Dynamic Light Scattering of Nanoparticles: Insights and Methods

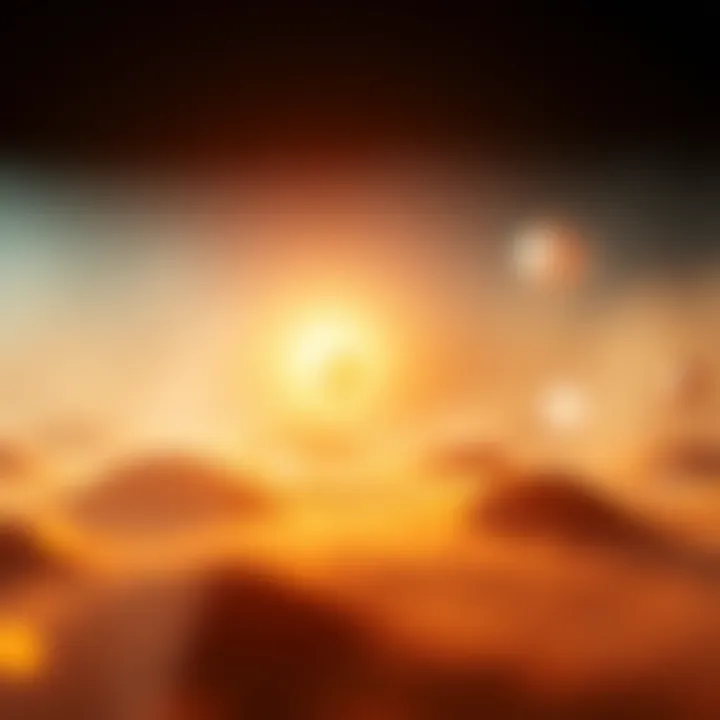
Intro
In the evolving world of nanotechnology, understanding the behavior and characteristics of nanoparticles is crucial. Among the various methods utilized for nanoparticle analysis, Dynamic Light Scattering (DLS) holds a significant place due to its effectiveness in providing insights into particle size distribution and dynamics. The beauty of DLS lies in its simplicity and robustness; it delivers data that is invaluable across fields including materials science, biomedicine, and environmental studies. This technique has changed the paradigms of how we can not only observe but also harness the properties of nanoparticles.
The forthcoming sections will delve into the methodologies that enable DLS as a key player in nanoparticle characterization, explore its multifaceted applications, and discuss the inherent challenges that come with it. From innovation to practical applications, the full spectrum of DLS will be laid out, offering readers a comprehensive perspective on this pivotal technique.
Methodologies
Dynamic Light Scattering employs various research techniques to measure the intensity of scattered light from particles that are in constant motion. Essentially, this motion arises from Brownian motion, where particles are subjected to random collisions in a fluid medium, leading to fluctuations in scattered light intensity.
Description of Research Techniques
There are a couple of fundamental approaches to conducting DLS studies. The most prevalent method involves using a monochromatic light source, typically a laser, which illuminates the sample. As the particles move, the scattering pattern changes, allowing for a calculation of their size based on the speed of these movements.
Another noteworthy technique is the correlation function, which analyzes how the intensity fluctuations of scattered light correlate over time. By applying the principles of autocorrelation, researchers can deduce the diffusion coefficients of the particles, translating them into particle size distributions. This correlation can be thought of as eavesdropping on the dance of particles within a liquid, capturing the rhythm of their movements.
Tools and Technologies Used
In today's high-tech landscape, the tools that facilitate DLS have become more sophisticated. Instruments generally include:
- Laser Diffraction Systems: These systems are integral for generating the light source necessary for scattering measurements.
- Photodetectors: These devices capture the intensity of light scattered from the sample, crucial for interpreting the data.
- Computational Software: Advanced algorithms analyze the scattered data, drawing out information related to size distribution and even the zeta potential of nanoparticles.
Discussion
Comparison with Previous Research
Over the years, DLS has evolved, paralleling advancements in both technology and methodology. Previous studies relied heavily on manual calculations and simpler instrumentation, often resulting in lower accuracy and higher incidences of error. The introduction of digital processing techniques has dramatically transformed this landscape. Today, DLS can yield sizes down to the nanoscale with more precision and reliability than ever before.
Theoretical Implications
From a theoretical standpoint, the implications of DLS extend far beyond simple measurement; they pave the way for deeper discussions about particle behavior at the nanoscale. As the science evolves, DLS promises not only insight into sizes but also informs stability, aggregation, and interactions of nanoparticles. Such knowledge can drastically affect applications across drug delivery systems, where the behavior of nanoparticles must be understood to optimize therapeutic outcomes.
"The dynamics of light scattering provide a window into the unseen world of nanoparticles, allowing scientists to unravel mysteries that hold the key to future technological breakthroughs."
