Simulation Software: Tools like Aspen Plus for process simulation help predict outcomes for hydrogen production and consumption.
- Data Analytics Platforms: Statistical software such as R or Python libraries can analyze data trends related to hydrogen markets.
- Environmental Assessment Tools: Lifecycle assessment tools evaluate environmental impacts associated with hydrogen production and utilization.
Using these tools, a structured approach is taken to obtain relevant data about hydrogen technology.
Discussion
Comparison with Previous Research
Previous studies have explored hydrogen predominantly through the lens of its production methods, like electrolysis and steam methane reforming. However, recent research expands on this by emphasizing hydrogen's application as a versatile energy vector in various sectors. As newer technologies emerge, researchers are starting to acknowledge how hydrogen can bridge gaps in renewable energy logistics, enhancing energy security.
Theoretical Implications
The theoretical framework surrounding hydrogen poses an interesting narrative. Analysts are increasingly linking hydrogen's potential to key theories of energy transitions and sustainability. Hydrogen could play a significant role in decarbonization strategies. Scholars argue its integration into existing energy systems could catalyze a broader shift towards global energy sustainability.
"The transition to a hydrogen economy may represent a fundamental restructuring of energy systems as we know them."
This article aims to synthesize these threads of research and discussion, reaffirming the importance of hydrogen in a sustainable energy landscape.
Foreword to Hydrogen
Hydrogen stands as one of the most promising elements in the quest for sustainable energy solutions. Understanding its significance in the modern energy landscape provides a foundational basis for the ensuing discussion on its numerous advantages and applications. The exploration of hydrogen is essential, not just for the immediate benefits it offers, but also for its potential to shape future energy systems.
Definition and Properties
Hydrogen is the simplest and most abundant element in the universe, consisting of just one proton and one electron. It is a colorless, odorless gas at room temperature. This diatomic molecule, H₂, exhibits unique properties that make it advantageous as an energy carrier. Notably, it possesses a high energy content per mass. When stored and used correctly, hydrogen can provide a clean alternative source of energy. Its combustion produces water vapor as the only byproduct, making it environmentally friendly. As nations strive to reduce carbon emissions, hydrogen's role becomes increasingly important.
Moreover, hydrogen can exist in various forms: gaseous, liquid, or solid. This versatility allows for a range of storage and transportation methods, enhancing its feasibility as a sustainable energy source.
Historical Context
The journey of hydrogen is rich with scientific discovery and innovation. Although hydrogen was first recognized as an element in the 18th century, its potential applications were not fully understood until more recent decades. Early experiments highlighted its explosive properties, which garnered interest and concern alike.
By the mid-20th century, hydrogen began to find its footing in various sectors, notably in chemical manufacturing and space exploration. The Apollo missions showcased hydrogen’s potential as a rocket fuel. In the latter part of the 20th century and into the 21st, the growing awareness of climate change ignited renewed interest in hydrogen as a clean energy alternative. Governments and researchers now view hydrogen not merely as a chemical compound but as a cornerstone of future energy solutions.
Hydrogen as an Energy Carrier
Hydrogen, as an energy carrier, is pivotal in discussions surrounding future energy systems. It offers distinct advantages over conventional fossil fuels and even some renewable sources. Hydrogen's ability to store and transport energy efficiently can significantly contribute to energy resilience. This characteristic is what makes hydrogen an appealing option for integrating renewable energy sources into the grid, such as wind and solar. When energy production exceeds demand, excess energy can be converted to hydrogen for later use. This flexibility is essential as it aligns with the growing need for sustainable energy solutions.
Mechanisms of Energy Storage
Hydrogen storage technology encompasses various methods that ensure energy is not lost and can be utilized when required. The primary approaches include:
- Compressed Hydrogen: This method involves compressing hydrogen gas in high-pressure tanks. It is a straightforward approach but requires robust containment structures to handle high pressures safely.
