Understanding the DNA Mutation in Sickle Cell Anemia
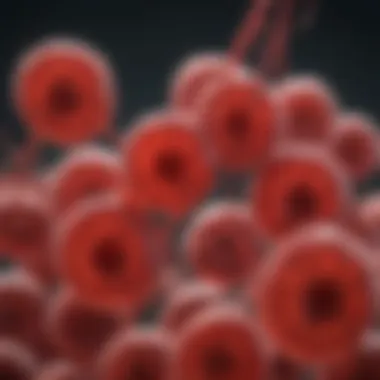
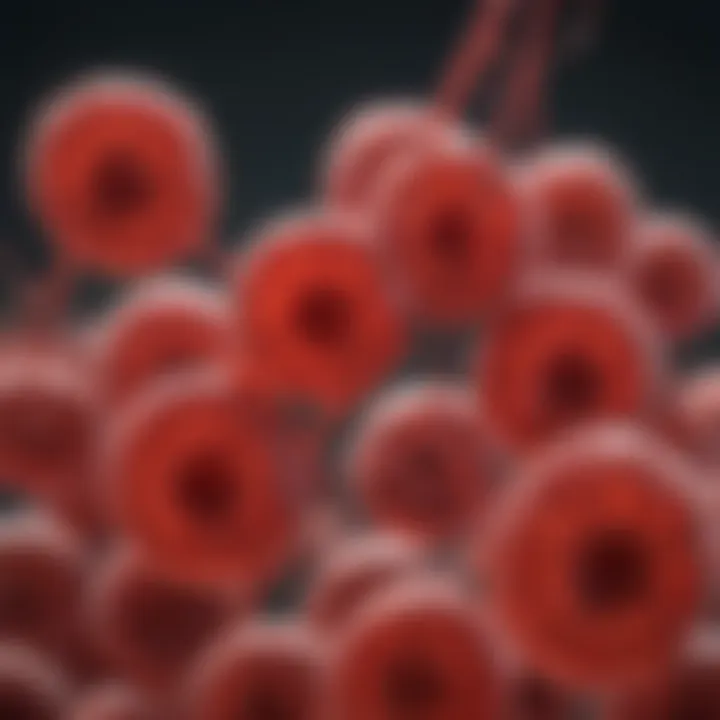
Intro
Sickle cell anemia is a genetic disorder that alters the shape and functionality of red blood cells. This condition primarily results from a specific mutation in the DNA sequence coding for hemoglobin, the protein responsible for oxygen transport in the bloodstream. Understanding the molecular basis of this mutation is crucial for comprehending the broader implications of the disease, including its inheritance, pathology, and treatment strategies. The interconnected aspects of DNA mutations, genetic research, and clinical outcomes highlight the significance of this area in modern medicine.
Methodologies
The study of sickle cell anemia has evolved through various research methodologies over the years, allowing scientists to better understand the mutation and its effects on hemoglobin.
Description of Research Techniques
One fundamental technique used is DNA sequencing. This allows researchers to read the genetic code and identify the exact mutation. In sickle cell anemia, the mutation is a single nucleotide substitution where adenine (A) is replaced by thymine (T) in the hemoglobin gene, resulting in the substitution of glutamic acid by valine in the protein sequence.
Other techniques include polymerase chain reaction (PCR), which amplifies small segments of DNA, making it easier to analyze genetic variations. Additionally, genetic screening has become a prevalent method for identifying carriers of the sickle cell trait in populations at higher risk.
Tools and Technologies Used
Recent advancements in genetic analysis tools have transformed the study of sickle cell anemia. Technologies such as CRISPR gene editing enable precise modifications of genes, opening potential avenues for therapeutic interventions. Bioinformatics tools assist in analyzing genetic data, enhancing our understanding of the mutation's implications on cellular function.
Researchers also use hemoglobin electrophoresis to separate different types of hemoglobin. This technique is instrumental in diagnosing sickle cell disease by revealing the presence of abnormal hemoglobin S (HbS).
Discussion
Understanding the mutation behind sickle cell anemia not only sheds light on the disorder itself but also contributes to the broader field of genetic research. The theoretical implications of this knowledge extend beyond the disease.