In sum, DLS is not just a technique—it's a bridge between understanding and application in a field that is as intricate as it is revolutionary. With ongoing research and development, the possibilities for advancements in DLS continue to expand, offering new ways to manipulate and utilize nanoparticles for practical purposes.
Prolusion to Nanoparticles and DLS
Nanoparticles have grown as a key player in various scientific fields due to their unique properties that differ significantly from their larger counterparts. With sizes ranging from 1 to 100 nanometers, these particles exhibit remarkable behaviors influenced by their surface area, shape, and quantum effects. Understanding nanomaterials is essential in pushing the frontiers of engineering, medicine, and environmental science.
One prominent method for studying nanoparticles is Dynamic Light Scattering (DLS). DLS allows researchers to determine the size distribution and behavior of nanoparticles in solution with high precision. Its significance lies not only in measuring particle sizes but also in exploring their interactions, stability, and efficacy in various applications.
In this article, we will explore the fundamentals of nanoparticles and the DLS technique. We will uncover the way DLS operates, the equipment necessary for effective measurement, and the implications of those measurements in a practical context.
Key benefits of understanding DLS and nanoparticles include:
- Insight into how nanoscale materials can be used in technology, especially in drug delivery systems.
- Better decision-making in the design and development of nanomaterials for specific applications.
- Awareness of challenges that DLS faces, such as issues with aggregation and sensitivity in detection.
In addressing these topics, we aim to provide clarity and critical knowledge that will help students, researchers, educators, and professionals navigate the intricate landscape of nanomaterials and DLS.
Understanding Nanoparticles
At the heart of nanotechnology lies nanoparticles, tiny elements that can change the rules of the game in diverse applications—from electronics to pharmaceuticals. These particles can be made from metals, ceramics, or even polymers, and their characteristics can be tailored by modifying their size, shape, and chemical composition.
The small size of nanoparticles leads to a high surface area-to-volume ratio which grants them unique optical, electronic, and magnetic properties. For example, gold nanoparticles exhibit a vivid red hue due to surface plasmon resonance, making them ideal for applications in biomedical imaging or targeted therapy. Understanding these properties opens doors to innovative uses, such as targeted drug delivery where nanoparticles can be designed to release their therapeutic load only in specific cells, minimizing side effects.
In the realm of materials science, controlled synthesis of nanoparticles allows for the development of stronger, lighter, and more efficient materials for construction, aerospace, and many other fields.
Overview of Dynamic Light Scattering
Dynamic Light Scattering (DLS) is a powerful tool in the characterization of nanoparticles, leveraging the principles of light scattering to deliver rapid and accurate size measurements. The principle behind DLS is quite straightforward: when a laser beam hits a suspension of nanoparticles, the particles scatter the light in all directions. As they move, their positions fluctuate, leading to variations in the intensity of scattered light over time. By analyzing these fluctuations, scientists can extract particle size information.
The beauty of DLS lies in its ability to measure nanoparticles in complex suspensions without the need for extensive sample preparation. This method can provide crucial insights regarding the stability and behavior of nanoparticles in different environments. It plays a vital role across diverse fields, including pharmaceutical formulation, diagnostics, and material sciences.
Key Points of DLS:
- Non-invasive technique: Preserves sample integrity while obtaining size data.
- Fast real-time analysis: Results can be generated in minutes.
- Quantitative data: Provides size distributions, allowing for a comprehensive understanding of sample characteristics.
"The ability of DLS to give a rapid size analysis of nanoparticles makes it invaluable in research and quality control in industries.
In summary, as we delve deeper into the principles of DLS and its applications, it becomes apparent that understanding nanoparticles and this measurement technique is not just academically interesting; it truly holds substantial practical implications across numerous industries.