- Liquid Hydrogen: Hydrogen can be liquefied by cooling it to extremely low temperatures. This method allows for denser storage but demands significant energy to maintain low temperatures.
- Metal Hydrides: These are compounds formed with metals that absorb hydrogen. The metal can release hydrogen when exposed to specific conditions, making it a compact storage option.
- Chemical Hydrogen Storage: In this case, hydrogen is stored in chemical bonds and released through chemical reactions. This category may include organic compounds or other chemical systems.
Each of these systems has its pros and cons regarding capacity, safety, and energy efficiency.
Transportation Applications
Hydrogen's utility in transportation is one of its most compelling aspects. As the world pushes towards reducing carbon emissions, hydrogen presents a viable alternative.
Hydrogen Fuel Cell Vehicles
Hydrogen fuel cell vehicles (FCVs) operate using a technology that converts chemical energy directly into electricity, emitting only water as a byproduct. This feature is crucial as it aligns with global climate goals. "Zero emissions" is a powerful appeal for consumers and policymakers alike. FCVs can achieve longer ranges and shorter refueling times compared to traditional battery electric vehicles, making them practical for various applications.
Moreover, hydrogen fuel cells are less sensitive to cold weather than batteries, which can falter in low temperatures. However, the infrastructure for fueling remains a challenge as only a few regions offer widespread refueling stations.
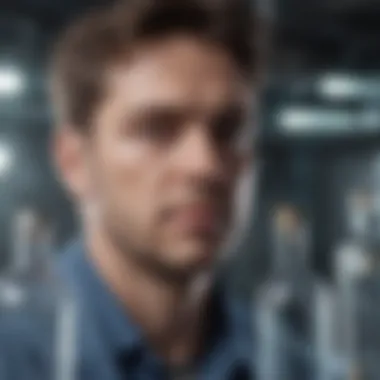
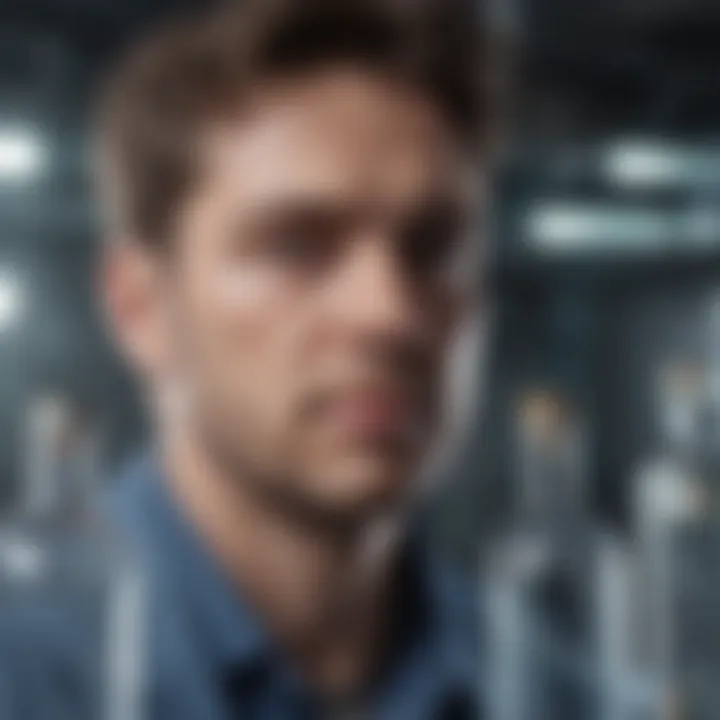
Comparative Analysis with Battery Electric Vehicles
Comparing hydrogen FCVs with battery electric vehicles (BEVs) reveals insightful contrasts. While BEVs rely on storing energy in batteries, FCVs generate electricity on-demand. This aspect can lead to quicker refueling times for FCVs, as they can be refueled in about five minutes, similar to conventional gasoline vehicles.
However, BEVs have a more established network of charging stations. This infrastructure development has been a significant contributor to the surge in BEV popularity.
