Electricity Transfer: Mechanisms and Future Trends
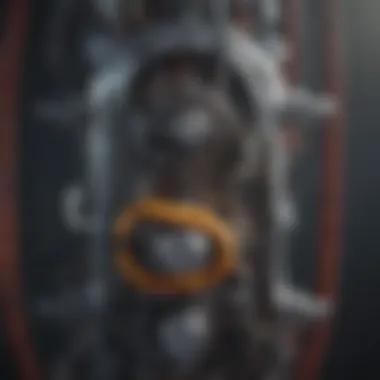
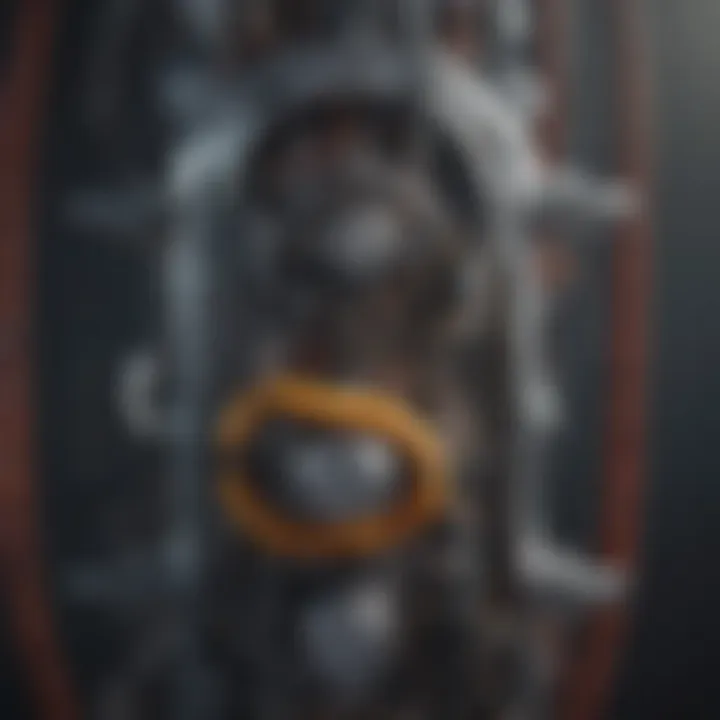
Intro
Electricity transfer is a critical aspect of modern society, underpinning various systems that power our daily lives. Understanding the mechanisms behind this transfer reveals the intricate frameworks that allow electric current to move efficiently from one point to another. From household appliances to advanced industrial systems, the methods of electricity transfer are diverse and complex.
In this article, we will explore the different ways electricity can be transferred, including both conventional methods and innovative technological advancements. We will analyze the implications of these methods in terms of efficiency and sustainability. Additionally, we will examine future directions in electricity transfer, assessing ongoing research and emerging trends that could reshape the field.
This exploration will be particularly useful for students, researchers, educators, and professionals in the energy sector. By articulating the fundamental principles underlying electricity transfer, this guide aims to enhance comprehension and provoke thought about the future of electric energy systems.
Methodologies
Investigating the mechanisms of electricity transfer demands a meticulously designed approach. By employing various research techniques, scholars and practitioners can assess the efficiency and effectiveness of different methods.
Description of Research Techniques
Research in electricity transfer often incorporates both experimental and theoretical methodologies. Experimental techniques include laboratory experiments that measure the performance of specific transfer methods. In contrast, theoretical techniques involve modeling and simulation to understand the underlying principles at play.
Tools and Technologies Used
The tools involved in researching electricity transfer vary widely. They often include:
- Specialized software for modeling electric currents and fields, such as COMSOL Multiphysics or ANSYS Electromagnetics.
- Laboratory equipment for testing electrical components like oscilloscopes and multimeters to measure voltage, current, and resistance.
- Data collection and analysis tools to interpret experiments and validate findings.
"Understanding the mechanisms behind electricity transfer is essential not only for improving efficiency but also for fostering sustainable energy practices."
By implementing these methodologies, researchers can generate valuable insights into electricity transfer mechanisms, which directly influences applications in technology and sustainability.