Comparison with Previous Research
Historically, studies on sickle cell disease have provided insights into population genetics and hereditary conditions. Earlier research primarily focused on the clinical manifestations of the disease. Modern studies utilize advanced genetic techniques that permit a more granular view of the mutation's effects at the molecular level. These developments have vastly improved our comprehension of the disorder and its treatment options.
Theoretical Implications
The implications of identifying the specific mutation impact various medical fields, including pharmacogenomics and gene therapy. Understanding the genetic basis of sickle cell anemia paves the way for targeted therapies, which can be more effective compared to traditional treatments. This genetic insight also emphasizes the importance of genetic counseling in at-risk populations, preventing the perpetuation of the disease through informed reproductive choices.
"Understanding the underlying genetic mutations is essential for the future of personalized medicine and effective treatment strategies."
By articulating the methodologies and discussions surrounding sickle cell anemia, we illuminate the intricacies of genetic research. It also reinforces the need for continual exploration in the field to unravel the complexities of genetic disorders.
Prolusion to Sickle Cell Anemia
Sickle cell anemia represents a significant public health concern and is one of the most studied genetic disorders. Understanding this condition is crucial, as it reflects not only the complexities of human genetics but also the broader implications for healthcare management. This introduction lays the foundation for deeper exploration into the genetic mutation associated with sickle cell anemia and its impacts on individuals and society.
Sickle cell anemia is characterized by the presence of abnormal hemoglobin, known as hemoglobin S. This leads to the deformation of red blood cells into a crescent or sickle shape. These abnormally shaped cells can cause various complications, including pain crises and organ damage. Many people affected by the condition struggle with symptoms throughout their lives, making early detection and management vital.
Moreover, sickle cell anemia's historical and social context provides valuable insights into how genetic disorders are perceived and treated across different communities. This underscores the importance of research and educational initiatives that promote a better understanding of the condition.
As we move into the specifics of sickle cell anemia, we must first define what it is and explore its historical context. This will help frame the more intricate discussions around its genetic basis and the implications for individuals affected by the disorder.
Defining Sickle Cell Anemia
Sickle cell anemia is a genetic blood disorder caused by a single alteration in the DNA sequence of the HBB gene. This gene is responsible for producing the beta-globin subunit of hemoglobin. The mutation involves the substitution of adenine with thymine at the sixth codon of the beta-globin gene, leading to the production of hemoglobin S instead of the normal hemoglobin A.
This change in hemoglobin structure causes red blood cells to become rigid and take on a sickle shape under low oxygen conditions. Unlike typical disc-shaped red blood cells, sickled cells are less flexible and can block blood flow in small vessels, causing pain and potential organ damage.
Knowing the biological underpinnings of this disorder is essential for understanding how it is inherited, the associated symptoms, and the treatment options available.
Historical Context
The historical context of sickle cell anemia is layered and multifaceted. The earliest references to the disease can be traced back to ancient Egypt. However, it was not until the early 20th century that medical science began to understand the genetic basis of the disorder. In 1910, Dr. James Herrick first described the disease, simplifying the complex banking of its cellular characteristics.
Throughout the years, several key discoveries have emerged regarding its inheritance patterns and implications. The realization that sickle cell anemia is an autosomal recessive trait steered further research on genetic disorders. As scientists delved into this area, they uncovered the wider prevalence of the allele in individuals of African descent, leading to discussions around migration patterns and the evolutionary advantages the trait must have offered in the past, particularly against malaria.
In more recent times, advancements in genetic research, such as CRISPR technology, have paved the way for potential curative approaches. The conversation surrounding sickle cell anemia extends into public health strategies, ethical considerations in genetic testing, and the necessity for educational outreach aimed at communities disproportionately affected.
Overall, the historical data adds layers of understanding to the clinical and social aspects of sickle cell anemia, enriching the discourse on its genetics and treatment.
Genetic Basis of Sickle Cell Anemia
The genetic foundation of sickle cell anemia holds significant importance in understanding this disorder. This section delves into key elements like the nature of DNA, types of mutations, and the specific mutation leading to sickle cell anemia. Grasping these aspects is essential for students, researchers, and professionals as it provides insight into not just the disease but also the broader implications of genetic disorders. Recognizing how these mutations arise can lead to better diagnostic and treatment strategies.