Principles of DLS
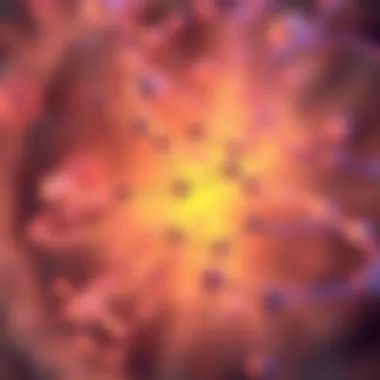
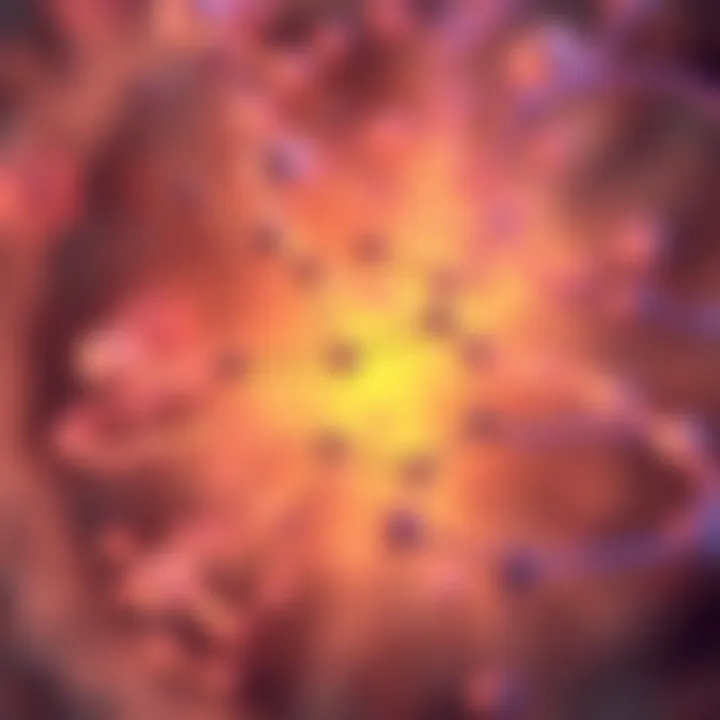
Dynamic Light Scattering (DLS) is often considered the backbone of nanoparticle characterization techniques. Understanding the principles of DLS is paramount, as it intricately informs the methodology and ultimately impacts the application of the data collected. The core of DLS revolves around how light interacts with particles in suspension, providing insights into their size and distribution. This foundational knowledge translates into various fields, including materials science and biomedicine, where knowing the specifics of particle behavior can make a significant difference.
Light Scattering Phenomena
Light scattering is the cornerstone of DLS. When a laser light hits a nanoparticle, the light is scattered in various directions due to the particle's presence. The behavior of these scattered light waves, especially how they fluctuate over time, gives us critical data about the particle's size. For instance, if there is a quick change in the intensity of the scattered light, it suggests that the particles are small and moving about rapidly.
It's fascinating to note that larger particles scatter light less intensely but tend to drift slower, influencing the autocorrelation function used in DLS analysis. This relationship is an essential aspect that researchers must consider: larger and smaller particles do not only behave differently in terms of scattering but also have varied impacts on the medium they occupy. Thus, understanding this phenomenon isn't just about measurement but involves a deeper comprehension of nanoparticle interactions within suspensions, reshaping experimental designs accordingly.
Particle Size and Diffusion
The size and mobility of nanoparticles play a crucial role in DLS measurements. At its essence, the capacity to identify size distributions is tied to the particles' Brownian motion—random motion arising from collisions with solvent molecules. When discussing particle size in the context of DLS, one must remember that size can imply a range of physical characteristics, including surface area, reactivity, and even biological compatibility.
In practical terms, DLS can provide an average particle size, often represented as the Z-average. However, the more the particles vary in size, the less precise this average becomes. This aspect highlights the necessity of understanding how diffusion rates correlate with particle size: smaller particles diffuse more rapidly than larger ones. This relationship not only aids in particle characterization but also offers insight into stability and behavior in various applications, particularly when it comes to formulation and delivery in biomedicine.
Mathematical Models in DLS
Mathematics plays a significant role in translating light scattering data into understandable information about nanoparticles. Several models exist to interpret DLS data effectively. The simplest starts with the Stokes-Einstein equation, which relates the diffusion coefficient of particles to their size:
Where:
- D is the diffusion coefficient,
- k is the Boltzmann constant,
- T is the temperature,
- θ is the viscosity of the solvent,
- r is the radius of the particle.
This equation is foundational, as it connects temperature and viscosity directly with particle size. Advanced models, like the cumulant analysis, offer more nuanced insights by fitting the correlation functions obtained from DLS experiments, allowing researchers to decipher not just average sizes but also size distributions more accurately.
In summary, grasping the principles behind DLS is more than just a technical requirement; it’s about realizing how these principles integrate with experimental practices, leading to significant advancements in scientific research and application.
Understanding these principles can enhance a researcher’s capacity to interpret data critically, thereby expanding the capability to innovate within various scientific fields.
Instrumentation and Techniques
Dynamic Light Scattering (DLS) stands as a crucial methodology within nanoparticle analysis, serving both academic and industrial sectors. A solid grasp of the instrumentation and techniques involved in DLS feeds directly into its effectiveness, accuracy, and reliability. Understanding what makes up the equipment and how it is employed is more than just trivial knowledge; it’s akin to knowing the ropes of a trade. The choice of instruments and the methods of calibration directly affect the quality of the resulting data, which in turn influences any conclusions drawn from the measurements.