Discussion
Comparison with Previous Research
When analyzing recent developments in electricity transfer, it is important to juxtapose them with previous research. Historically, electricity transfer focused largely on traditional conductive materials like copper and aluminum. Recent innovations, however, examine alternative materials like graphene, which promises enhanced performance. The shift towards new materials signifies a pivotal moment in the field, addressing limitations of past methodologies.
Theoretical Implications
Theoretical advancements in the understanding of electricity transfer have profound implications. New theories propose more efficient ways to simplify transmission processes while minimizing losses. Additionally, these advancements assist in addressing modern energy challenges, such as the need for enhanced grid stability and integration of renewable energy sources.
With the knowledge gained from past research and current innovations, the future of electricity transfer looks promising. Researchers will continue to explore more efficient and sustainable methods, paving the way for technological advancements that benefit society.
Understanding Electricity Transfer
Electricity transfer is a foundational component of modern technology and infrastructure. It serves as the lifeblood of numerous systems, from residential wiring to vast industrial applications. Recognizing the mechanisms behind electricity transfer is essential for anyone involved in engineering, energy management, or technology innovation. As energy demands continue to rise, understanding these principles becomes more vital.
Electricity transfer encompasses various processes, from generation to end-use. Each step involves intricate physical laws and technologies that ensure energy is conveyed effectively and safely. Enhancing this understanding can lead to improvements in energy efficiency, safety, and sustainability, which are critical as society aims for greener solutions.
Definition of Electricity Transfer
Electricity transfer refers to the movement of electrical energy from one point to another. This movement can occur through different mediums and methods, primarily via conductors. The process involves several key elements, including voltage, current, and resistance. Voltage represents the electrical potential difference, current is the flow of electric charge, and resistance is the opposition to the flow of that charge.
Electricity transfer is not limited to a single form. It manifests as both alternating current (AC) and direct current (DC), each having unique characteristics and applications. AC is commonly used in power grids due to its ability to be transmitted over long distances with minimal energy loss. In contrast, DC is prominent in battery-powered devices.
Significance in Modern Society
The significance of electricity transfer in modern life is profound. From the simple act of turning on a light to operating complex industrial machines, electricity is integral. The dependance on reliable electricity transfer systems ensures that homes are powered, businesses operate efficiently, and essential services, such as healthcare, function uninterrupted.
- Economic Growth: Efficient electricity transfer plays a crucial role in economic development. Industries rely heavily on power for manufacturing and services. When electricity is delivered effectively, productivity increases.
- Technological Advancements: Innovations in communication and information technology also depend on robust electricity transfer mechanisms. Fields like telecommunications cannot function without reliable electricity.
- Energy Sustainability: Understanding and improving electricity transfer can contribute to sustainability goals. Renewable energy sources, such as solar and wind, require efficient transfer systems to make a meaningful impact on energy supply.
In summary, electricity transfer is a multifaceted topic that underpins much of contemporary life. As energy demands evolve, so does the pressing need to rethink and optimize how electricity is transferred across various platforms.
Mechanisms of Electricity Transfer
The mechanisms of electricity transfer form the core of electrical engineering and technology. They define how electrical energy moves from one point to another, allowing for a wide array of applications across industries and daily life. Understanding these mechanisms is essential for improving efficiency, enhancing performance, and driving innovation in energy systems.