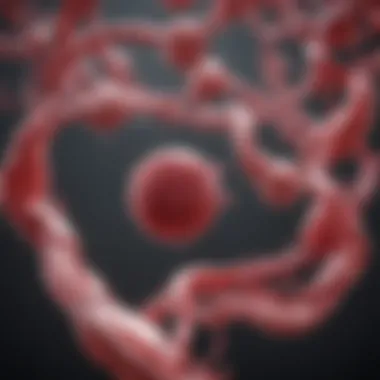
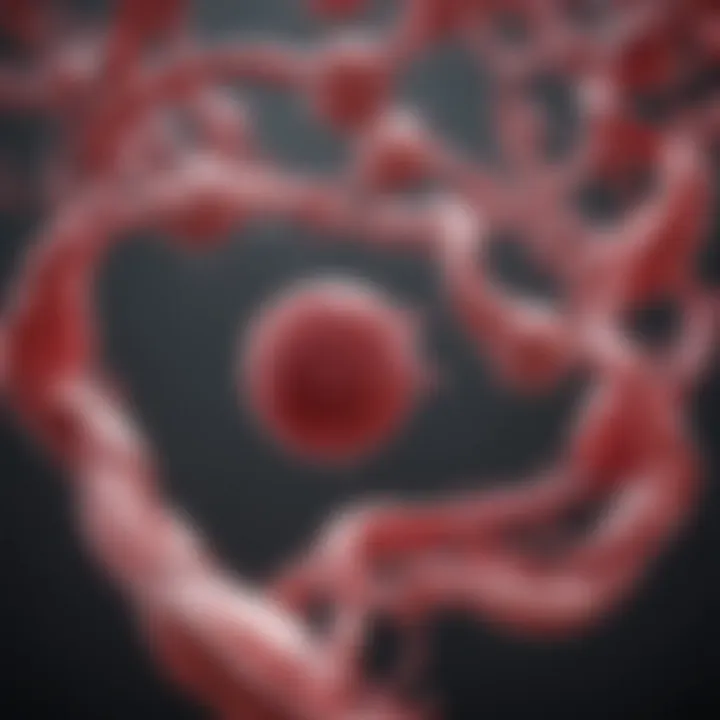
Understanding DNA and Genes
DNA, or deoxyribonucleic acid, carries the genetic blueprint for all living organisms. It consists of sequences of nucleotides, which are the building blocks of genes. Each gene encodes instructions for the synthesis of proteins that perform crucial functions in the body. In the context of sickle cell anemia, the relevant gene is the HBB gene, located on chromosome 11. This gene is responsible for producing beta-globin, a component of hemoglobin.
Genes can be seen as a set of instructions, encoding not only physical features but also various traits including susceptibility to diseases. Understanding how the HBB gene operates provides insight into how mutations can lead to severe health issues like sickle cell anemia.
Types of DNA Mutations
Mutations in DNA can occur in several ways, and understanding these types can clarify how diseases develop. Here are common types of mutations:
- Substitutions: One nucleotide is replaced by another. This type of mutation is significant in sickle cell anemia.
- Insertions: Extra nucleotides are added into the DNA sequence.
- Deletions: Nucleotides are removed from the DNA sequence.
Among these mutations, substitutions are particularly relevant for sickle cell anemia as they directly result in changes to the hemoglobin structure.
Mutation Leading to Sickle Cell Anemia
The mutation responsible for sickle cell anemia is a single nucleotide substitution. Specifically, a thymine (T) is replaced with adenine (A) in the HBB gene. This seemingly minor change has dramatic effects. As a result, the amino acid glutamic acid is replaced with valine in the beta-globin chain. This alteration causes hemoglobin molecules to aggregate under low oxygen conditions, leading to the characteristic sickle-shaped red blood cells.
This mutation not only explains the physiological manifestations of the disease but also illuminates the genetic inheritance patterns that follow from it. The insight into this mutation underscores the critical need for continued research in genetics, as it amounts to more than just understanding one disease—it opens pathways for diverse therapeutic interventions and better public health policies.
Mechanism of the Mutation
Understanding the mechanism behind the DNA mutation that causes sickle cell anemia is crucial for grasping the broader implications of the disease. This knowledge aids in decoding how a single genetic alteration can lead to significant physiological and health challenges. Additionally, comprehending these mechanisms lends insight into potential treatment strategies and preventive measures.
