Exploring FRET: Principles and Applications
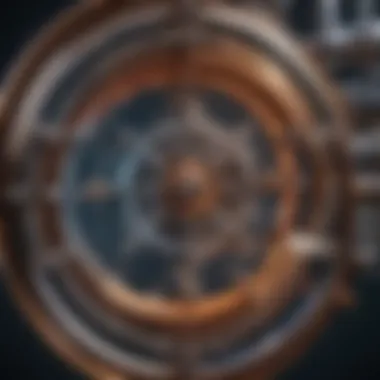
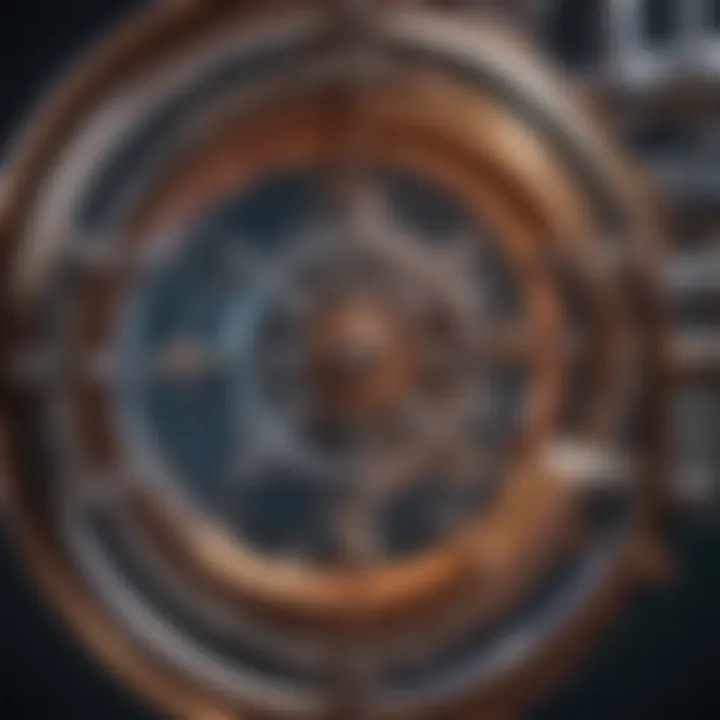
Intro
Forster Resonance Energy Transfer (FRET) represents a critical chapter in the realm of photophysical phenomena, recognized widely in biological and chemical applications. This mechanism fundamentally involves the non-radiative transfer of energy from an excited donor chromophore to an acceptor chromophore. The implications of this process can be felt across various scientific disciplines, where understanding energy transfer is pivotal for advancing knowledge in molecular interactions, biosensing, and imaging.
In this discussion, we will explicate the core principles behind FRET, elucidate its operational mechanisms, and unveil its diverse applications. Emphasis will also be placed on the parameters that influence the efficiency of FRET, along with insights into emerging trends and future directions for its technological integration.
Methodologies
Description of Research Techniques
The exploration of FRET typically involves a range of sophisticated research techniques. Spectroscopic methods, particularly fluorescence spectroscopy, are crucial in studying energy transfer. The techniques such as ensemble FRET and single-molecule FRET provide insight into both average behavior and individual molecular interactions, thereby enriching our understanding of biological systems.
In ensemble measurements, the average fluorescence intensity from a population of molecules is analyzed. This approach allows for the assessment of FRET efficiency, which depends on the distance between the donor and acceptor chromophores, as well as their relative orientations. Conversely, single-molecule FRET allows for a more nuanced view by tracking the interactions of individual molecules in real-time. This specificity lays the groundwork for detailed investigations into dynamic processes at the molecular level.
Tools and Technologies Used
A suite of advanced tools complements the methodologies employed in FRET research. Key instruments typically include:
- Fluorescence microscopes for imaging proteins tagged with fluorescent labels.
- Time-correlated single-photon counting (TCSPC) that measures the time interval between photon arrivals, aiding in precise calculations of FRET efficiency.
- Plate readers for high-throughput screening of FRET assays.
These instruments enable researchers to gather quantitative data, thereby enhancing both the reliability and applicability of their findings.
Discussion
Comparison with Previous Research
FRET has evolved significantly since its initial discovery. Earlier studies primarily focused on elucidating the theoretical underpinnings without delving into extensive applications. Over time, advancements in molecular biology and imaging techniques have enabled researchers to exploit FRET for practical applications, such as mapping protein interactions in living cells and probing changes in conformational states of biomolecules.
