Carrier Controllers: Functions, Applications, and Future Trends
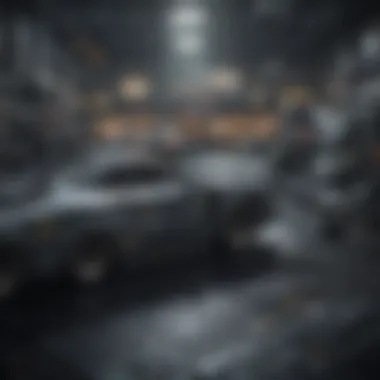
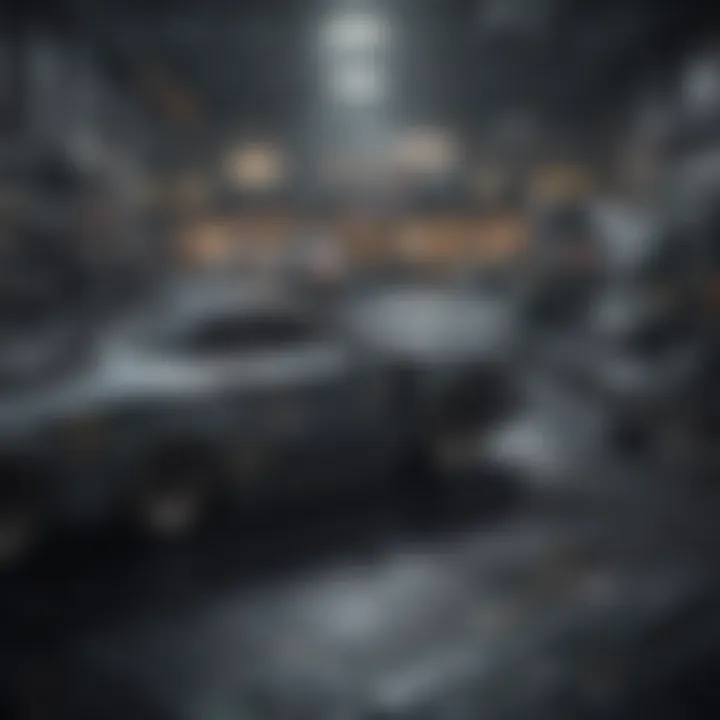
Intro
Carrier controllers play a pivotal role in various scientific domains, governing the movement and behavior of carriers—such as electrons, ions, and excitons—in numerous applications. The significance of these controllers extends beyond simple management; they are integral to enhancing system performance and achieving optimal functionalities in fields such as semiconductor physics, materials science, and quantum computing.
Understanding carrier controllers requires a nuanced comprehension of their underlying principles and operational mechanisms. These devices enable precise regulation of carrier dynamics, thus facilitating advancements in technology and science. In this exploration, we will dissect the functionalities of carrier controllers, evaluate their applications, and address their implications across different fields, contributing to a deeper understanding of their roles.
This article aims to provide readers, including students, educators, and industry professionals, with a thorough analysis of carrier controllers. We will delve into current research trends, technological advancements, and future directions that promise to reshape how we perceive and utilize these devices. By articulating this complex subject matter clearly, we hope to spark further inquiry and enhance the knowledge surrounding carrier controllers.
Methodologies
In examining the depths of carrier controllers, several research methodologies underpin the analysis. The choice of methodology significantly influences the outcomes of the study, providing both qualitative and quantitative data on the functionality and applications of these controllers.
Description of Research Techniques
Research on carrier controllers often involves a variety of techniques, including but not limited to:
- Experimental Techniques: Laboratory-based experiments where various parameters are manipulated, allowing for observation of carrier dynamics under controlled conditions.
- Simulation Approaches: Computational models simulating carrier behavior in different environments provide insights into expected outcomes without necessitating extensive physical experiments.
These methodologies enable researchers to build a comprehensive understanding of carrier behaviors and the effects of different control mechanisms.
Tools and Technologies Used
The investigation of carrier controllers utilizes an array of tools and technologies. Notable examples include:
- Field-Effect Transistors (FETs): For understanding controlled carrier transport in semiconductor devices.