Furthermore, the production of batteries involves sourcing materials that can raise ethical concerns, whereas hydrogen can be produced through various eco-friendly methods, such as water electrolysis using renewable energy.
In summary, while both hydrogen fuel cell vehicles and battery electric vehicles offer pathways to minimize carbon footprints, the choice between them depends on various factors including range, refueling convenience, and availability of infrastructure. The discussion around these options informs future transportation policies as we move towards sustainable energy solutions.
As hydrogen adoption continues to increase, understanding these applications and their implications will be crucial for stakeholders across various sectors.
Economic Advantages of Hydrogen
Hydrogen holds significant economic advantages that can change the landscape of energy production and consumption. As a versatile resource, it presents pathways for cost savings, job creation, and new industrial opportunities. The importance of this topic lies in understanding how hydrogen can be utilized effectively in various sectors, ultimately leading to a more sustainable and efficient energy system.
Cost-Effectiveness in Production
Steam Methane Reforming
Steam Methane Reforming (SMR) is a prevalent method for hydrogen production. This process involves reacting methane with steam at high temperatures to produce hydrogen and carbon dioxide. One key characteristic of SMR is its ability to leverage existing natural gas infrastructure, making it a practical choice for hydrogen production.
SMR contributes to the overall economic viability by providing a relatively low-cost source of hydrogen. Its established technology reduces initial investment costs, which is crucial for industries looking to transition to hydrogen. The unique feature of SMR is its efficiency, with current systems reaching up to 70% conversion rates. However, the process entails significant carbon emissions, which can lead to regulatory pushback.
Advantages:
- Utilizes existing infrastructure
- Lower initial investment
- High efficiency
Disadvantages:
- Significant greenhouse gas emissions
- Dependence on fossil fuels
Electrolysis of Water
Electrolysis of Water stands as a clean method for hydrogen production, using electricity to split water into hydrogen and oxygen. This process offers a significant economic advantage by enabling the production of green hydrogen when renewable energy sources are used. A major characteristic is its scalability. Electrolysis can be implemented on a small or large scale, depending on energy needs.
The unique benefit of this technology is its potential for sustainable hydrogen production. When coupled with solar or wind energy, electrolysis is an effective solution to reducing dependence on fossil fuels. However, it is important to note that the current cost of electricity and efficiency of the electrolytic cells can limit the economic viability in some circumstances.
Advantages:
- Can produce green hydrogen
- Scalable to meet energy demands
Disadvantages:
- High operational costs
- Energy efficiency still developing
Job Creation in Hydrogen Economy
As the hydrogen economy continues to evolve, there is a compelling argument for job creation within this sector. A recent analysis highlights that transitioning to hydrogen will generate numerous job opportunities across various fields. Manufacturing, research and development, and installation of hydrogen infrastructures promise to be significant employment sources.
A shift towards hydrogen could lead to not only new jobs but also the upskilling of current workers in traditional energy industries. Education and training programs focused on hydrogen technologies will be critical to equip workers for these new roles. This creates a dual benefit: fostering economic growth while allowing for a sustainable energy transition.
Moreover, a proactive hydrogen policy can attract investments, further stimulating growth in related sectors. As industries seek to innovate, the demand for hydrogen technologies will likely grow, creating a ripple effect that enhances employment across multiple sectors.
Environmental Benefits
The environmental benefits of hydrogen are significant and multifaceted. As the world grapples with climate change and deteriorating air quality, hydrogen presents itself as a viable solution for reducing pollution and moving toward sustainability. In this section, we will detail two major benefits: the reduction of greenhouse gas emissions and the improvement of air quality.
Reduction of Greenhouse Gas Emissions
One of the primary arguments for adopting hydrogen as an energy carrier is its potential to minimize greenhouse gas emissions. Traditional energy sources, such as coal and natural gas, release large amounts of carbon dioxide when burned. When hydrogen is used as a fuel, especially when it is produced from renewable resources, the combustion emits only water vapor as a byproduct. This contrasts sharply with fossil fuels, which contribute significantly to global warming.