Theoretical Implications
The theoretical framework of FRET revolves around several critical factors, including distance, spectral overlap, and quantum yield of the fluorescent molecules involved. A deeper exploration of these parameters not only elucidates the underlying physics but also fosters innovation in designing new probes and improving existing technologies. Researchers are continuously seeking ways to enhance FRET efficiency through novel chromophore developments and structural modifications.
In summary, Forster Resonance Energy Transfer is a nuanced, multifaceted phenomenon. As it permeates various scientific fields, its principles and applications hold the potential to innovate and innovate once more. By addressing the methodologies, tools, and implications of FRET, we lay the groundwork for ongoing exploration in the future.
Prelims to Forster Resonance Energy Transfer
Forster Resonance Energy Transfer (FRET) occupies a central position in the study of molecular interactions, particularly in biological and chemical contexts. This phenomenon provides profound insights into the dynamics of biomolecules, enabling researchers to explore interactions at the nanoscale. Understanding FRET is crucial not only for fundamental research but also for its applications in various fields such as imaging, drug discovery, and biosensing. As science advances, the relevance of FRET becomes more apparent, facilitating breakthroughs in areas like cellular biology and nanotechnology.
Definition and Overview
FRET refers to the non-radiative transfer of energy from an excited donor chromophore to an acceptor chromophore. This transfer is highly efficient when the two chromophores are in close proximity, typically within 1 to 10 nanometers. The efficiency of energy transfer is sensitive to several parameters, such as the distance between the donor and acceptor, their relative orientation, and the spectral overlap of their emission and absorption. An important aspect of FRET is its ability to provide real-time insights into molecular interactions, which is vital for understanding biological processes and developing new technologies.
Historical Context
The concept of FRET emerged from early studies in photophysics. The phenomenon was first described by Hermann Förster in the late 1940s, when he introduced the theory of resonance energy transfer. His work laid the foundation for a deeper understanding of molecular interactions. Over the decades, advancements in fluorescence microscopy and detection techniques have significantly broadened the applications of FRET. Today, it is a pivotal tool used in studies of protein-protein interactions and cellular signaling. FRET's evolution reflects the growing demand for high-resolution techniques that can probe the complexities of molecular biology.
"FRET has become an indispensable tool in modern biology, linking advances in chemistry with the intricacies of living systems."
Fundamental Principles of FRET
Forster Resonance Energy Transfer (FRET) serves as a cornerstone in various research applications, particularly in biological sciences. Understanding the fundamental principles of FRET is crucial as it sheds light on how energy can be transferred between two chromophores, opening avenues for numerous experimental methodologies. The underlying mechanisms of FRET are essential for achieving reliable and reproducible results in diverse contexts such as cellular imaging, protein-protein interactions, and molecular diagnostics. This section elucidates the intricacies of FRET, allowing readers to comprehend the importance of distance, spectral overlap, and temporal aspects that govern energy transfer efficiency.
Energy Transfer Mechanism
The process of energy transfer in FRET involves a donor and an acceptor chromophore. When the donor is excited by light, it can transfer energy to the acceptor without emitting a photon. This happens through dipole-dipole interactions, which operate over a short range, specifically within 1 to 10 nanometers. It is important to note that this mechanism is non-radiative; thus, the efficiency of energy transfer relies on the spatial and spectral properties of both chromophores.
Key factors influencing this mechanism include:
- Orientation of Dipoles: The relative orientation between the donor and acceptor dipoles affects transfer efficiency. A higher overlap of dipole orientations leads to better transfer.
- Distance: The energy transfer efficiency decreases sharply with increasing distance, following a power law relationship. The efficiency can be described using the Förster equation, which models how efficiency drops as distance increases.
- Spectral Overlap: There must be an overlap between the emission spectrum of the donor and the absorption spectrum of the acceptor. A strong spectral overlap enhances the probability of energy transfer.
"The efficiency of FRET is not just a principle of physics; it requires the understanding of molecular interactions to unlock its full potential in practical applications."
Role of Distance and Spectral Overlap
Distance and spectral overlap are pivotal for the effectiveness of FRET. In practical applications, researchers often manipulate these parameters to optimize experiments.