- Spectroscopic Techniques: Such as Raman and photoluminescence spectroscopy, which assist in analyzing the properties of carriers.
- Advanced Software: Tools like COMSOL Multiphysics for simulations and Electromagnetic Field (EMF) modeling are essential for studying carrier behavior under varying conditions.
By leveraging these tools, researchers achieve a finer grasp of the mechanics governing carrier controllers.
Discussion
Comparison with Previous Research
A comparative analysis reveals that while foundational principles of carrier dynamics remain consistent, significant advancements have emerged in recent years. Contemporary research increasingly focuses on integrating artificial intelligence and machine learning for optimizing the functionalities of carrier controllers. Previous studies lacked this integration, often relying solely on traditional methods.
Theoretical Implications
The implications of these advancements are profound. Not only do they enhance performance metrics, but they also extend the applicability of carrier controllers across various disciplines. The evolution of theoretical frameworks surrounding carrier dynamics can lead to innovative applications in fields like nanotechnology and quantum electronics, demonstrating the versatile nature of these devices.
Understanding Carrier Controllers
The study of carrier controllers is a significant area within the domains of engineering and technology. They play a crucial role in a variety of systems that require precise control and optimization. Their relevance spans different applications, including electronics, automation, and communication systems. A clear understanding of carrier controllers enables professionals and researchers to design and implement effective solutions in an increasingly sophisticated technological landscape.
Carrier controllers function effectively by managing the behavior of carriers, which can be understood as particles or signals that convey information or energy in a specific medium. The manipulation of these carriers is paramount for achieving desired outcomes in many technological processes. Understanding the principles behind these controllers helps in optimizing performance, reducing errors, and improving system reliability.
Definition and Purpose
A carrier controller is an electronic device or system designed to regulate the flow of carriers in a given application. The primary goal of such controllers is to maintain a desired behavior or output, whether it be in signal processing, data transmission, or other operational contexts. These controllers can adjust parameters like frequency, amplitude, or other relevant characteristics based on the feedback they receive.
The purpose of carrier controllers is multifaceted. They ensure stability and efficiency in systems, which are essential for operations in critical industries. By providing feedback mechanisms, these controllers adapt to changing conditions, thereby enhancing overall system performance. Their design can vary significantly, depending on the specific application and requirements.
Historical Context
The concept of carrier controllers traces back to early advancements in control systems theory. Over the decades, there have been notable developments, particularly with the advent of analog and digital technologies. Early versions relied on simple mechanical or electrical methods to regulate carriers, which gradually evolved into more sophisticated electronic controllers.
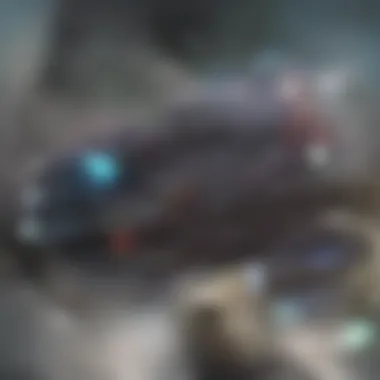
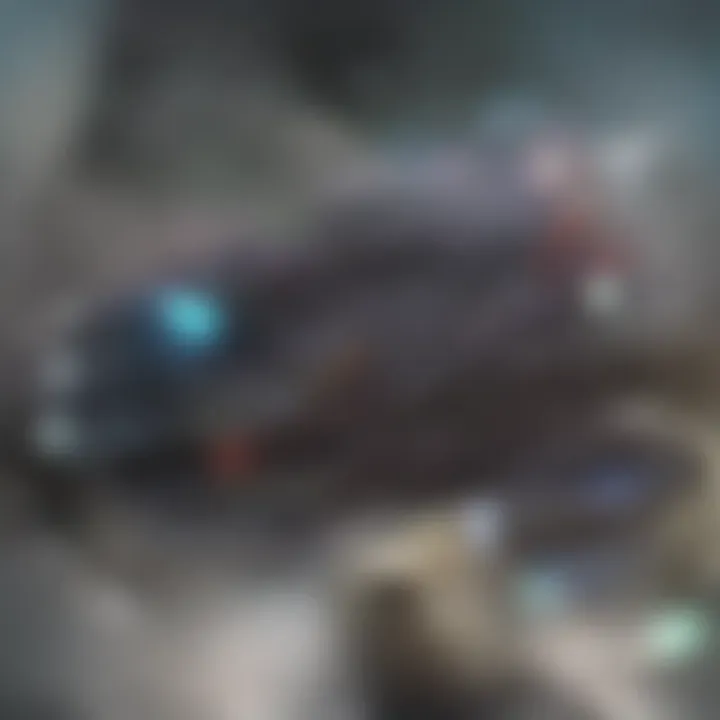
In the latter half of the 20th century, with the emergence of microprocessors and digital signals, carrier controllers became more precise and versatile. This era marked a significant shift in how control systems were designed, with digital control offering enhanced capabilities and flexibility. The increasing demand for automation in various sectors further propelled innovations in this field.