Conductors vs. Insulators
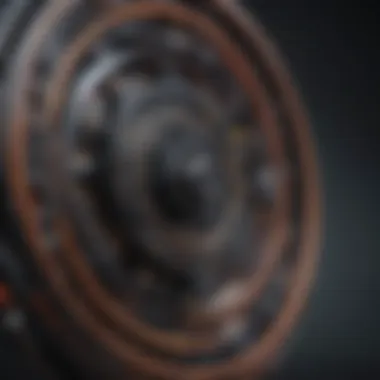
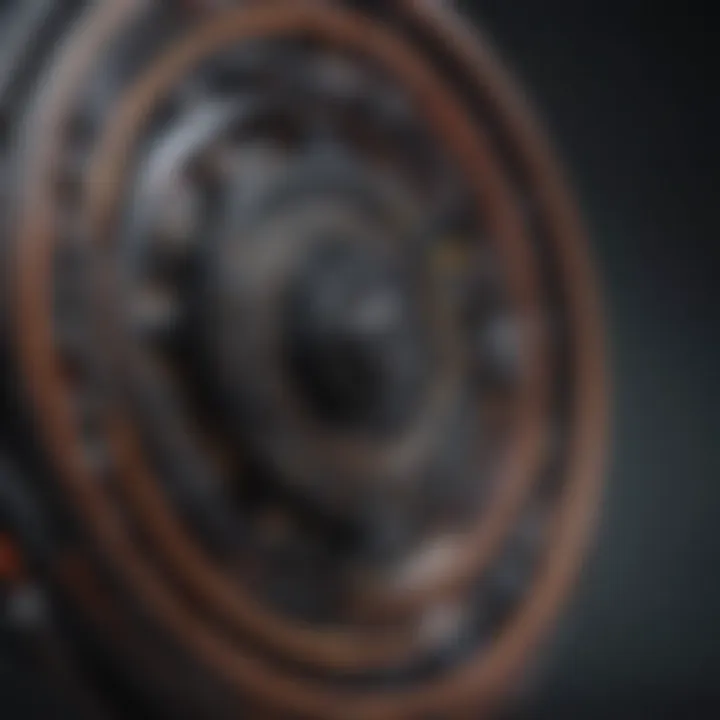
In the realm of electricity transfer, the distinction between conductors and insulators is fundamental. Conductors are materials that allow the easy flow of electricity due to their atomic structure. Metals like copper and aluminum are prime examples, offering low resistance and high conductivity. These materials are crucial in wiring and transmission systems.
On the other hand, insulators are materials that hinder the flow of electrical current. Substances such as rubber, glass, and plastics are common insulators. Their primary purpose is to protect and separate conductive materials, preventing unwanted current leakage which could lead to hazards.
In practical applications, the interplay between conductors and insulators is vital. For example, power lines use conductive metals encased in insulative materials to transmit electricity safely over long distances. This synergy ensures both efficiency and safety in electricity transfer processes.
Electromagnetic Induction
Electromagnetic induction is another key mechanism in the transfer of electricity. This phenomenon occurs when a change in magnetic field within a closed loop induces an electric current. The principle was first discovered by Michael Faraday and has since been foundational to many technologies.
One of the most significant applications of electromagnetic induction is in transformers, which are essential for altering voltage levels in power transmission. By adjusting the voltage, electricity can be efficiently transmitted over vast distances, minimizing energy loss. Moreover, induction is utilized in electric generators where mechanical energy is converted into electrical energy, a process integral to the functioning of modern power plants.
Transmission Lines
Transmission lines represent the physical pathways through which electrical energy travels from generating stations to consumers. These lines are designed to handle high voltages necessary for efficient power distribution. The configuration and materials used in transmission lines deeply influence their performance.
Key factors in transmission line design include:
- Line Voltage: Higher voltages reduce current, which minimizes energy loss during transfer.
- Conductor Material: Choices like aluminum or copper impact conductivity and overall efficiency.
- Line Length: Longer distances can introduce significant energy losses unless properly managed.
Modern transmission systems integrate sophisticated technologies, such as smart grids, to monitor and optimize electricity flow. These systems can adjust to real-time demands, enhancing the reliability and efficiency of electricity transfer.
"Transmission lines are the veins of electric power systems, crucial for maintaining flow and connectivity."
In summary, the mechanisms of electricity transfer provide the backbone of our electrical infrastructure. Understanding the characteristics of conductors and insulators, the role of electromagnetic induction, and the design considerations for transmission lines will allow for continued advancements and innovations in electricity delivery.
Types of Electricity Transfer
The concept of electricity transfer is central to various technologies that shape our daily lives. Understanding the different types of electricity transfer is essential, as each method has unique characteristics, implications, and applications. This section will explore three primary methods: Direct Current (DC), Alternating Current (AC), and Wireless Electricity Transfer. By examining these types, we will highlight their significance in improving efficiency and implementing sustainable energy solutions.