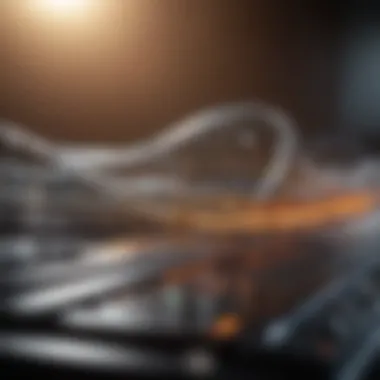
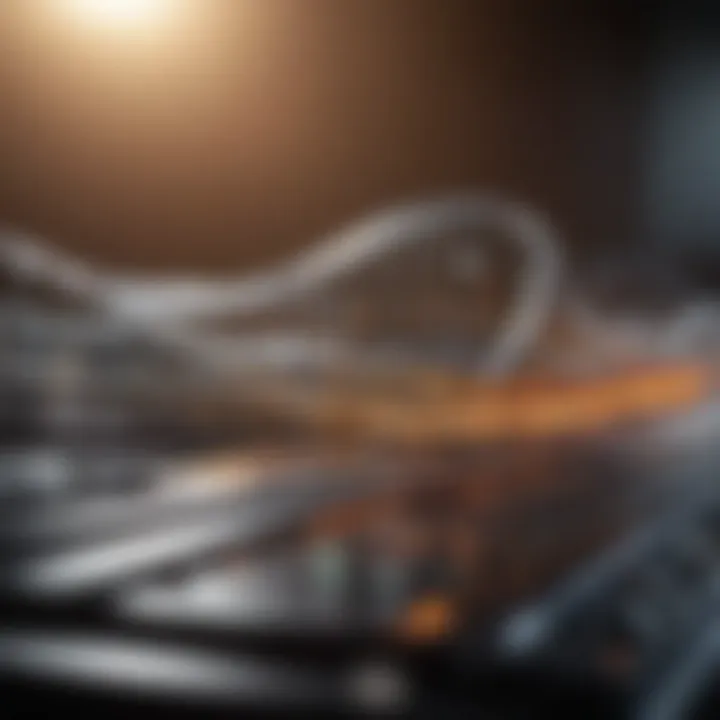
- Distance: The efficiency of energy transfer is highly sensitive to the donor-acceptor separation. The typical range for effective FRET is generally in the range of 1 to 10 nm. At greater distances, the likelihood of energy transfer diminishes significantly. This characteristic allows researchers to use FRET as a measurement tool for studying molecular distances within complex biological systems.
- Spectral Overlap: The overlap integral quantitatively expresses how well the emission spectrum of the donor coincides with the absorption spectrum of the acceptor. Greater overlap intensifies the FRET signal. Thus, selecting appropriate donor and acceptor pairs based on their spectral properties is a crucial step in experiment design.
Time-Resolved FRET Measurements
Time-resolved FRET measurements provide an additional layer of analysis for understanding energy transfer dynamics. This approach involves monitoring the fluorescence decay times of the donor in the presence of the acceptor.
- Fluorescence Lifetime: In FRET experiments, a significant change in fluorescence lifetime of the donor upon interaction with an acceptor is indicative of energy transfer. This allows researchers to differentiate between FRET events and other types of fluorescence quenching.
- Kinetic Analysis: Applying time-resolved techniques enables the assessment of kinetic parameters, which is essential for modeling complex biological processes. By analyzing decay kinetics, researchers can gain insights into conformational changes and interactions within biomolecular systems, thereby advancing our understanding of cellular mechanisms.
The Mathematics of FRET
The exploration of Forster Resonance Energy Transfer (FRET) goes beyond experimental observation. The mathematical framework behind FRET is essential for quantifying the phenomena observed in various applications, particularly in the biological sciences. Understanding the mathematics of FRET aids in predicting outcomes, optimizing conditions, and interpreting data effectively. This knowledge provides a structured approach to analyze the efficiency and dynamics of energy transfer between chromophores, which is vital for advancing research in fields such as molecular imaging and biosensing.
FRET Efficiency Calculations
FRET efficiency is a critical parameter in assessing energy transfer performance. The efficiency of this transfer depends on several factors, including the distance between donor and acceptor molecules, spectral overlap, and the quantum yield of the donor. The equation for calculating FRET efficiency (E) is:
[ E = \frac11 + (R_0/r)^6 ]
Where:
- (R_0) = Forster radius (distance at which FRET efficiency is 50%)
- (r) = distance between donor and acceptor pairs
This relationship defines how energy transfer increases as chromophores approach each other within the optimal distance. Understanding this distance-dependence allows researchers to design experiments and set conditions that maximize energy transfer.