Today, as industries continue to evolve with the integration of artificial intelligence and machine learning, the design and functionality of carrier controllers are also advancing. Researchers are exploring new methods and technologies to improve their responsiveness and efficiency in complex environments. The historical context helps to appreciate the trajectory of carrier controller development and its pivotal role in modern science and engineering.
Fundamental Principles of Operation
Understanding the fundamental principles of operation for carrier controllers is essential for grasping their significance and functionality. These principles provide a framework for how these systems function, manage, and optimize various applications across different fields. The focus here is on carrier dynamics and control mechanisms, which are pivotal in enhancing system performance and ensuring efficient operation of complex systems.
Carrier Dynamics
Carrier dynamics refer to the behavior of carriers, such as electrons in semiconductors, as they interact within different environments. Understanding carrier dynamics is critical for designing effective carrier controllers.
- Key Factors Affecting Dynamics:
- Interaction with electric fields
- Temperature variations
- Material properties
These factors influence how carriers move, which in turn affects the performance of the entire control system. Designers must account for these interactions to create robust and responsive controllers. Importantly, by focusing on carrier dynamics, engineers can develop strategies to mitigate issues like drift and noise, which can disrupt system performance.
Control Mechanisms
Control mechanisms are the processes employed to manage the behavior of carrier controllers. They ensure that the output of the system aligns with desired performance metrics. Two predominant mechanisms are feedback control and feedforward control.
Feedback Control
Feedback control is a process where the controller continuously monitors the output and makes adjustments based on differences between the desired and actual outputs. This mechanism offers several benefits:
- Stability: By adjusting the process in response to actual conditions, feedback control helps maintain system stability.
- Adaptability: It can accommodate variations in system parameters, making it useful in dynamic environments.
However, feedback control can introduce delays in response time, particularly if the system experiences rapid changes. It is often popular in systems that require high precision, such as temperature regulation in industrial processes.
Feedforward Control
Feedforward control is a proactive approach that anticipates disturbances and compensates for them before they affect the system. This mechanism relies on knowledge of the system and its environment.
- Anticipation: One of its main characteristics is the ability to predict disturbances based on input data.
- Speed: Because it does not rely on output measurements, feedforward control can react more quickly than feedback control.
However, feedforward control requires an accurate model of the system. If this model is incorrect, the system can perform poorly. Despite this drawback, it is a valuable choice for applications where speed and quick adaptation are crucial, such as in high-speed manufacturing processes.
Types of Carrier Controllers
Understanding the types of carrier controllers is crucial for grasping their varied applications and efficiencies. From recommending suitable controller choices for specific scenarios to recognizing their operational paradigms, this section lays the groundwork for advanced discussions in automation, communication, and electronics. Each type comes with its own advantages and disadvantages, influencing system performance in different domains.
Analog vs Digital Controllers
Characteristics of Analog Controllers
Analog controllers function by processing a continuous set of input signals. They operate on the principle of proportional control, where the output is directly correlated to the input. This characteristic enables them to manage systems where precise signal representation is imperative. Their performance is often favored in environments where noise is minimal, and signal integrity is crucial.