Key Considerations:
- Production Methods: The environmental impact of hydrogen depends heavily on its production methods. Green hydrogen, produced through the electrolysis of water using renewable energy, offers the cleanest option.
- Transition to Hydrogen Economy: Shifting from fossil fuels to hydrogen could drastically reduce the carbon footprint of industries like transportation and manufacturing. The challenge remains in scaling up production and ensuring that infrastructure supports widespread adoption.
This potential for drastic emissions reduction could play a critical role in meeting global climate targets and transitioning to a low-carbon economy.
Air Quality Improvement
The implications of hydrogen extend beyond just carbon emissions; they also encompass air quality. Many urban areas suffer from air pollution, primarily due to vehicle emissions and industrial activities. Hydrogen fuel cell vehicles, for instance, emit no harmful pollutants such as nitrogen oxides and particulate matter, which are major contributors to smog and respiratory health issues.
Benefits of Hydrogen for Air Quality:
- Reduced Pollutants: Utilizing hydrogen does not just lower greenhouse gas emissions; it also significantly cuts down on hazardous air pollutants.
- Health Implications: Improved air quality translates to better public health outcomes. By reducing the presence of pollutants, cities can decrease healthcare costs and improve the quality of life for their citizens.
"Switching to hydrogen fuel isn’t just about energy; it’s about ensuring a healthier environment for future generations."
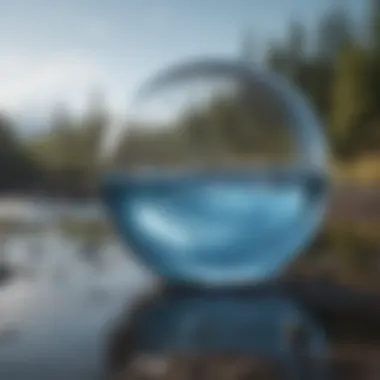
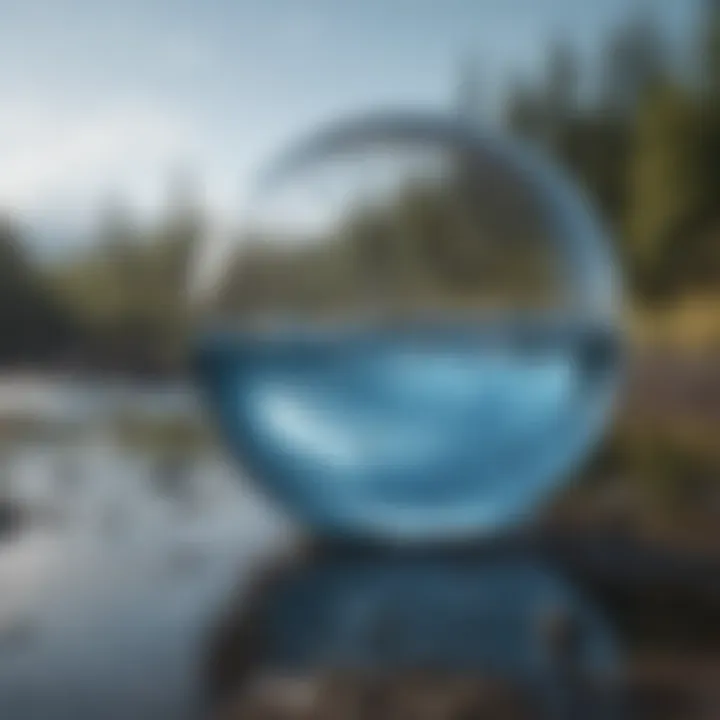
Through careful implementation and policy support, hydrogen can serve as an essential tool in the quest for cleaner air and a sustainable environment. The environmental benefits provided by hydrogen are significant, making it a crucial element in the global energy transition.