Also, one must consider the spectral characteristics of the donor and acceptor. The overlap integral calculates how well the emission spectrum of the donor overlaps with the absorption spectrum of the acceptor. This overlap is crucial for efficient energy transfer. Accurate efficiency calculations help in making informed decisions regarding the chromophores used in experiments, ultimately influencing experimental outcomes and interpretations.
Kinetic Modeling of Energy Transfer
Kinetic modeling serves as a tool to describe the temporal dynamics of energy transfer processes. The fundamental approach involves setting up differential equations that account for the rates of excitation and transfer between donor and acceptor. By modeling these interactions, researchers gain insights into how various conditions affect FRET kinetics.
A basic model can incorporate variables like:
- Rate of donor excitation
- Rate of FRET
- Rate of non-radiative decay of the donor
Where:
- ([D]) = concentration of donor
- ([A]) = concentration of acceptor
- (k_ex) = rate constant for excitation
- (k_FRET) = rate constant for FRET
- (k_nr) = rate constant for non-radiative decay
This equation illustrates the dynamic interaction between the donor and acceptor and how various factors influence the energy transfer process over time. Kinetic modeling is indispensable, enabling researchers to predict responses to varying experimental conditions and refine their approach accordingly. Such modeling paves the way for practical applications, including those in drug discovery and cellular imaging.
Factors Influencing FRET
Understanding the factors influencing Forster Resonance Energy Transfer (FRET) is essential, as these factors directly impact the efficiency and reliability of the energy transfer process. This knowledge is critical for both theoretical studies and practical applications in multiple scientific fields such as biochemistry and nanotechnology. By analyzing the interplay of various elements, researchers can optimize experimental conditions to achieve accurate and meaningful results.
Chromophore Properties
The intrinsic properties of chromophores play a vital role in FRET efficiency. Chromophores are molecules that absorb and emit light and are fundamental components in FRET systems. Several characteristics of chromophores can significantly affect energy transfer:
- Absorption Spectrum: The overlap between the emission spectrum of the donor and the absorption spectrum of the acceptor is crucial. A higher spectral overlap increases the likelihood of energy transfer, thus enhancing FRET efficiency.
- Quantum Yield: The quantum yield of a chromophore dictates how efficiently it can convert absorbed light into emitted light. Higher quantum yields in the donor chromophore lead to increased energy available for transfer to the acceptor.
- Fluorescence Lifetimes: Longer fluorescence lifetimes in the donor improve FRET, as the donor can transfer energy over a longer time frame before it emits a photon.
By optimizing these properties, researchers can design more effective FRET probes for applications in cellular imaging or molecular interaction studies.
Environmental Conditions
The environment in which FRET occurs is equally significant. Various abiotic factors can influence the interaction between the donor and acceptor chromophores:
- pH Levels: FRET efficiency can vary dramatically with changes in pH, affecting the protonation states of chromophores, which in turn alters their spectral properties.
- Ionic Strength: High ionic strength may influence the arrangement of the chromophores, potentially impacting their proximity and energy transfer rates.
- Temperature: Fluctuations in temperature can change the kinetic properties of the molecules involved. Higher temperatures might lead to increased energy states, affecting the efficiency of FRET.
Adapting experimental conditions to manage these environmental influences is crucial for accurate FRET measurements.
Conformational Changes in Complexes
FRET is heavily dependent on the spatial arrangement of the donor and acceptor. The conformational state of a complex can lead to significant variations in energy transfer efficiency:
- Binding Affinity: When proteins or other molecules bind, their relative positions change. A favorable binding conformation can greatly enhance FRET efficiency by placing donor and acceptor closer together.
- Dynamic Movement: Intrinsic flexibility within a molecular complex can result in fluctuating distances between the donor and the acceptor. This dynamic behavior necessitates interpretation of FRET data with careful consideration of potential changes in conformation during the measurement.
- Allosteric Effects: Changes in one part of a molecule can indirectly affect the positioning of another, thus influencing FRET efficiency.