A key characteristic of analog controllers is their simplicity. They usually consist of operational amplifiers, resistors, and capacitors that are easy to implement in various systems. This simplicity can make them a beneficial choice for certain applications—especially those with lower complexity. However, the unique feature of analog controllers lies in their ability to facilitate real-time adjustments. This is crucial when immediate feedback is necessary for controlling temperature, speed, or position substantially.
Despite their benefits, analog controllers do have limitations. They can be susceptible to noise, which may affect performance in environments with high electromagnetic interference. Moreover, analog controllers often require more space due to their hardware components.
Advantages of Digital Controllers
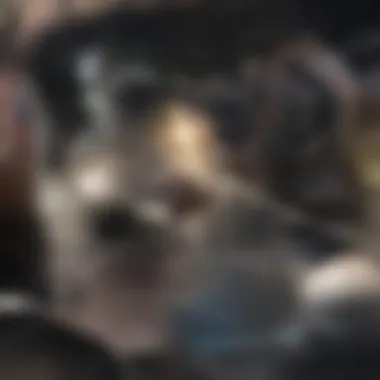
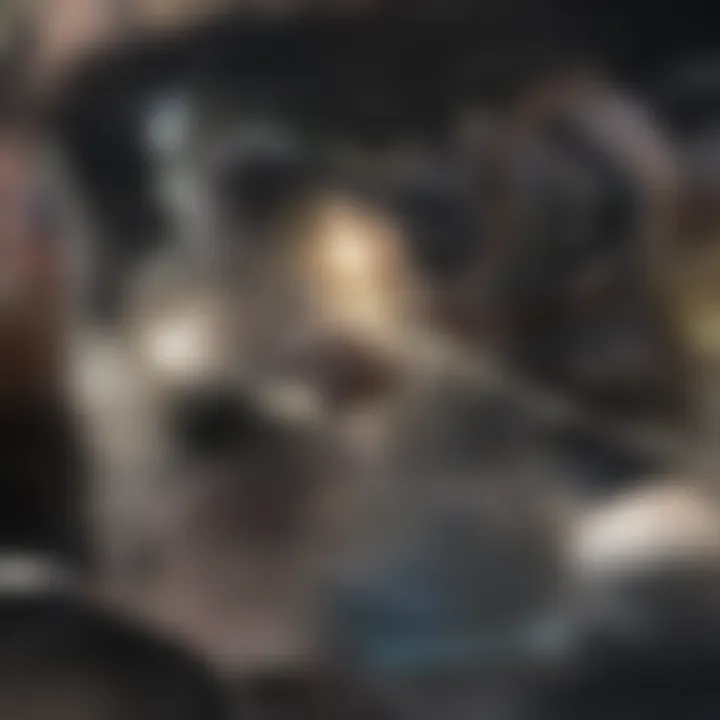
Digital controllers are increasingly becoming the standard due to their flexibility and capability. They convert analog signals into a digital format before processing. This digitization allows for precise control strategies, enhancing system performance.
A primary advantage of digital controllers is their programmability. With this feature, operators can alter the control parameters without needing to redesign hardware, providing adaptability. Digital controllers typically enable more complex computational techniques, such as PID (Proportional-Integral-Derivative) control, making them popular choices across a range of industries.
Another remarkable benefit of digital controllers is their robustness against noise. Because they operate on discrete signals, they are generally less affected by electromagnetic interference. However, one must consider the unique feature of increased processing time. The need for an Analog-to-Digital conversion may introduce some latency, which could be a disadvantage in time-sensitive applications.
Proportional, Integral, and Derivative Controllers
Proportional, Integral, and Derivative (PID) controllers integrate three fundamental control actions to enhance system stability and response. Proportional control adjusts the output proportionately to the error signal, providing a moderate fan-out effect in control.
The Integral component addresses the accumulated error over time, effectively eliminating steady-state errors that may persist under proportional control alone. Meanwhile, the Derivative function predicts future errors based on the current rate of change, improving system responsiveness and stability.
Using PID controllers can optimize performance in various applications such as robotics and process control. This combination helps balance control performance while minimizing overshoot and oscillations.