Industrial Applications of Hydrogen
Hydrogen plays a significant role in various industrial sectors. Its versatile applications not only fuel current manufacturing processes but also foster innovation in sustainable practices. Understanding hydrogen's impact on industrial applications is essential in recognizing its broader implications on global energy systems. Industries that utilize hydrogen can significantly benefit from improved efficiencies and reduced environmental footprints.
Hydrogen in Chemical Manufacturing
Ammonia Production
Ammonia production is one of the most prominent uses of hydrogen in chemical manufacturing. The Haber-Bosch process, which synthesizes ammonia from nitrogen and hydrogen, is vital for producing fertilizers. This process has allowed for mass food production, supporting the growing global population. The key characteristic of ammonia production is its reliance on hydrogen as a feedstock.
Additionally, ammonia serves as a hydrogen carrier, enabling easier storage and transport of hydrogen for various applications. The demand for ammonia is steadily increasing due to its centrality in agricultural practices and its potential in energy storage. However, conventional methods of ammonia production can be energy-intensive and contribute to greenhouse gas emissions.
Advantages of ammonia production:
- Facilitates large-scale food production
- Supports energy transition through hydrogen storage
Disadvantages:
- High energy consumption for traditional methods
- Associated carbon emissions if fossil fuels are used
Petrochemical Processes
In the realm of petrochemical processes, hydrogen plays an indispensable role. It is crucial in the refining of crude oil, where it helps in breaking down larger hydrocarbons into valuable products such as gasoline and diesel. Hydrogenation reactions, facilitated by hydrogen, are essential in converting unsaturated hydrocarbons to saturated, thus enhancing the quality of fuels.
The key characteristic of petrochemical processes is the efficiency that hydrogen brings to these refining operations. When implemented correctly, hydrogen can not only improve product yields but also reduce the need for other, more environmentally harmful substances.
Unique features of petrochemical processes include:
- Integration with existing refining systems
- Potential for circular economy through sustainable hydrogen sourcing
Advantages:
- Enhances fuel quality and efficiency
- Reduces emissions when sourced sustainably
Disadvantages:
- Hydrogen production can be carbon-intensive if using natural gas
Usage in Metals Industry
The metals industry significantly benefits from hydrogen as well. Hydrogen is increasingly used in processes such as direct reduction of iron ore. This method is more environmentally friendly than traditional methods that rely on coal. Using hydrogen not only reduces carbon dioxide emissions but produces water vapor as a byproduct instead. The transition to hydrogen in metallurgy can lead to a cleaner production process.
Moreover, hydrogen's role goes beyond steel; it is also being explored in other metallurgical applications. With innovations emerging in how hydrogen can be generated and utilized, the future of metals processing seems set to evolve further toward sustainability and efficiency.
Hydrogen Production Technologies
Hydrogen production technologies play a crucial role in maximizing the utility of hydrogen as an energy source. The development of effective and efficient methods is essential to meet the growing energy demands and transition towards sustainable energy systems. This section explores various current methods and innovative technologies pertinent to hydrogen production, assessing their viability and impact on both the economy and the environment.
Current Methods and Techniques
Several established methods exist for hydrogen production, each with their benefits and drawbacks. The most common techniques include steam methane reforming and electrolysis of water.
- Steam Methane Reforming (SMR): This technique involves extracting hydrogen from natural gas but does emit carbon dioxide in the process. It is currently the predominant method for industrial hydrogen production.
- Electrolysis of Water: Electrolytic hydrogen production splits water into hydrogen and oxygen using electricity. This method can provide green hydrogen, especially when renewable energy sources power the electrolysis.
Both methods have a significant role in the energy landscape, but optimal hydrogen production relies on integrating these techniques with renewable resources to minimize environmental impacts.
Innovative Production Technologies
Innovative production technologies are emerging to enhance hydrogen production efficiency and sustainability. Below, we discuss two noteworthy methods: Biomass Gasification and Photoelectrochemical Water Splitting.