Single Nucleotide Substitution
The pivotal event in sickle cell anemia arises from a specific alteration in the DNA sequence of the hemoglobin gene, HBB. This alteration is a single nucleotide substitution, where an adenine (A) is replaced by thymine (T) at the sixth codon of the beta-globin gene. The codon changes from GAG to GTG, resulting in the amino acid change from glutamic acid to valine in the hemoglobin protein. This seemingly minor modification leads to profound changes in hemoglobin properties.
This substitution is significant because it transforms the normal hemoglobin A (HbA) into sickle hemoglobin (HbS). The structural integrity of hemoglobin is vital for the proper transport of oxygen. When the structure of hemoglobin changes as a result of this mutation, it causes the red blood cells to deform into a sickle shape. Such red blood cells have a reduced ability to flow through small blood vessels, leading to various clinical complications.
"A single nucleotide change can shift one’s health destiny, highlighting the delicate balance of genetics"
Effects on Hemoglobin Structure
The transition from HbA to HbS due to the single nucleotide substitution results not just in a simple protein modification but brings about complex biochemical repercussions. Sickle hemoglobin has a tendency to polymerize under low oxygen conditions. This polymerization causes red blood cells to become rigid and distorted, straying from their normal biconcave disc shape.
The abnormal structure leads to several consequences:
- Reduced Oxygen Carrying Capacity: Sickled cells cannot transport oxygen efficiently, leading to symptoms of anemia.
- Blockage of Blood Flow: The deformed cells can aggregate and plug small capillaries, causing pain crises and organ damage due to decreased blood supply.
- Increased Hemolysis: Sickled cells have a shorter lifespan, resulting in higher rates of destruction in the spleen.
These structural changes not only create immediate health issues but set the stage for chronic complications in multiple organ systems. Thus, understanding both the single nucleotide substitution and its downstream effects provides a comprehensive insight into the pathophysiology of sickle cell anemia.
Inheritance Patterns
Understanding the inheritance patterns of sickle cell anemia is crucial. This section offers insights into the mechanisms by which the disease is passed from generation to generation. This information is vital for families affected by the condition and for healthcare professionals making informed decisions on management and genetic counseling.
Autosomal Recessive Inheritance
Sickle cell anemia follows an autosomal recessive inheritance pattern. This means that two copies of the sickle cell allele, one from each parent, are necessary for a child to have the disease. When both parents carry one copy of the mutated gene, there are several possible outcomes for their offspring:
- Normal carrier (non-affected): One in four chances. The child inherits two normal alleles.
- Carrier: Two in four chances. The child inherits one normal and one sickle cell allele. They carry the trait but do not exhibit symptoms of the disease.
- Affected: One in four chances. The child inherits two sickle cell alleles, resulting in sickle cell anemia.
This pattern can have significant implications for family planning. Parents who are carriers can choose to undergo genetic testing to assess the likelihood of their children being affected.
Risk of Offspring Inheritance
The risk of offspring inheriting sickle cell anemia is not just a matter of chance. It depends on genetic counseling, family history, and understanding the probabilities based on the inherited alleles. Here are key considerations:
- Genetic Testing: It is advisable for prospective parents, especially those with a family history of sickle cell anemia, to undergo carrier screening.
- Counseling: Genetic counselors can assist families in understanding the testing results and what they mean for future pregnancies.
- Awareness: Being informed about inheritance risks allows families to make educated decisions. Awareness of sickle cell trait can help in preparing for health implications associated with the disease.
Understanding inheritance patterns is not just academic; it has real-life implications for individuals and families navigating the complexities of sickle cell anemia.
Sickle cell anemia has far-reaching consequences beyond just the health of individuals. The inheritance patterns affect public health initiatives, genetic research, and healthcare policy geared towards managing and treating this chronic condition.
Clinical Manifestations
The clinical manifestations of sickle cell anemia are a central focus of this article. Understading these manifestations is crucial for identifying, managing, and treating the disease effectively. The symptoms and complications that arise from sickle cell anemia can significantly impact the quality of life for patients. By examining these clinical aspects, healthcare providers can foster better patient outcomes and enhance their overall approach to care.