DLS Equipment Components
A DLS setup can seem like a simple arrangement of parts, but each component plays a pivotal role in ensuring accurate light scattering measurements. Let's go over the primary components:
- Laser Source: The heartbeat of any DLS equipment. The laser provides the coherent light that illuminates the nanoparticle suspension, and typically, a solid-state laser with a wavelength around 633 nm is used.
- Sample Cell: This is where the magic happens. The sample cell, also known as a cuvette, must be transparent to the laser light. It’s often made of quartz to avoid any scattering errors.
- Detector: This device captures the scattered light, allowing scientists to measure the intensity fluctuations over time. It can be a simple photomultiplier tube or a more sophisticated avalanche photodiode, depending on the sensitivity required.
- Autocorrelator: A vital piece that analyzes the time-dependent intensity fluctuations of the scattered light. This is what ultimately gives the particle size data.
- Data Processing Software: No analysis can be complete without robust software that processes, interprets and visualizes the data collected. The algorithms running in the background are the brains of the operation, converting light intensity data into size distributions.
Overall, the harmony of these components is what makes DLS a preferred method for studying nanoparticles.
Calibration Procedures
Calibration is not just a regulatory exercise; it ensures that the DLS system produces reliable and reproducible results. When you’re diving into the world of nanoscale measurements, even the slightest drift can lead to significant discrepancies. Here’s an overview of the calibration process:
- Preparation of Standard Solutions: For accurate calibration, one generally uses standard solutions of particles with known sizes—like polystyrene or silica latex beads.
- Initial Setup Checks: Before delving into the measurements, it’s vital to ensure all parts of the equipment are functioning correctly. This includes alignment of the laser, ensuring the detector is receiving light, and checking for any background noise.
- Measurement Series: The redistribution of scattered light is measured in different configurations to obtain a variety of readings, which establishes a baseline. The average over multiple readings will improve the reliability.
- Adjustments to Parameters: It might be necessary to tweak settings such as the acquisition time, and the focus of the laser beam to obtain the best results.
- Ongoing Calibration: Regular checks with fresh standard solutions should be part of any diligent scientific routine. Keeping tabs on calibration helps maintain the reliability of long-term measurements.
Effective calibration not only mitigates errors but also enhances confidence in the data interpretations made over the course of research or product development.
By recognizing the importance of these components and the calibration process, researchers can glean significant insights when employing DLS techniques for their nanoparticle studies.
Sample Preparation for DLS
Sample preparation is a cornerstone in ensuring the reliability and accuracy of Dynamic Light Scattering (DLS) results. When it comes to characterizing nanoparticles, taking a meticulous approach during preparation can dramatically influence the quality of the generated data. If samples are not prepared correctly, even the best DLS instruments may yield misleading results. Thus, understanding the principles of sample preparation is not just beneficial; it’s essential for drawing trustworthy conclusions in research and applications.
Choosing Suitable Solvents
The choice of solvent plays a crucial role in DLS. The solvent must not only be compatible with the nanoparticles but should also maintain the required stability of the suspension. Factors such as molecular weight, dielectric constant, and boiling point can inform the decision. For example:
- Water is often the go-to solvent due to its universal accessibility and compatibility with many materials, especially for biological applications.
- Organic solvents like ethanol or acetone might be preferable when working with hydrophobic nanoparticles. They can help reduce aggregation or unwanted interactions that may arise in aqueous environments.
Considerations should also include the viscosity of the solvent. A solvent that’s too viscous can slow down the diffusion of particles, affecting the scattering profile. Hence, a careful balance must be achieved.
Ensuring Homogeneity in Samples
Once the suitable solvent is chosen, the next challenge is achieving homogeneity within the sample. This is vital because DLS works by measuring the fluctuations in intensity of scattered light caused by particles moving in the medium. Non-homogeneous samples can result in varied scattering patterns that lead to inaccurate particle size distributions. Some effective strategies include:
- Sonication: Applying ultrasonic waves can help disperse aggregates and ensure a uniform distribution. However, careful attention is needed to avoid excessive energy input, which can alter particle characteristics.
- Gentle stirring or mixing: This can also promote uniformity without compromising the stability of the nanoparticles. But avoid harsh mixing methods that produce shear forces, as these can lead to either particle aggregation or, conversely, fragmentation.
"In DLS, preparation is as critical as the measurement. If one doesn’t get it right in the lab, the data might lead you down the wrong path entirely."
The integrity of the sample should be continuously monitored. Regularly checking for signs of sedimentation or aggregation helps maintain a representative state for analysis. Proper record-keeping during sample preparation phases contributes to the overall scientific rigor of the project. By prioritizing suitable solvents and ensuring uniformity, researchers can enhance the reliability of DLS measurements significantly.