Biomass Gasification
Biomass gasification is a process where organic materials are converted into hydrogen by heating them in low-oxygen environments. This technology contributes to waste reduction by using agricultural and industrial residues.
One key characteristic of biomass gasification is its ability to process various types of feedstock, making it a flexible choice for hydrogen production. The unique aspect of this process lies in its dual benefit of creating hydrogen while managing waste simultaneously. Despite these advantages, it may face challenges regarding feedstock availability and competition with food production.
Photoelectrochemical Water Splitting
Photoelectrochemical water splitting leverages sunlight to drive hydrogen production through the catalytic conversion of water. This method is notable for its potential to produce renewable hydrogen directly from solar energy, making it a promising solution for sustainable hydrogen generation.
The central feature of this technology is its use of semiconductor materials that facilitate the absorption of sunlight. The advantage here is the high potential for efficiency in hydrogen production from a renewable source. However, challenges include the need for more research on the stability and cost-effectiveness of materials used in the process.
In summary, while current methods of hydrogen production, such as SMR and electrolysis, are vital, innovative technologies like biomass gasification and photoelectrochemical water splitting hold promise for a more sustainable future.
Challenges Facing Hydrogen Adoption
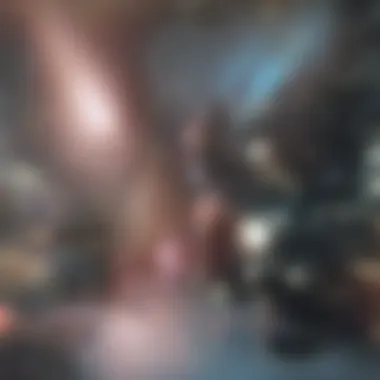
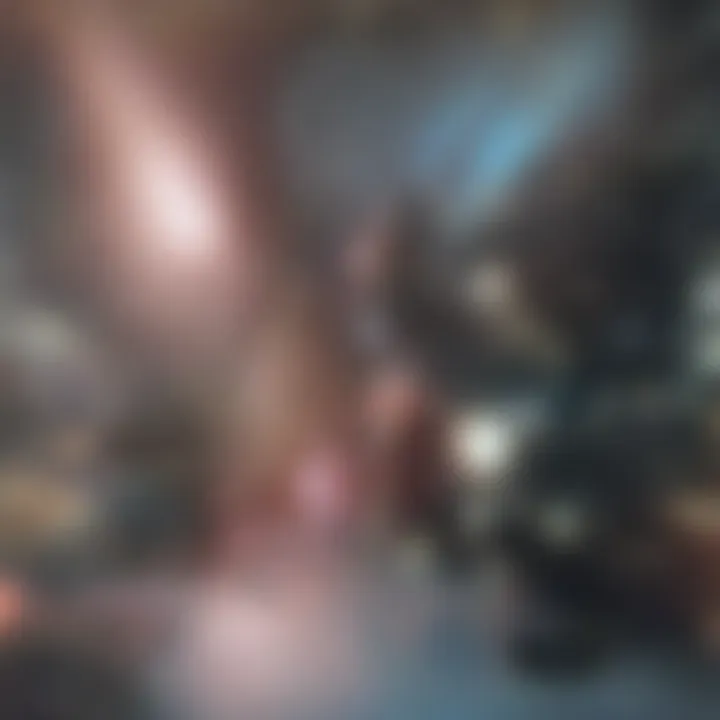
The journey towards integrating hydrogen into the global energy system is not without its hurdles. As potential benefits become more recognized, it is crucial to address the challenges that stand in opposition to its wider adoption. Addressing the infrastructure limitations and safety concerns is key not only for organizations thinking to invest in hydrogen solutions but also for regulatory frameworks that need to adapt for accommodating this technology.
Infrastructure Limitations
The infrastructure for hydrogen production, storage, and distribution is still developing. Current facilities are largely designed for fossil fuels and do not easily accommodate hydrogen’s unique properties. Hydrogen has a low energy density by volume, which means storing it requires pressure tanks or cryogenic conditions. Transporting hydrogen also presents its own challenges, as the existing pipeline network is primarily tailored for natural gas.