With this coherent understanding of carrier controller types, one can delve into their applications and technological significance in the current landscape.
Applications of Carrier Controllers
The realm of carrier controllers extends far beyond theoretical frameworks; it significantly impacts real-world applications. Understanding their applications is essential for delving into the intricacies of technology and systems that enhance everyday life and specialized industries. Carrier controllers play a vital role in improving performance, ensuring reliability, and facilitating innovation across various sectors. Their versatility makes them indispensable tools, driving advancements and efficiency in numerous fields.
In Electronics and Communication
Carrier controllers serve a critical function in electronics and communication systems. They regulate signal processing, ensuring that data transmission remains stable and reliable. In radio frequency systems, for example, these controllers maintain signal clarity and reduce noise interference. This precision is especially crucial in environments with high electromagnetic interference.
Moreover, in the realm of telecommunications, carrier controllers help manage bandwidth and optimize the flow of data. By implementing advanced algorithms, they can dynamically adjust resources to meet changing demands. This adaptability not only enhances user experience but also maximizes network efficiency. The integration of digital carrier controllers further strengthens communication systems, providing robust performance under various conditions and ensuring higher rates of data transfer.
In Robotics and Automation
In robotics and automation, carrier controllers are fundamental to the operation and precision of robotic systems. These controllers facilitate motion control, allowing robots to perform tasks with exceptional accuracy. The feedback mechanisms employed in these systems ensure that movements conform to the programmed parameters, making them essential in applications such as manufacturing and assembly.
Furthermore, carrier controllers contribute to autonomous navigation in robots. For example, self-driving vehicles rely heavily on carrier controllers to interpret sensor data, adjust speeds, and safely maneuver through different environments. The continuous flow of information between the sensors and the controller is critical for real-time decision-making. As the field progresses, research into applying artificial intelligence with carrier controllers is paving the way for even more advanced robotics.
In Transportation Systems
Carrier controllers are also integral to modern transportation systems. They enhance traffic management and safety by controlling signals and monitoring vehicle flow. Implementing efficient carrier controllers reduces congestion and minimizes delays, contributing to overall system reliability. For instance, in intelligent traffic signal systems, these controllers process real-time data from various sensors to optimize traffic light sequences, adapting to current conditions.
In public transportation, carrier controllers oversee operations such as train signaling systems, ensuring safety and punctuality. The transition to smart transportation systems has further emphasized the necessity of sophisticated carrier controllers that can integrate with various technologies, including GPS and real-time monitoring systems. Overall, the applications of carrier controllers span numerous fields, demonstrating their critical role in enhancing the functionality and efficiency of various technologies connected to daily life.
Current Trends in Research
The exploration of carrier controllers has entered a new phase characterized by significant advancements and dynamism. Current trends in research focus on enhancing the efficiency and applicability of these systems across various domains. With evolving technologies, researchers are compelled to examine how these controllers can adapt and integrate with advanced functionalities. This keeps them relevant in today's fast-paced technological landscape.
Development of Smart Controllers
The demand for smart controllers has been on the rise. These are developed to enhance the automation and control capabilities of traditional carrier controllers. Smart controllers utilize sensors, modern algorithms, and networking capabilities. This allows for real-time monitoring and decision-making. The integration of Internet of Things (IoT) devices plays a crucial role here. It enables carriers to communicate across networks, optimizing performance and energy consumption, which is increasingly critical in industries like manufacturing and transportation.
One of the main benefits is the ability to analyze data from multiple sources. Smart controllers can adjust their operation based on real-time data, leading to improved responsiveness and accuracy. As industries strive for greater efficiency, applying smart technologies becomes vital. The incorporation of machine learning algorithms is also changing the landscape of control systems. They can predict failures and adapt operations proactively, enhancing overall reliability and uptime.
Integration with Artificial Intelligence
The integration of Artificial Intelligence with carrier controllers marks another significant research trend. AI enhances the decision-making processes within controllers. This approach can lead to more intelligent systems that learn from past operations and continuously improve their functionality. AI-driven models allow for the automation of complex tasks, which were traditionally challenging due to their variability and unpredictability.