Direct Current () Transfer
Direct Current (DC) transfer is characterized by the unidirectional flow of electric charge. This means that electrons move in a single direction, usually from the negative terminal to the positive terminal. DC is widely used in batteries, which are vital for portable electronic devices. The primary advantage of DC is its ability to maintain a constant voltage, making it suitable for low voltage applications and electronic circuits.
In practical terms, DC transfer is common in devices such as mobile phones, laptops, and solar panels. Solar cells produce DC electricity, which can be stored in batteries or converted to AC for power grids. However, transmitting DC over long distances presents challenges, primarily due to energy losses in the conductors. Technologies like HVDC (High Voltage Direct Current) systems have emerged as effective solutions, minimizing losses and facilitating efficient transmission over vast distances.
Alternating Current (AC) Transfer
Alternating Current (AC) transfer involves the periodic reversal of the direction of electron flow. AC is the standard form of electricity supplied to homes and industries, and it operates at various voltage levels. The main benefit of AC is its ability to be easily transformed to different voltage levels using transformers. This property allows long distances transmission with significantly reduced energy losses.
AC is predominant in power generation and has been instrumental in the development of the electrical grid. Its versatility enables it to power a wide range of devices, from household appliances to large industrial machines. Additionally, AC motors are widely used in various applications due to their efficiency and robust performance. However, AC has complexities such as harmonics, which need careful management in electrical systems.
Wireless Electricity Transfer
Wireless electricity transfer is an emerging technology that allows electrical energy to be transmitted without physical wires. This method employs various techniques such as inductive coupling and resonant inductive coupling. Inductive coupling is largely used in applications like wireless charging pads for smartphones and electric toothbrushes.
Wireless transfer systems enhance convenience but also present unique challenges, including efficiency and range limitations. Currently, these systems are most effective in short distances. For example, electric vehicle charging stations are gradually adopting wireless technologies, representing a significant step towards cleaner energy transfer methods. The potential for expanding wireless transfer capabilities holds promise for future innovations in energy distribution and usage.
Understanding these types of electricity transfer equips us with knowledge essential for assessing their roles in both current technologies and future advancements.
In summary, the types of electricity transfer each serve distinct functions within the broader landscape of energy distribution. As technology progresses, continuous improvements in efficiency and applications are anticipated across all these methods.
Efficiency in Electricity Transfer
Efficiency in electricity transfer is a cornerstone of modern electrical systems. It refers to the ratio of useful power output to the total power input. High efficiency ensures that less energy is wasted, which can lead to lower operational costs and reduced environmental impact. Enhancing efficiency is essential for both existing and emerging technologies. The discussion will cover several aspects, including factors that affect efficiency and the innovative solutions currently available.
Factors Affecting Efficiency
Various factors can influence the efficiency of electricity transfer. These include:
- Conductor Material: Conductors like copper have lower resistance than aluminum, affecting how much energy is lost as heat.
- Distance: The greater the distance electricity travels, the more energy is lost due to resistance along the transmission lines.
- Voltage Levels: High-voltage transmission reduces current and, therefore, the energy loss associated with resistance, considerably improving efficiency.
- Temperature: Higher temperatures increase resistance, leading to more energy losses.
- Load Variability: Constant monitoring of load conditions and adapting the power supply can improve efficiency significantly.
Understanding these factors helps engineers design systems that optimize electricity transfer, reducing waste at every step.