Investment in hydrogen infrastructure is essential. This includes developing facilities for electrolysis, enhancing pipeline networks, and establishing refueling stations that can support hydrogen vehicles. Moreover, retrofitting existing gas pipelines for hydrogen use presents technical and regulatory complications that need thorough examination. As of now, limited access to hydrogen refueling stations hampers the growth of hydrogen vehicle markets.
Safety Concerns
While hydrogen is a prevalent element with many promising applications, it does pose safety challenges. Hydrogen is highly flammable and can ignite easily, which necessitates rigorous safety protocols for its production, handling, and transportation.
Training for personnel who work with hydrogen technologies is equally important. Safety measures must be implemented at production sites, transport facilities, and end-user locations. Industry standards need to evolve to encompass specific safety guidelines for hydrogen, encompassing emergency preparedness and response.
"Hydrogen, though abundant, must be handled with care to minimize risks and maximize its potential."
Public perception also plays a significant role. Misinformation regarding hydrogen safety, rooted in historical accidents involving hydrogen leaks and explosions, can lead to skepticism. Addressing these misconceptions through education and transparent information dissemination is crucial in fostering acceptance of hydrogen technologies.
Policy and Regulatory Framework
The policy and regulatory framework surrounding hydrogen plays a crucial role in the advancement and adoption of hydrogen technologies. Governments must create conducive environments that support innovation, investment, and research. Various policies, ranging from subsidies to stricter emissions regulations, affect how hydrogen is perceived in the energy landscape. A strong policy framework will encourage stakeholders to invest time and resources into hydrogen, ensuring its successful integration into existing systems.
The intersection of policies can significantly influence the overall effectiveness of hydrogen as an energy solution. By setting clear guidelines and goals, policymakers can stimulate both public and private sectors to collaborate seamlessly on hydrogen initiatives. This collaboration is vital for overcoming barriers encountered in infrastructure and safety, which are essential for widespread hydrogen use.
Incorporating hydrogen into national energy strategies not only enhances energy security but also positions countries as frontrunners in the global energy transition. These frameworks should also consider the socio-economic factors that can further promote hydrogen in different sectors.
"A robust regulatory environment establishes the foundation upon which the hydrogen economy can flourish."
Government Incentives for Hydrogen
Government incentives are pivotal in shaping the hydrogen landscape. Various types of financial support are available for hydrogen projects ranging from grants and subsidies to tax breaks. These incentives can directly lower the overall costs associated with research, production, and deployment of hydrogen technologies.
Several key elements of government incentives include:
- Research and Development Grants: Funding for innovation can help improve hydrogen technologies, making them more efficient and cost-effective.
- Tax Credits: Offering tax credits for companies investing in hydrogen infrastructure can stimulate private sector investment.
- Direct Subsidies: These can help offset the initial costs of hydrogen fuel cells and production facilities.
- Pilot Programs: Governments can fund pilot projects to demonstrate the viability of hydrogen applications in real-world scenarios.
International Treaties and Agreements
International collaboration on hydrogen is essential for the development of integrated solutions across borders. Many countries recognize the significance of global climate agreements and have begun to incorporate hydrogen into their commitments. Treaties and agreements can outline cooperation on hydrogen research, trade policies, and technical standards.
Some notable aspects of international treaties regarding hydrogen include:
- Shared Research Initiatives: Countries can collaborate on large-scale research projects to share knowledge and technology.
- Standardization of Hydrogen Technologies: Agreements can help create uniform standards for hydrogen production and use, facilitating easier trade and cooperation.
- Investment Frameworks: These can encourage foreign investment in hydrogen technologies and infrastructure across nations.
By fostering international relationships focused on hydrogen, countries can leverage shared knowledge and resources, accelerating progress towards a sustainable energy future.