For example, using AI algorithms, carrier controllers can start to anticipate system responses based on historical data trends. This reduces human error and increases system precision. The potential for AI in carrier controllers also extends to fault detection and diagnosis, whereby the system can identify issues before they escalate, improving maintenance strategies.
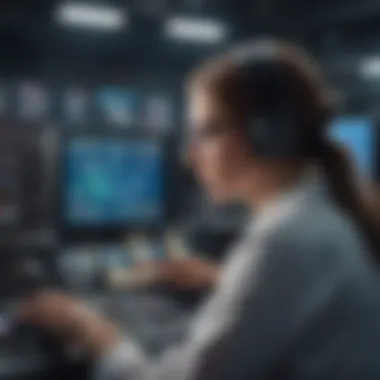
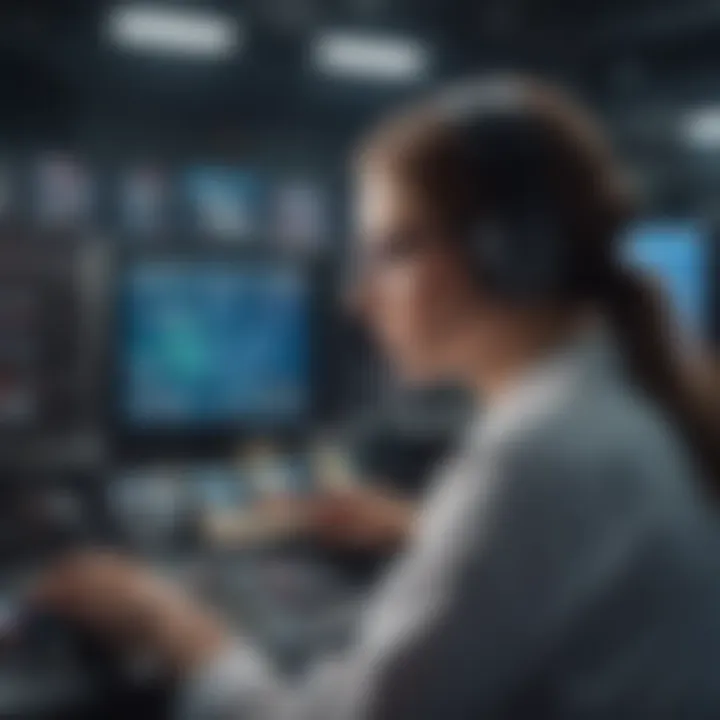
"The synergy of AI and carrier controllers is paving the way for systems that are not only reactive but also predictive in nature. This shift promises substantial enhancements in operational efficiency."
As the research progresses, the necessity to address ethical considerations surrounding AI deployment needs emphasis. Transparency, accountability, and reliability must underpin these innovations to ensure the development of trustworthy systems.
In summary, examining current trends in carrier controller research reveals a concerted effort towards integrating smart technologies and artificial intelligence. These advancements not only increase efficiency but also improve the sustainability of operations across various sectors.
Technological Advancements
The importance of technological advancements in carrier controllers cannot be overstated. They are critical for enhancing the performance, efficiency, and adaptability of these systems across various applications. As technology progresses, carrier controllers become more sophisticated, allowing for improved control over dynamic systems. This has significant implications for fields like telecommunications, robotics, and transportation.
One of the notable aspects of technological advancement is the miniaturization of control system components. This can lead to lighter and more compact devices, which are essential for applications where space and weight are constraints. Increased integration of more features into single chips also improves efficiency, simplifying design and reducing production costs while enhancing reliability.
Moreover, advancements in algorithms play a crucial role. Modern control systems utilize advanced algorithms to optimize performance. The incorporation of machine learning techniques enables the systems to adapt to changing conditions and improve over time. This dynamic adjustment is particularly valuable in environments that are unpredictable, such as autonomous vehicles or industrial automation.
"The future of carrier controllers lies in their ability to learn from data and adjust dynamically to various scenarios, leading to unprecedented levels of efficiency and reliability."
Key benefits to highlight include:
- Real-time performance: Enhanced computational power allows for quicker data processing and decision-making, directly influencing the responsiveness of the control system.