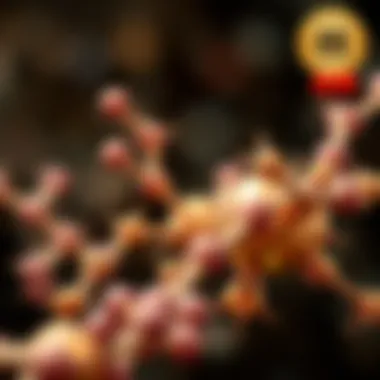
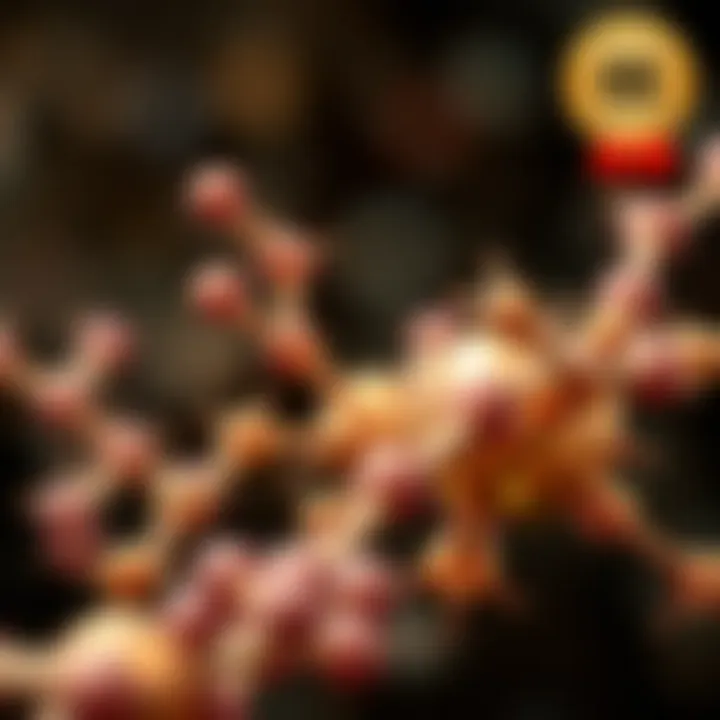
Data Analysis in DLS
Data analysis plays a pivotal role in the accuracy and effectiveness of Dynamic Light Scattering (DLS) techniques when it comes to characterizing nanoparticles. Without a thorough understanding of data analysis, researchers may miss essential insights that can influence their findings and overall project direction. A well-executed analysis ensures that the data derived from DLS experiments is reliable and can inform future research or applications.
Understanding Correlation Functions
Correlation functions serve as the backbone of DLS data analysis. These mathematical functions represent the relationship between the scattered light fluctuations over time. In simpler terms, they're like a clear window into how particles behave in a solution. By analyzing these fluctuations, researchers can gather clues about the size, distribution, and movement of nanoparticles.
DLS technology measures the distance and time between light scattering events, creating a dynamic picture of how particles change over time. Typically, the measured autocorrelation function shows the decay of light intensity over time and can be mathematically described as:
The function's decay time gives insight into particle size; faster decay correlates to smaller particles while slower decay indicates larger ones.
"Correlation functions give us a lens through which we can observe the dance of nanoparticles in motion. Without that lens, the picture remains hazy at best."
Moreover, the outcomes from correlation functions are not merely tentative numbers. They allow researchers to assess sample homogeneity, which is crucial in ensuring that the data is representative of the sample as a whole. Understanding how to interpret correlation functions is key for anyone venturing into this analysis realm.
Interpreting Size Distributions
Once correlation functions are established, interpreting size distributions is the next critical step. Size distribution interpretations can present a comprehensive overview of the particle population in a sample, often displayed in histograms or graphs.
In the realm of DLS, size distributions are typically modeled following a few common algorithms, such as: Daniele's method
- Non-negative least squares (NNLS)
- CONTIN algorithm
- Regularization methods
These algorithms convert the scattering data into meaningful sizes. A particulary valuable method is the CONTIN algorithm, which creates probability distribution of sizes and enables researchers to identify a range of particle sizes within the sample—something that's vital in applications like drug delivery or environmental monitoring.
While interpreting size distributions can seem straightforward, complications may arise. For instance, polydispersity—when particles vary in size—can skew results if not properly accounted for. Hence, robustness in analysis, paired with a keen understanding of these methods, is essential to draw reliable conclusions.
Finally, accurate size distribution analysis provides an edge when it comes to correlating particle characteristics with their behaviors and interactions in various scenarios, be it drug localization or material properties in advanced technologies.
Thus, data analysis not just enhances understanding—it's absolutely fundamental in steering research towards successful outcomes.