Future Perspectives of Hydrogen
The future perspectives of hydrogen are crucial in understanding how this versatile element can significantly shape our energy systems and industrial processes. As nations strive to reduce carbon footprints and meet climate goals outlined in international agreements, hydrogen emerges as a strategic asset. It offers not only a potential means for decarbonizing existing sectors but also a pathway for innovation in energy storage and transport solutions.
Emerging Technologies
Technological advancement plays a vital role in hydrogen's future. The current landscape showcases several innovative methods for hydrogen production and utilization. Notably, electrolysis has gained traction; it involves splitting water into hydrogen and oxygen using renewable electricity. This process results in green hydrogen, a clean solution that can be produced wherever renewable resources are abundant.
Other promising technologies include biomass gasification, which converts organic materials into hydrogen and syngas, and thermochemical water splitting. Each technology presents distinct advantages and challenges, but collectively they demonstrate the vast potential for increasing hydrogen production efficiency and sustainability.
"Investments in research and development can lead to breakthroughs that lower production costs, enhancing economic viability."
Further, hydrogen fuel cells represent a significant leap in energy conversion technologies. They allow for efficient energy transfer, powering everything from vehicles to stationary applications. As these technologies mature, the ability to integrate hydrogen into existing infrastructure becomes more plausible. Governments and private enterprises must work together to ensure that these innovations translate into scalable solutions.
Trends in Global Energy Transition
The global energy transition is moving towards low-carbon technologies, and hydrogen occupies a pivotal position in this shift. The rise of hydrogen economies is evident in various countries' policy frameworks, with governments setting ambitious targets for hydrogen adoption. Countries like Germany and Japan are leading the way in creating regulatory environments that facilitate investment in hydrogen-related technologies, providing a template for others to follow.
Furthermore, the shift to hydrogen is not only confined to energy production but also extends to heavy industries. Sectors such as steelmaking and chemicals are exploring hydrogen as an alternative to fossil fuels. This transition signifies a collective move towards decarbonization across multiple sectors, exploiting hydrogen's potential as a versatile energy carrier.
Epilogue
The conclusion is not just a summary, but a vital component that emphasizes the essence of the article. It serves as a bridge that encapsulates the multifaceted benefits of hydrogen. A thorough discussion on hydrogen’s potential impacts across various sectors reinforces its importance. Moreover, this conclusion articulates the pressing challenges and future directions for hydrogen as a key player in the energy landscape.
Summary of Key Points
In this article, we explored a range of significant aspects of hydrogen, including:
- Definition and Properties: Understanding hydrogen’s fundamental characteristics.
- Hydrogen as an Energy Carrier: Mechanisms and applications in transportation.
- Economic Advantages: Analyzing costs, job creation, and production methods.
- Environmental Benefits: The role of hydrogen in reducing emissions and improving air quality.
- Industrial Applications: Uses in various manufacturing sectors.
- Production Technologies: Current methods and innovations that influence hydrogen production.
- Challenges: Identifying infrastructure limitations and addressing safety concerns.
- Policy Framework: Government roles and international treaties impacting hydrogen adoption.
- Future Perspectives: Reviewing emerging technologies and global energy transition trends.
These points collectively detail how hydrogen stands as a multifaceted solution to contemporary challenges, demonstrating its potential as a sustainable energy resource.
Final Thoughts on Hydrogen's Role
The role of hydrogen in the modern energy landscape is more than a theoretical discussion. It signifies a shift towards a more sustainable and efficient energy system. The benefits of hydrogen revolve around its versatility, which can support decarbonization efforts, facilitate energy storage, and contribute to the circular economy. Its ability to integrate with existing infrastructure while promoting innovative technologies further establishes its potential.
Hydrogen is positioned not only as an energy carrier but also as a material that can shift paradigms in energy consumption and production. Investing in hydrogen technology and infrastructure signifies commitment to a cleaner, more resilient energy future. Stakeholders, researchers, and policymakers are encouraged to prioritize hydrogen as a central element of their strategic energy planning.