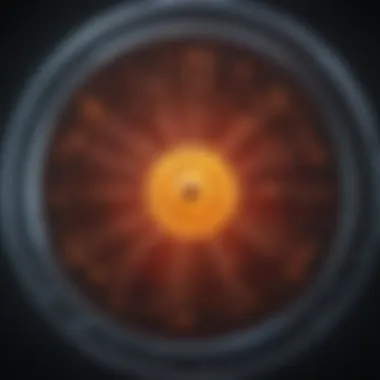
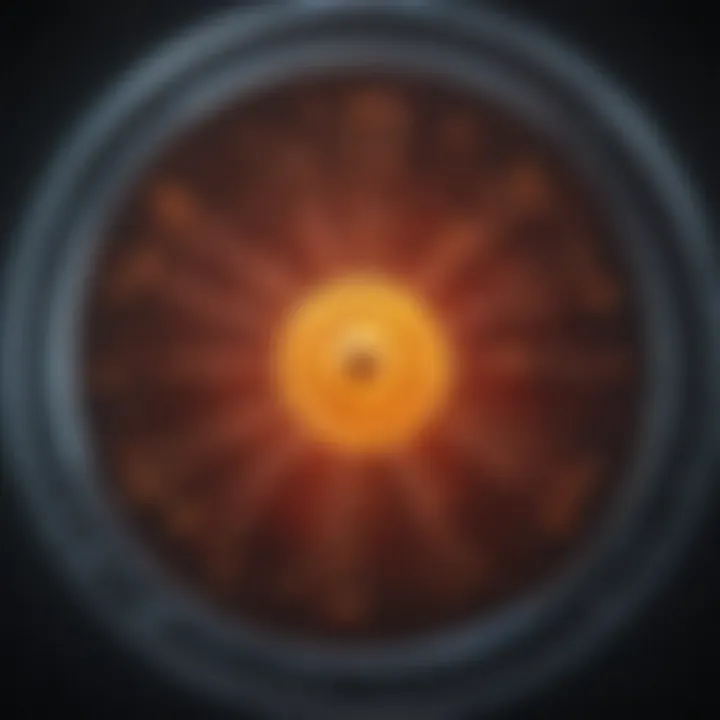
Understanding these conformational dynamics allows for more precise applications of FRET in studying biomolecular interactions and in the design of probes.
FRET is not merely an optical tool; it operates under a complex interaction of various factors, each influencing its efficiency. Recognizing these influences is key to leveraging FRET for advanced research and applications.
Applications of FRET in Biological Sciences
Forster Resonance Energy Transfer (FRET) has fundamentally transformed the approach to studying biological systems. Its applications are far-reaching, and they offer significant insights into molecular and cellular interactions. The use of FRET in biological sciences enhances our understanding of various processes, from protein interactions to cellular mechanisms. This section explores the specific implementations of FRET, highlighting its advantages and considerations in each area of application.
FRET in Protein-Protein Interaction Studies
FRET is widely recognized for its role in elucidating protein-protein interactions. When two proteins of interest are tagged with appropriate donor and acceptor fluorophores, the energy transfer can serve as an indicator of their proximity and interaction. This interaction is typically quantified through FRET efficiency, which is influenced by the distance between the donor and acceptor.
One notable advantage of using FRET in these studies is its ability to provide real-time information about dynamic interactions within living cells. Traditional methods, such as co-immunoprecipitation, offer limited insights into temporal dynamics. FRET offers higher resolution and temporal resolution, making it a more flexible option. Researchers can obtain kinetic data on interactions and monitor changes in response to different stimuli or experimental conditions.
Applications in Cellular Imaging
Another significant application of FRET is in the field of cellular imaging. By utilizing fluorophores that demonstrate FRET, researchers can visualize not just static structures but also dynamic processes within cells. FRET can be employed in investigating subcellular compartments, protein localization, and signaling pathways. This technique allows for the study of various biological events at the cellular level with remarkable specificity.
The process of imaging can yield valuable information about physiological changes in live cells. This is particularly useful in fields such as cancer research, where understanding the localization and interaction of proteins can indicate abnormal cellular functions.
Overall, FRET in cellular imaging provides a non-invasive way to monitor cellular activities in real time, possibly leading to new insights into cellular mechanisms and behaviors.
Role of FRET in Drug Discovery
FRET is increasingly being applied within the context of drug discovery. It can potentially streamline the search for new pharmacological agents by providing insights into the interactions between potential drug candidates and their targets. Observing how a drug affects the interaction between proteins can aid in understanding its mechanism of action and efficiency.