- Energy efficiency: Improved control strategies often lead to significant energy savings, making systems more sustainable.
- Scalability: New technologies allow for easier scaling of systems, whether in manufacturing or precision agriculture, particularly when integrating Internet of Things capabilities.
Nevertheless, the integration of new technologies into existing frameworks poses challenges. Compatibility with legacy systems must be considered, as older infrastructure may hinder the implementation of advanced features. The upfront costs of adopting these technologies can also be a deterrent, especially if organizations do not anticipate a clear return on investment.
Recent Innovations in Control Systems
Recent innovations have significantly transformed the landscape of carrier controllers. One of the prominent trends is the development of cloud-based control systems. These systems leverage cloud computing resources to operate more complex algorithms without the constraints of local processing power. This also enables remote monitoring, updates, and maintenance, which are crucial for systems deployed in inaccessible locations.
In addition to this, advancements in sensor technology are noteworthy. Modern sensors are more sensitive and offer higher data accuracy, allowing controllers to make better-informed decisions. This improvement fosters enhanced precision in areas such as robotics, where exact movements are fundamental for success.
Another interesting innovation is the introduction of flexible and adaptive control techniques, which allow systems to learn from their environments in real-time. The controllers equipped with such techniques can adjust their responses based on varying inputs, leading to better overall performance.
Furthermore, innovation in communication protocols offers benefits such as improved data transfer rates and reduced latency. Technologies like 5G play a significant role in enhancing the communication loop between controllers and their operating environments, resulting in more effective management of dynamic systems.
Future Directions and Challenges
Looking forward, the trajectory of technological advancements in carrier controllers is likely to head towards greater integration of artificial intelligence. As AI continues to improve, we can expect carrier controllers to become even more autonomous. This transition may enable fully autonomous systems in various sectors such as transportation and healthcare.
Despite the promise AI holds, there are challenges ahead. Data privacy concerns are paramount, especially when sensitive information is gathered and analyzed by these autonomous systems. Regulatory frameworks will need to catch up with these advancements to ensure that ethical standards are upheld.
Additionally, as systems become more complex, the demand for specialized skills in operation and maintenance will increase. The workforce must adapt to keep pace with technological changes, which could lead to a skills gap in certain sectors.
Finally, ensuring cybersecurity is a pressing challenge. As systems gain connectivity and become more integrated into the broader internet ecosystem, they also become more susceptible to cyber threats. Establishing robust security measures will be essential to safeguard these systems and their functionalities.
Closure
In this article, we have examined carrier controllers and their significant role across various fields. These controllers serve critical functions in maintaining system stability and performance. The analysis highlights several key elements that stand out in understanding the value of carrier controllers.
Summary of Key Insights
Carrier controllers are essential for effectively managing dynamic systems. They employ diverse control mechanisms, including feedback and feedforward strategies. Below are key insights drawn from our exploration:
- Control Mechanisms: Understanding both feedback and feedforward control mechanisms is vital. Feedback control reacts to changes in system states, while feedforward control anticipates disturbances, providing a more proactive approach.
- Technological Impact: The integration of artificial intelligence into carrier controllers has the potential to revolutionize applications in fields such as robotics and transportation. Smart controllers can adapt in real time, improving efficiency and adaptability.
- Diverse Applications: From electronics to automation, carrier controllers find relevance across many domains. Their ability to optimize system performance is invaluable in enhancing operational capabilities.
In summary, these insights elucidate the importance of carrier controllers in modern technology and their continued evolution.
Call for Further Exploration
As we conclude, it is clear that the study of carrier controllers opens up numerous avenues for future research. Questions remain about how these systems can be further optimized:
- Integration Challenges: There are still hurdles in effectively integrating advanced algorithms with existing systems. Understanding these challenges can lead to better performance and reliability.
- Ethical Considerations: As smart controllers become prevalent, ethical considerations regarding their deployment must be examined. How these technologies impact society is a critical topic for future discussions.
- Cross-Disciplinary Insights: Collaboration across disciplines can drive innovation. Researchers in control systems, artificial intelligence, and application-specific fields should explore shared goals.