Symptoms of Sickle Cell Anemia
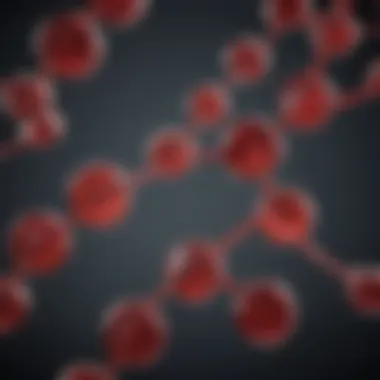
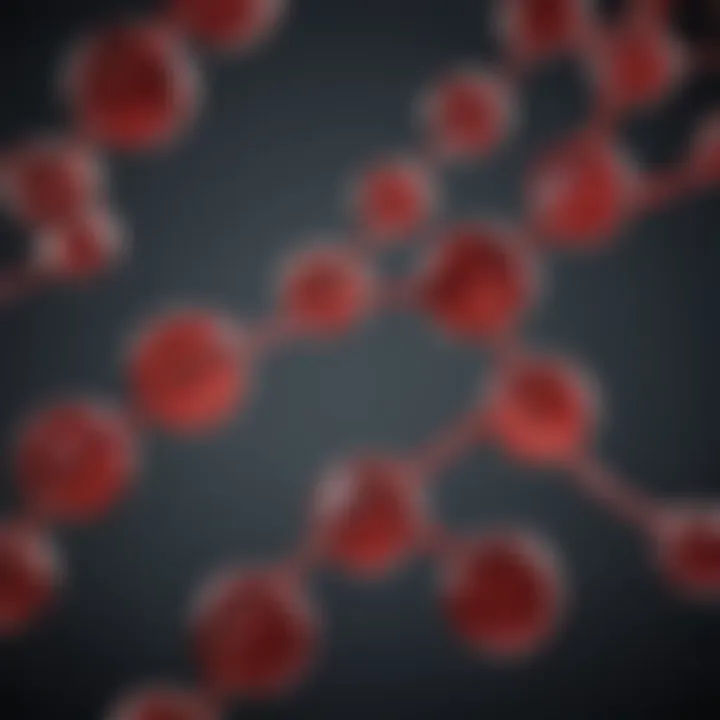
Sickle cell anemia presents with a variety of symptoms that often vary in severity. The primary symptoms typically include:
- Anemia: A result of the rapid destruction of sickle cells, leading to fatigue and weakness.
- Pain Crises: Also known as vaso-occlusive crises, these episodes are often sudden and painful. Pain can occur anywhere in the body, commonly in the bones and chest.
- Swelling: Swelling in the hands and feet, known as dactylitis, can occur due to blocked blood flow.
- Frequent Infections: Sickle cells can damage the spleen, making individuals more susceptible to infections.
- Delayed Growth: Children with sickle cell anemia may experience slower growth and delayed puberty.
Individuals with sickle cell anemia often experience fluctuating symptoms, leading to a complex clinical picture that can evolve over time.
Patients may notice that their symptoms can be triggered by factors such as dehydration, extreme temperatures, or high altitudes. Hence, understanding these triggers is vital for effective management and prevention strategies.
Complications Associated with the Disease
The complications of sickle cell anemia merit serious consideration. They can be life-threatening and can present a substantial burden on affected individuals. Recognizing potential complications helps in early intervention and ongoing management. Some common complications include:
- Acute Chest Syndrome: A serious condition involving lung-related symptoms, often requiring hospitalization.
- Stroke: Blocked blood flow to the brain can occur due to sickle cells, leading to strokes.
- Organ Damage: Continuous sickling can result in damage to organs such as the kidneys and liver.
- Leg Ulcers: These are open sores that can form due to poor blood circulation.
- Pulmonary Hypertension: High blood pressure in the lungs can develop, causing shortness of breath and fatigue.
Understanding these complications is vital for both patients and healthcare professionals. It empowers them to take proactive steps in monitoring symptoms and seeking care when necessary.
In summary, the clinical manifestations of sickle cell anemia highlight the importance of awareness and education surrounding the disease. By emphasizing symptoms and complications, healthcare providers can create targeted treatment plans that address the needs of individual patients.
Diagnosis of Sickle Cell Anemia
The diagnosis of sickle cell anemia is a crucial step in the management of this genetic disorder. Early detection can significantly improve patient outcomes, reducing the risk of severe complications. Therefore, understanding the various methods for diagnosing sickle cell anemia is essential for healthcare professionals, patients, and families affected by this condition.