Using FRET, researchers can assess how drugs alter the dynamics of protein interactions, identifying potential therapeutic targets. Additionally, this technique can be crucial in high-throughput screening assays, reducing the time and resources needed to test numerous compounds quickly.
"FRET not only aids in understanding the fundamental properties of biomolecules but also pushes the boundaries of innovation in drug discovery methodologies."
FRET's integration into drug discovery processes exemplifies its relevance and versatility in advancing biological research. In summary, the applications of FRET in biological sciences are extensive and critical for advancing our knowledge across numerous fields. The combination of real-time analysis, spatial resolution, and its usability in various contexts renders FRET a valuable tool for researchers and educators, ultimately fostering deeper insights into molecular biology.
FRET in Materials Science
Forster Resonance Energy Transfer (FRET) has a considerable role in materials science. Its principles can improve the design and functionality of various materials. Notably, FRET can enhance the properties of nanomaterials and integrate them into advanced photonic devices. Understanding the applications of FRET in this field allows scientists and engineers to create innovative materials with unique features. Moreover, it fosters interdisciplinary research that connects biology, chemistry, and material engineering.
Nanomaterials and Surface-Enhanced FRET
Nanomaterials hold substantial promise in advancing FRET applications. Their unique physical and chemical properties facilitate better interaction between donor and acceptor chromophores. As a result, the efficiency of energy transfer increases. Surface-enhanced FRET occurs when metal nanostructures, like gold or silver nanoparticles, are utilized to amplify the local electromagnetic field. This enhancement allows for more effective coupling between the energy donor and acceptor.
- Key benefits of nanomaterials in FRET:
- Improved signal-to-noise ratio
- Increased sensitivity in biosensing applications
- Enhanced resolution in imaging techniques
Additionally, the size and shape of these nanomaterials can be engineered to optimize FRET conditions. Researchers can manipulate these characteristics to tailor the energy transfer properties as desired.
Integration in Photonic Devices
The integration of FRET with photonic devices is another significant development in materials science. Photonic devices utilize light to convey information across various applications. Implementing FRET into these systems can enhance performance significantly. The ability to control the flow of energy can lead to advancements in telecommunications, sensors, and imaging technologies.
- Applications of FRET in photonic devices include:
- Development of high-efficiency light-emitting diodes
- Creation of labels for optical sensors
- Innovations in quantum dot applications for solar cells
The advantages of using FRET in photonic devices are clear. Enhanced sensitivity, improved energy conversion efficiency, and reduced operational costs are just a few benefits.
FRET enhances the capabilities of materials science by enabling the creation of innovative, multifunctional materials. Understanding its principles is crucial for pushing the boundaries of current technologies.
Technological Innovations in FRET
Technological innovations in Förster Resonance Energy Transfer (FRET) play an essential role in expanding the capabilities and applications of this phenomenon. As the demand for more precise and efficient techniques in scientific research increases, advancements in FRET technologies cater to these needs. Understanding these innovations is crucial for researchers aiming to leverage FRET in various fields such as biology, chemistry, and materials science.
The advancements focus on improving sensitivity and specificity, making it possible to study complex biological systems with greater detail. Additionally, these innovations aim to streamline experimental procedures and enable the analysis of a larger number of samples in less time. Therefore, the significance of these innovations cannot be overstated, as they enhance the effectiveness of FRET applications in experimental and practical scenarios.