Applications of DLS
Dynamic Light Scattering (DLS) serves as a vital tool in various fields, particularly for nanoparticle characterization. Understanding its applications not only aids in advancing technology but also paves the way for innovative solutions to contemporary challenges. This section delves into key applications and highlights their significance in nanotechnology, biomedicine, and environmental sciences.
Nanoparticle Characterization
In the realm of nanotechnology, the characterization of nanoparticles is crucial. DLS offers a non-invasive and rapid approach to measuring particle size distribution, a pivotal factor in defining the efficacy of nanoparticles in applications ranging from drug delivery to materials synthesis.
Some specific advantages of DLS in nanoparticle characterization include:
- Quick Results: Unlike some traditional methods, DLS can provide rapid assessments, saving time in research phases.
- Minimal Sample Preparation: Samples can be analyzed in a straightforward manner, preserving their natural state, which is essential for accurate results.
- Broad Size Range Detection: DLS can effectively characterize a wide range of sizes, typically from a few nanometers up to several micrometers, covering most nanoparticles of interest.
Moreover, DLS can be used to monitor changes in nanoparticle size over time, enabling researchers to track aggregation or disaggregation phenomena in real-time. This dynamic observation helps delineate the stability and behavior of nanoparticles in different environments, which is vital for practical applications.
Biomedicine and Drug Delivery
The biomedicine sector reaps significant benefits from DLS applications, particularly in drug delivery systems. With the growing emphasis on targeted therapies, understanding the size and distribution of drug carriers like liposomes or polymeric nanoparticles becomes paramount.
The benefits of applying DLS in this context are manifold:
- Effective Drug Formulation: DLS enables the formulation of drug carriers that optimize delivery efficacy, ensuring that therapeutic agents maintain their intended functionality.
- Real-Time Monitoring: As drug delivery systems are administered in vivo, DLS can facilitate real-time assessments of their behavior and interactions within biological systems.
- Customized Medicine: By screening nanoparticles based on size and surface properties using DLS, personalized treatment options can be developed, improving patient outcomes.
Overall, deploying DLS in drug delivery research offers a robust framework for improving therapeutic strategies, ultimately targeting diseases more effectively.
Environmental Monitoring
Environmental applications of DLS are increasingly recognized, as tracking nanoparticles in environmental samples helps assess ecological impacts. Nanoparticles can enter water systems through various means, making their detection crucial for studying pollution and environmental safety.
Key areas where DLS contributes include:
- Detection of Contaminants: DLS can help identify and measure the size and distribution of harmful nanoparticles present in water or soil samples, providing insights into pollution levels.
- Monitoring Remediation Processes: In cases where remediation of polluted sites is necessary, DLS can be employed to monitor changes in particle size that indicate the success of treatments.
- Research on Nanomaterials: Understanding the fate of engineered nanomaterials in the environment is vital for developing better materials that minimize risks to human health and ecosystems.
"The application of DLS for environmental monitoring underscores its versatility and adaptability, reflecting the contemporary needs for reliable analysis tools in the face of growing environmental challenges."
In short, the multitude of applications stemming from DLS in characterizing nanoparticles illustrates its importance in both scientific research and practical applications across various sectors. The ability to provide accurate measurements quickly and more efficiently than traditional methods enhances our understanding of nanoparticles, driving innovations and solutions to modern-day challenges.
Challenges in DLS Analysis
Dynamic Light Scattering (DLS) is a powerful method for characterizing nanoparticles, yet, like any scientific technique, it comes with its share of challenges. Recognizing and addressing these challenges is crucial for obtaining accurate results. Analysts need to be aware of various factors that may influence the measurements, as this can have direct implications for the reproducibility and reliability of the data. A well-rounded understanding of these challenges enables researchers to make informed decisions and choose the most appropriate methodologies for their nanoparticle studies.