Innovative Solutions for Enhanced Efficiency
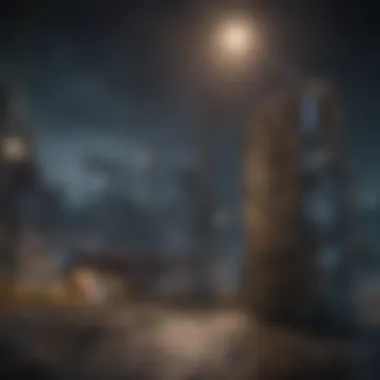
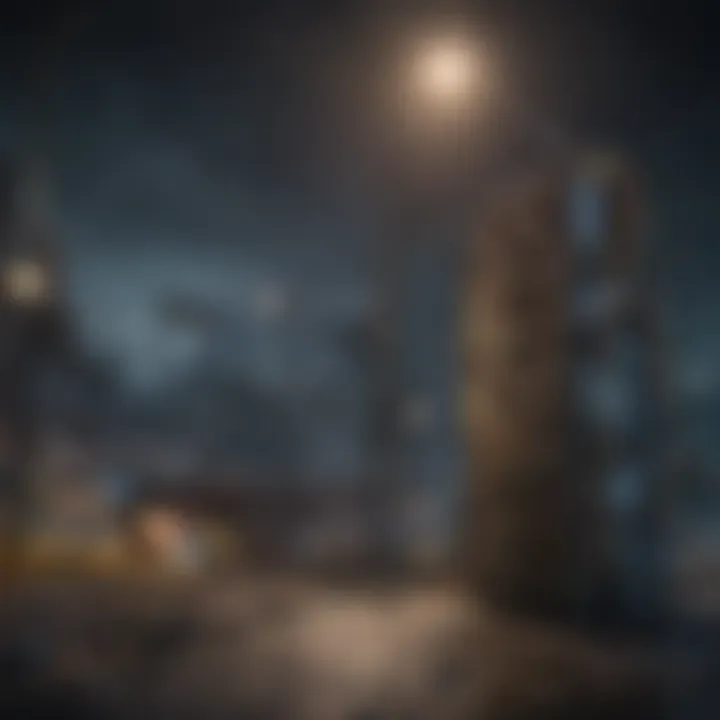
In recent years, several innovative solutions have emerged to boost efficiency in electricity transfer:
- Smart Grids: These advanced systems use digital technology to manage electricity demand more effectively, balancing supply and reducing losses.
- Superconducting Materials: Research on superconductors presents the potential to eliminate resistance entirely, making electricity transfer nearly perfect.
- High-Voltage Direct Current (HVDC): This technology improves long-distance electricity transmission, reducing losses compared to traditional AC methods.
- Distributed Energy Resources (DERs): Incorporating local energy sources like solar panels helps cut down transmission distances, thereby enhancing efficiency.
- Energy Storage Systems: Efficient batteries and storage technologies can stabilize supply and demand, leading to less waste during periods of low usage.
"An efficient electric system is not just beneficial but essential for sustainable development in today's world."
Implementing these solutions can significantly impact energy consumption practices, paving the way for a more sustainable future.
By focusing on efficiency, society can make strides toward more effective energy use, benefiting both the economy and the environment.
Applications of Electricity Transfer
The significance of electricity transfer extends far beyond mere power distribution. Its applications touch every aspect of modern life, cementing its role as a backbone for technological growth. Understanding the applications of electricity transfer not only illustrates its practical benefits but also highlights its vital role in fostering innovation across various sectors. From industry to home life, and through advancements in electric vehicles, the strategies for transferring electricity are critical to current and future developments.
Industrial Applications
In industrial settings, electricity transfer systems are integral for operational efficiency and productivity. Large manufacturing facilities utilize robust electrical systems to power machinery and equipment. These systems often involve complex networks of transmission lines and transformers that manage high voltage levels, ensuring a stable energy supply needed for production processes. For instance, factories leveraging automated assembly lines rely heavily on uninterrupted electricity transfer to maintain their workflows.
Moreover, industries are increasingly adopting smart grid technologies, which facilitate real-time monitoring and optimization of energy distribution. This can lead to significant cost savings and reduced downtime, which are crucial for maintaining competitive advantage in a fast-paced market. Important elements to consider include:
- Energy Efficiency: Reduced energy waste through optimized transfer systems.
- Real-Time Monitoring: Ability to address potential issues before they affect production.
- Sustainability Initiatives: Integrative systems that support renewable energy sources.
Residential Applications
In homes, electricity transfer is pivotal for day-to-day activities. From basic lighting and heating to powering sophisticated smart home devices, efficient electricity distribution contributes greatly to comfort and convenience. The rise of smart home technology further illuminates the importance of effective electricity transfer. Devices such as smart thermostats or automated lighting systems not only enhance user experience but also lead to better energy management.