Advancements in FRET Probes
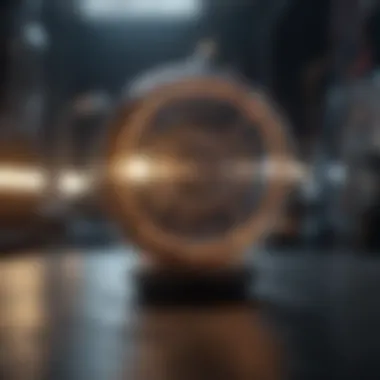
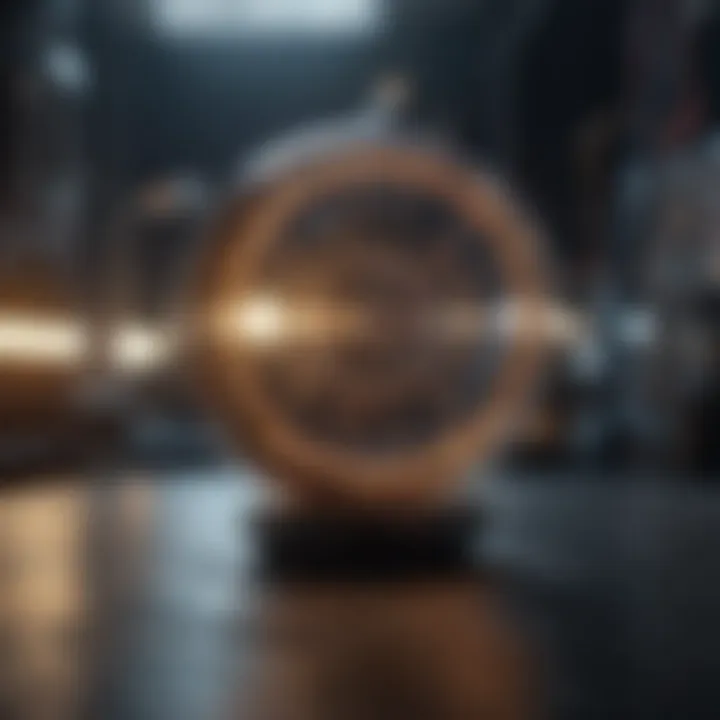
The development of novel FRET probes has been a game changer in many research areas. Modern probes are designed to have improved photophysical properties, increasing their brightness and stability, which directly affects the efficiency of energy transfer. For example, new types of fluorescent proteins and synthetic dyes have emerged, offering better spectral characteristics. These advancements facilitate higher resolution imaging and more reliable quantification of biomolecular interactions.
Moreover, multifunctional probes that can integrate multiple FRET pairs in a single construct have been introduced. These constructs allow for simultaneous monitoring of several interactions or processes, providing a comprehensive view of cellular dynamics.
The continuous refinement of these FRET probes contributes significantly to our understanding of complex biological phenomena, allowing for real-time observation of processes at the molecular level.
High-Throughput FRET Screening Techniques
High-throughput FRET screening techniques represent another crucial innovation in the field. These methods enable researchers to conduct extensive screenings of samples in a relatively short time frame. This ability is particularly valuable in drug discovery and the screening of potential therapeutic compounds.
Recent development of automated systems that integrate FRET screening provides significant advantages. Such systems streamline the data collection process while minimizing operator errors, enhancing reproducibility. The application of robotics ensures that high volumes of samples are processed efficiently, leading to faster identification of candidate molecules.
Additionally, advancements in software that analyzes FRET data contribute to improved accuracy in quantifying interactions. For example, sophisticated algorithms can model complex interactions in real-time, providing insights that were not previously accessible.
High-throughput FRET techniques have revolutionized the way researchers approach screening and characterization of biomolecular interactions, dramatically increasing the pace of discovery.
Limitations and Challenges of FRET
The exploration of Forster Resonance Energy Transfer (FRET) reveals not only its potential but also the limitations that researchers must navigate. Understanding these challenges is crucial for advancing FRET applications effectively. Successful integration of FRET techniques depends heavily on addressing these limitations. There are two primary areas of concern: difficulties in data interpretation and technological constraints.
Challenges in Interpretation of FRET Data
Data interpretation stands as a significant hurdle in FRET studies. FRET efficiency is sensitive to various factors, such as the donor-acceptor pair, their relative orientations, and environmental conditions. These parameters can lead to misinterpretations, particularly when experimental conditions are not well-controlled. The following are critical issues faced in the interpretation process:
- Variability in Background Fluorescence: High background signals can obscure true FRET signals, complicating the results.
- Non-specific Interactions: The presence of unintended interactions among chromophores may lead to false positives in FRET efficiency calculations.
- Distance Dependency: Small changes in distance between donor and acceptor can dramatically change the FRET efficiency. This creates challenges when trying to correlate energy transfer to specific molecular conformations or interactions.
Due to such intricacies, it is vital for researchers to utilize robust controls and validation methods when analyzing FRET data. This becomes essential to mitigate pitfalls and improve the reliability of the conclusions drawn.
Technological Constraints
While FRET serves as a powerful tool in myriad applications, its implementation is limited by certain technological constraints. These limitations can restrict the precision and reliability of FRET measurements. Key constraints include:
- Instrumentation Limitations: Not all fluorescence spectroscopy systems are equipped to handle the complexities of FRET. Specialized equipment may be necessary to obtain high-quality data.