Impact of Aggregation
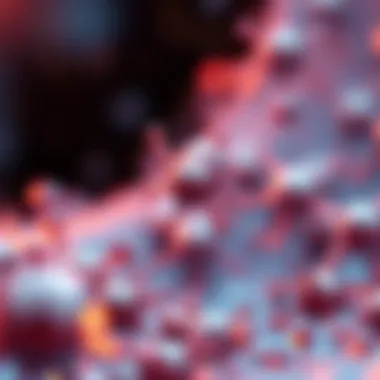
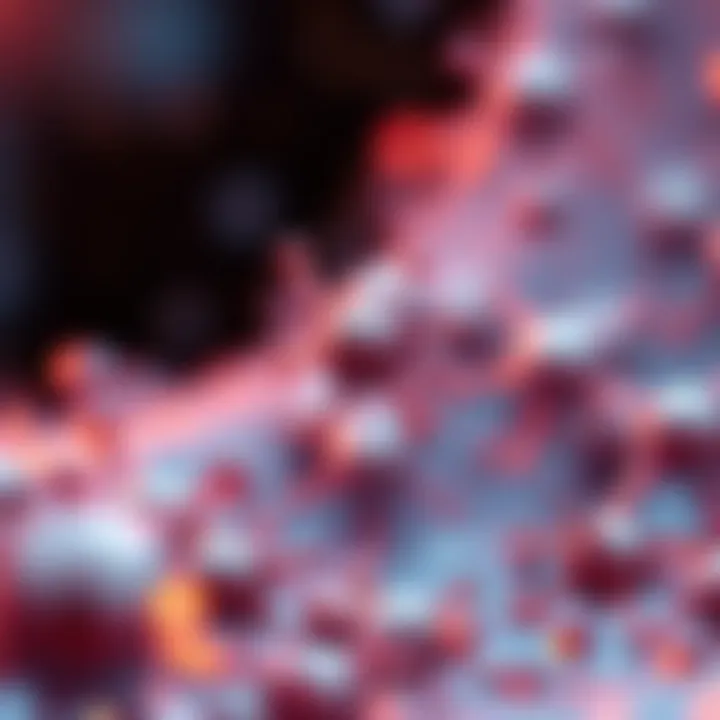
One of the most significant challenges in DLS analysis is the impact of aggregation on particle size determination. When nanoparticles aggregate, they form clusters that can skew the results dramatically. Aggregates appear larger than individual nanoparticles, potentially leading to misleading interpretations concerning the size distribution.
It’s imperative to maintain system homogeneity, which means ensuring that the sample remains dispersed and doesn’t form clumps during analysis. Not just that, but the presence of impurities or external agents can also exacerbate aggregation, resulting in disturbances in the scattering signal.
To counteract these effects, researchers often employ various techniques:
- Ultrasonication: This helps disperse the particles and break down aggregates.
- Choice of solvents: The use of selective solvents can stabilize the dispersion and minimize the likelihood of aggregation.
- Continuous monitoring: Employing real-time data monitoring can help identify aggregation events as they happen, allowing for immediate corrective actions.
Being meticulous at this stage ensures not only the accuracy of the measurements but also the robustness of the conclusions drawn from them.
Limitations of Detection Sensitivity
Detection sensitivity is another critical aspect that can constrain DLS analysis. While advancements in technology have improved sensitivity, limitations still exist. DLS techniques are often best suited for nanoparticles in the range of 1 nm to 1 µm. However, particles that fall outside this range, either being too small or too large, can be challenging to evaluate reliably.
Factors contributing to sensitivity limitations include:
- Background noise: This can occur from solvents, laboratory equipment, or other environmental influences, which may mask signals from the nanoparticles.
- Concentration levels: High concentrations can lead to multiple scattering events, complicating size determinations.
- Refractive index matching: The accuracy of size determinations is highly dependent on knowing the correct refractive indices of both the nanoparticles and the dispersant. Any discrepancies here can lead to significant errors.
It’s crucial for practitioners to recognize these limitations. By tailoring DLS setups and continuously refining methodologies, researchers can improve detection sensitivity and ensure more reliable size characterization.
"In the world of nanoparticle analysis, precision is not just a goal; it is an absolute necessity."
Understanding these challenges sets the stage for enhanced analytical strategies and, ultimately, for the broader advancement of applications relying on DLS data. By actively engaging with these issues, one can navigate the complexities of DLS methods effectively.
Recent Advances in DLS Technology
In the rapidly evolving field of nanoparticle characterization, Dynamic Light Scattering (DLS) continues to push the boundaries with innovative technological advancements. Understanding these updates is vital, as they enhance the capability of DLS to yield more accurate and insightful data. These advancements not only refine measurement precision but also expand the range of applications in diverse scientific domains.
Innovations in Instrumentation
Modern DLS devices incorporate cutting-edge technology that improves the reliability of measurements. Some key innovations in instrumentation include:
- Increased sensitivity: New detectors can capture weaker signals, enabling analysis of smaller concentration samples.
- Angle-resolved DLS (AR-DLS): This technique allows for the measurement of particle size distribution across different scattering angles, enhancing data richness.
- Temperature control features: Adjusting temperature during analysis can help in understanding the thermodynamic behavior of nanoparticle dispersions.
- Integrated software solutions: Advanced software packages now utilize machine learning algorithms to enhance data processing efficiency, offering real-time analysis of complex datasets.
These instrumental advancements not only heighten precision but also simplify the overall user experience, making DLS more accessible to researchers.
Emerging Techniques and Enhancements
Alongside instrumentation advances, several new methodologies have emerged in DLS that hold promise for increased analytical capabilities. Some notable techniques include:
- Multi-angle DLS: This approach combines multiple scattering angles to ascertain a more nuanced understanding of the particle size distribution.