Key aspects in residential applications include:
- Convenience and Control: Users can manage energy consumption from their smart devices.
- Cost Efficiency: Efficient transfer reduces electricity bills through better usage patterns.
- Safety Features: Modern systems incorporate safety measures to prevent electrical hazards.
Electric Vehicles
The emergence of electric vehicles (EVs) represents a transformative application of electricity transfer, promoting sustainable transport solutions. EV charging infrastructure, such as charging stations, relies on an intricate system of electricity transfer to ensure vehicles are powered efficiently.
Factors to consider in the context of electric vehicles include:
- Charging Speed: Fast charging options to reduce downtime for users.
- Renewable Integration: Utilizing solar or wind power to charge EVs, enhancing sustainability.
- Infrastructure Development: Expanding charging networks to support growing EV adoption is critical.
The shift toward electric vehicles not only represents a change in transportation but also necessitates an evolution in how electricity transfer systems are structured.
Through all these applications, electricity transfer not only empowers existing technologies but also lays the groundwork for future innovations. Understanding and enhancing these applications is crucial to advancing society's energy landscape.
Future Trends in Electricity Transfer
The future of electricity transfer is not merely about continuing existing practices; it is about innovating and adapting to the evolving demands of modern society. Future trends in this field suggest profound impacts on efficiency, sustainability, and technology. By focusing on advancements, we see defined paths toward smarter systems and greener solutions. Key aspects include the integration of smart grid technologies and a greater emphasis on transitioning to sustainable energy sources. The benefits of these trends are multifold, including reduced energy costs, enhanced reliability, and lower environmental footprints. As we look forward, it is critical to consider both the opportunities and challenges these trends present.
Smart Grid Technologies
Smart grid technologies represent a significant leap in managing electricity transfer. This approach leverages digital communication technologies to enhance the efficiency and reliability of the electric grid. Through real-time monitoring, these systems can manage electricity consumption better, providing real-time data to both consumers and utilities.
Some key features of smart grids are:
- Demand response allows consumers to adjust their energy usage during peak periods, reducing strain on the grid.
- Distributed energy resources such as solar panels and wind turbines can be easily integrated, allowing for a more decentralized energy production model.
- Advanced metering infrastructure provides detailed data to consumers, enabling them to make informed decisions about their energy use.
"The implementation of smart grids is expected to reduce operational costs considerably while enhancing service reliability."
This transition helps in moving away from conventional, large-scale energy production to more localized and renewable sources, thus promoting sustainability and energy independence.
Sustainable Energy Solutions
Sustainable energy solutions are increasingly crucial in addressing the global energy crisis. With the need for cleaner energy sources, electricity transfer mechanisms must adapt to support these alternatives. Renewable resources like solar, wind, and hydroelectric energy are becoming more significant.