- Photostability of Probes: Chromophores used in FRET must exhibit high photostability. Compromised stability can lead to signal loss, derived from photobleaching, affecting the quantitative aspect of experiments.
- Efficiency of Probe Pair Selection: The choice of donor-acceptor pairs can influence the efficiency of energy transfer. Inadequate selection may yield limited or unreliable data.
To address these technological hurdles, advancements in probe design and instrumentation continue to progress, enabling more accurate applications of FRET in research.
Continuous improvement in technology and data analysis methods is essential for maximizing the potential of FRET, paving the way for groundbreaking applications.
In summary, recognizing and addressing the limitations and challenges of FRET is pivotal for ensuring accurate results. Researchers are encouraged to maintain awareness of these factors, guiding their investigations toward more reliable outcomes.
Future Directions in FRET Research
The field of Forster Resonance Energy Transfer (FRET) research continues to evolve, reflecting the growing interest among scientists in exploring new applications and refining existing methods. As our understanding of molecular interactions deepens, the implications for various scientific disciplines remain profound. This section highlights crucial aspects of future directions in FRET research, considering emerging technologies and potential benefits that can arise from innovative applications.
Emerging Applications in Synthetic Biology
Synthetic biology represents a frontier in biotechnology, where FRET has the potential to be transformative. Scientists are exploring ways to integrate FRET into engineered biological systems. By harnessing FRET, researchers can monitor gene expression, protein folding, and other cellular processes in real-time.
Some innovative applications may include:
- Gene Circuit Monitoring: FRET can effectively visualize and quantify the activities of synthetic gene circuits, offering insights into their behavior under various conditions.
- Protein Interaction Mapping: Using FRET-based biosensors that respond to protein interactions enables the study of complex biological systems with greater detail than traditional methods.
- Cellular Pathway Tracing: FRET can aid in tracing metabolic pathways, revealing how cells respond to environmental changes by mapping energy transfer between fluorescent proteins.
The adaptability of FRET in synthetic biology holds promise for advancements in genetic engineering and metabolic engineering. The integration of FRET can enable feedback loops in biological systems, making it possible to optimize processes such as biofuel production or drug synthesis.
Potential in Drug Delivery Systems
FRET's unique properties may also be employed to improve drug delivery systems. In this context, FRET can serve multiple functions, such as monitoring the release of therapeutic agents or assessing their interaction with cellular targets.
Key possibilities include:
- Targeting Mechanisms: By tagging drugs or nanoparticles with FRET donor-acceptor pairs, researchers can monitor the specificity of drug delivery to target cells, enhancing therapeutic efficiency.
- Real-Time Monitoring: With the aid of FRET, it becomes feasible to measure the kinetics of drug release from carriers. This real-time data can lead to improved dosing strategies and patient outcomes.
- Supramolecular Systems: FRET can facilitate the design of supramolecular systems that respond to biological signals, allowing for controlled drug release based on environmental triggers.
Culmination
The conclusion of this article synthesizes the wealth of information presented throughout, emphasizing the critical understanding and practical implications of Forster Resonance Energy Transfer (FRET). This photophysical phenomenon is not only pivotal in academic research but also possesses significant potential across various scientific fields. As highlighted earlier, FRET's ability to elucidate molecular interactions is invaluable, enabling advancements in biosensing and imaging technology.
Summary of Key Points
- FRET operates based on the energy transfer between two chromophores, dependent on factors such as distance, spectral overlap, and environmental conditions.
- The mathematical framework surrounding FRET efficiency calculations lays the groundwork for interpreting data accurately, which is essential for researchers.
- Applications of FRET span numerous domains, including cellular imaging, protein interactions, and drug discovery, demonstrating its versatility and importance in practical settings.
- Advancements in technology and probe design continue to enhance the capabilities of FRET, addressing many historical limitations.
- The exploration of future directions, such as synthetic biology and drug delivery systems, showcases the ongoing evolution and relevance of FRET in research.
Final Thoughts on the Future of FRET
Looking ahead, FRET presents an exciting landscape for further inquiry. The promise of emerging applications signals a new era where sophisticated experimental techniques can prob deeper into molecular dynamics. The integration of FRET with cutting-edge technologies may lead to groundbreaking discoveries in molecular biology and materials science. As researchers embrace these advancements, the potential for FRET to impact fields such as personalized medicine and therapeutic development becomes increasingly evident.