- Label-free DLS: Recent innovations allow for the analysis of samples without fluorescent or heavy metallic labels, improving sample integrity.
- DLS combined with microscopy techniques: This hybridization allows for a visual confirmation of particle characteristics seen in DLS, which can confirm suspicions derived from DLS data alone.
"With these emerging techniques, DLS analysis transitions from mere physical property analysis to a comprehensive characterization toolkit."
As the landscape of nanoparticle characterization continues to evolve, the integration of these innovations is proving essential for addressing complex challenges across industries such as pharmaceuticals, materials science, and even environmental monitoring. Staying abreast of these innovations will be critical for researchers aiming to enhance their analytical repertoires.
Future Directions in DLS Research
As the field of nanoparticle science progresses at a brisk pace, the future directions in Dynamic Light Scattering (DLS) research become ever more critical. DLS is not simply a method for particle size analysis; it represents a gateway to unlocking diverse applications, enhancing the understanding of materials at the nanoscale. There are specific elements that researchers and professionals in the scientific community should contemplate when considering the future of DLS.
Integration with Other Analytical Techniques
The intersection of DLS with other analytical techniques holds great potential. Combining DLS with microscopy methods like Scanning Electron Microscopy (SEM) and Transmission Electron Microscopy (TEM) can allow for complementary insights. For instance, while DLS provides size distributions in a bulk sample, microscopy offers a visual confirmation of individual particle shapes and structures. This holistic approach offers deeper insights into particle behavior in real-world applications.
- Here are a few analytical techniques that can be integrated with DLS:
- Microfluidics: When coupled with DLS, microfluidics can help in studying particle behavior under varying fluid dynamics and conditions.
- Nuclear Magnetic Resonance (NMR): NMR could provide additional information about the interactions between nanoparticles and their surrounding environment.
- X-ray Diffraction (XRD): This method can confirm crystalline structures, complementing the size data from DLS.
Benefits of this integration include improved accuracy in data interpretation and a more thorough understanding of complex nanoparticle systems, making it a vital area for future exploration.
Potential in New Applications
The adaptability of DLS facilitates its application in several emerging fields beyond conventional studies. As industries evolve and nanotechnology expands its reach, identifying and understanding these new applications is vital.
- Nanomedicine: DLS can be pivotal in the characterization of drug delivery systems, especially nanoparticles that carry therapeutics. By analyzing particle size and distribution, researchers can fine-tune the properties of drug formulations, enhancing their efficacy and safety in clinical settings.
- Energy Storage: Nanoparticles play a critical role in the development of advanced batteries and supercapacitors. DLS can help evaluate the size and distribution of materials used in electrodes, impacting their performance significantly.
- Environmental Applications: Monitoring pollutants at the nano-level is emerging as a priority. DLS can aid in tracking how nanoparticles interact with biological systems or degrade in the environment, informing regulatory policies and remediation strategies.
In sum, the prospects for DLS in various new applications are not just promising but essential, indicating a paradigm shift in its utilization within scientific research and industry.
As the link between research and real-world applications grows stronger, the future of DLS will likely influence many fields, steering advancements in technology and science alike.
Ending
In wrapping up our exploration of Dynamic Light Scattering (DLS) as a pivotal technique for nanoparticle characterization, it ’s essential to understand its substantial contributions across various fields. The manipulation of nanoparticles is crucial in contemporary science, particularly regarding their application in biomedicine, materials science, and nanotechnology. This article has established a foundation for appreciating DLS not just as a technique, but as a lens through which we can gain insights into the behavior and properties of nanoparticles.
Summary of Key Insights
The key takeaways from our discussion underscore several vital aspects of DLS:
- Versatility of Application: DLS finds itself employed in diverse areas including drug delivery, quality control in manufacturing, and environmental monitoring. Each of these applications demonstrates the method’s flexibility and efficiency.
- Methodological Integration: Innovative advancements are pushing DLS to combine with other analytical methods, like electron microscopy and atomic force microscopy. Such integrations promise deeper understanding and more robust analysis.
- Challenges Ahead: Despite its advantages, DLS isn't without obstacles. Factors like sample aggregation and sensitivity limits present challenges that scholars and practitioners must navigate.
- Future Prospects: The future of DLS is bright, with new technologies surfacing that may enhance its analytical capabilities. As research progresses, we can anticipate the emergence of novel applications, particularly in tailored drug development and nanomaterial engineering.
To conclude, emphasizing the importance of DLS in the scientific community is paramount. It acts not just as an analytical technique, but as a bridge connecting theoretical knowledge with practical applications. As we continue to refine our understanding of nanostructures, DLS will likely evolve, ushering in new frontiers in science and technology.