Key components of sustainable energy solutions include:
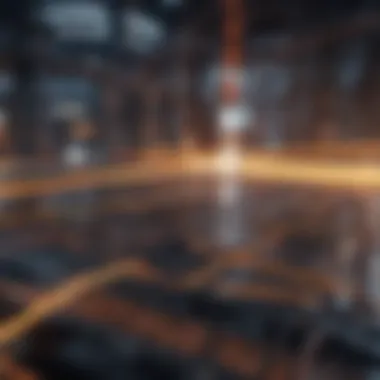
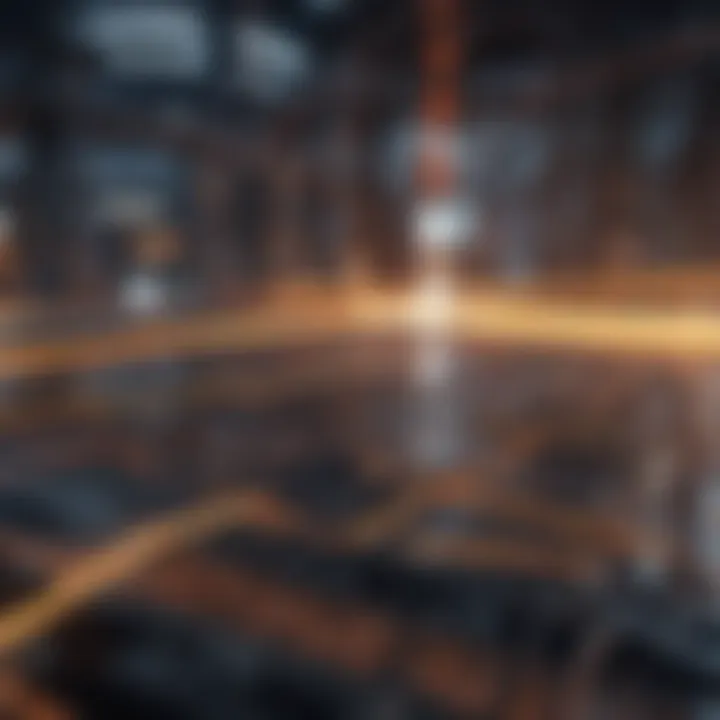
- Energy Storage Systems that ensure a consistent energy supply even when production is low.
- Electric Vehicles provide a dual benefit; they can act as energy consumers while also serving as storage units during peak demand.
- Microgrids that can operate independently from the national grid, enhancing local resilience and sustainability.
The ongoing shift toward renewable energy sources will not only lower greenhouse gas emissions but also encourage innovation in electricity transfer mechanisms. Solutions focusing on sustainability are vital for achieving long-term energy security and environmental preservation.
Safety Considerations in Electricity Transfer
Safety considerations are critical in the world of electricity transfer. As technology continues to evolve, the risks associated with electricity also increase. Understanding these risks helps to prevent accidents and injuries. The importance of this topic cannot be understated, as it encompasses both the safety of individuals and the effectiveness of electrical systems.
Understanding Electrical Hazards
Electrical hazards can manifest in several forms, including electrical shocks, burns, and explosions. These hazards can occur due to faulty wiring, improper use of electrical equipment, or failure to follow safety protocols. Some common types of electrical hazards include:
- Direct contact: This occurs when a person touches an energized conductor. It can lead to severe injuries or fatalities.
- Overcurrent and short circuits: These situations can cause overheating of electrical components, leading to fires.
- Electromagnetic fields (EMF): Prolonged exposure to high EMF levels may result in health concerns, although research is ongoing.
It's essential to recognize the signs of potential hazards and implement measures to mitigate them. Training and awareness programs can enhance the ability to identify risks and react appropriately during emergencies.
Safety Standards and Regulations
Safety standards and regulations play a vital role in electricity transfer. Organizations like the National Fire Protection Association (NFPA), International Electrotechnical Commission (IEC), and Occupational Safety and Health Administration (OSHA) establish guidelines that ensure electrical systems are designed and operated safely.
Key regulations include:
- National Electrical Code (NEC): This sets the foundation for safe electrical installations in residential and commercial settings.
- Regulatory Compliance: Electrical professionals must ensure compliance with local, state, and federal laws.
- Routine Safety Inspections: Regular assessments can identify potential hazards, ensuring that electrical systems remain safe and effective.
The implementation of stringent safety standards leads not only to the protection of life but also to the prevention of costly accidents and system failures.
"The only way to achieve safety in electrical transfer is through systemic adherence to established safety standards."
By understanding and following these safety considerations, individuals and organizations can significantly reduce the risks associated with electricity transfer. Fostering a culture of safety within an organization encourages continuous improvement in electrical safety practices, benefiting both current and future operations.
Global Impact of Electricity Transfer
Electricity transfer plays a critical role in shaping economies and environments across the globe. This topic reflects not only the methods of delivering electrical energy but also the far-reaching consequences that result from how this transfer is managed. Multiple dimensions need attention, notably the economic implications and environmental consequences.
Economic Implications
The economic landscape is heavily influenced by electricity transfer. Efficient electricity transfer systems directly correlate with enhanced productivity in both industrial and residential sectors. Investment in modern infrastructures, such as smart grids, facilitates greater reliability and reduces costs associated with electricity distribution.
- Job Creation: The growth in energy sectors, especially renewables, creates numerous job opportunities. Positions in maintenance, engineering, and technology development expand as demand for efficient systems rises.
- Cost Reduction: Improved methods of electricity transfer enable lower operational costs. For instance, efficient solar panel systems not only lower electricity bills but also provide economic incentives through governmental subsidies.
- Regional Development: Regions with robust electricity transfer systems are more attractive for businesses. This direct relation enhances economic development and promotes equitable growth across different areas.
Economic growth is often contingent upon effective electricity systems. Without it, communities may struggle to attract investments.
Environmental Considerations
The environmental aspects of electricity transfer cannot be overlooked. The processes involved in generating and distributing electricity have profound repercussions on ecological systems.
- Carbon Footprint: The transfer of electricity from fossil fuel-based sources contributes to significant greenhouse gas emissions. Transitioning to renewable energy sources like wind, solar, and geothermal significantly reduces the carbon footprint associated with electricity generation and transfer.
- Resource Management: Efficient transfer systems minimize waste during electricity distribution. Implementing technologies that enhance the grid's adaptability can lead to reduced energy loss, particularly during peak demand times.
- Sustainability Practices: Initiatives aimed at improving electricity transfer must align with sustainable practices. This includes investing in technologies that harness electricity from cleaner sources and developing strategies to phase out reliance on environmentally damaging practices.
In summary, the global impact of electricity transfer is multi-faceted. From strengthening economies through job creation and efficiency to addressing environmental challenges, its relevance cannot be overstated. It is essential to promote continuous innovation in this field to achieve a balanced approach that serves both economic growth and ecological integrity.
The End
The conclusion serves a vital role in encapsulating the significance and insights of the entire discussion regarding electricity transfer. It aims to reinforce the essential concepts presented throughout the article, allowing readers to walk away with a clear understanding of the subject. Addressing the key themes—from the mechanisms that govern electricity transfer to its extensive applications and the future directions—creates a cohesive narrative.
First, a summary of major points establishes context. Highlighting the differences between Direct Current and Alternating Current, and the importance of electromagnetic induction underlines foundational concepts. Furthermore, recognizing the role of efficiency in various applications, such as in electric vehicles and residential systems, showcases both practical implications and technological advancements. This creates a sense of continuity and importance as readers consider how these elements interact.
Second, discussing future trends in electricity transfer emphasizes the dynamic nature of the field. The emergence of smart grid technologies and sustainable energy solutions points toward an evolving landscape that can enhance efficiency and adaptability. Not only do these advancements affect the economic infrastructure, but they also hold implications for environmental sustainability.
Ultimately, the culmination of this article shall inspire further inquiry. With technological innovation accelerating in ways previously thought impossible, the discussion does not end here. Exploring future research directions can uncover new opportunities for advancements in electricity transfer systems.
"Understanding electricity transfer not only is integral for engineers and scientists but also transforms how society engages with energy resources."
Summary of Key Points
- Basics of Electricity Transfer: Understanding key mechanisms like conductors, insulators, and electromagnetic induction.
- Types of Transfer: Differentiation between Direct Current and Alternating Current, as well as wireless technologies.
- Applications: Use in industries, homes, and electric vehicles illustrates the breadth of impact.
- Efficiency: Acknowledging factors influencing efficiency and new technologies that improve it.
- Innovative Solutions: Future developments like smart grids and sustainable practices keep the discussion open for growth.
Future Research Directions
- Smart Grid Developments: Investigating how emerging technologies can improve reliability and efficiency.
- Sustainable Energy: Research into integrating renewable sources into existing electricity transfer systems.
- Materials Science: Exploring new conductive materials that can enhance efficiency and reduce losses during electricity transfer.
- Consumer Access: Studying how technology can democratize energy access, especially in underserved communities.
Addressing these focal points not only helps to solidifying the understanding of electricity transfer but also encourages the pursuit of advancements in the field. By emphasizing these directions, the hope is to inspire future scholarship and innovation.