Screening Methods
Screening for sickle cell anemia typically involves a blood test. This test is designed to identify the presence of hemoglobin S, the abnormal form of hemoglobin that characterizes this disorder. The two primary screening methods include:
- Hemoglobin Electrophoresis: This test separates different types of hemoglobin in the blood. By applying an electric field, various hemoglobin molecules migrate at different speeds. The results provide a clear picture of hemoglobin types present, including normal hemoglobin A and abnormal hemoglobin S.
- Newborn Screening: Most countries have implemented newborn screening programs. Blood samples taken shortly after birth are screened for sickle cell disease. Early detection allows for timely management and prevention of complications.
Other screening methods may include high-performance liquid chromatography (HPLC), which also separates hemoglobin types with high accuracy. Each method has its advantages and limitations, so it is essential to choose one based on the clinical context and available resources.
Confirmatory Tests
Once a screening test indicates the possibility of sickle cell anemia, confirmatory tests are performed to verify the diagnosis. These tests are thorough and aim to confirm the presence of hemoglobin S and determine the specific genotype. Key confirmatory tests include:
- Genetic Testing: This test analyzes the DNA for mutations in the HBB gene responsible for producing hemoglobin. Identifying specific mutations can help in understanding the disease's severity and can guide treatment decisions.
- Complete Blood Count (CBC): A CBC provides information about the overall health of the patient. It measures red blood cells, hemoglobin levels, and other components. Low hemoglobin levels may indicate anemia, which is often present in sickle cell anemia.
- Reticulocyte Count: This test measures the number of young red blood cells. Elevations in reticulocyte counts can indicate recent hemolysis, a common aspect of sickle cell anemia due to the destruction of sickled red blood cells.
Early diagnosis is not just about confirming sickle cell anemia; it sets the stage for monitoring and healthcare interventions that can improve quality of life.
In summary, the diagnosis of sickle cell anemia involves a combination of screening and confirmatory tests. Understanding these processes is vital, as early and accurate diagnosis can lead to appropriate management strategies. The focus on timely diagnoses can help alleviate symptoms and reduce the occurrence of complications associated with the disease.
Treatment Options
The treatment of sickle cell anemia requires a multifaceted approach to manage symptoms and prevent complications. This section is signficant for understanding how patients can achieve a better quality of life and potential avenues for curative strategies. The complexity of sickle cell anemia necessitates treatments that are both preventive and responsive to the crisis events the disease can induce.
Managing Symptoms
Symptom management is essential in the treatment strategy for sickle cell anemia. Many patients experience pain episodes, known as sickle cell crises, which can vary in frequency and intensity. Therefore, effective management strategies are required to help alleviate pain and maintain patients’ comfort. Here are several key methods:
- Pain Relief: Opioids are often required for severe pain management. Non-steroidal anti-inflammatory drugs (NSAIDs) can also be beneficial for milder pain symptoms. Pain management must be personalized to each patient's specific needs.
- Hydration: Maintaining adequate hydration is critical. It helps to reduce blood viscosity, potentially decreasing the risk and severity of sickle cell crises.
- Blood Transfusions: Regular blood transfusions can help reduce the risk of complications. Transfusions provide healthy red blood cells which can enhance oxygen delivery to tissues.
- Antibiotics and Vaccinations: Patients with sickle cell anemia are at higher risk for infections. Prophylactic antibiotics and timely vaccinations can prevent infections that may exacerbate symptoms.
- Management of Complications: Regular monitoring for organ complications, such as spleen dysfunction or kidney disease, is necessary to prevent further health issues.
These strategies can significantly improve the quality of life for patients, reducing the frequency and severity of crises. Systematic use of management tactics requires a coordinated effort among healthcare providers and patients.
Curative Approaches
While managing symptoms is crucial, there are also curative approaches emerging in the treatment of sickle cell anemia. These include:
- Bone Marrow Transplantation: This remains the only established curative procedure for sickle cell anemia. However, it is typically reserved for individuals with severe disease, given the risks associated with the procedure. Finding a suitable donor is also a significant challenge.
- Gene Therapy: Advances in gene therapy are showing promise. Techniques involve modifying the patient's own cells to correct the mutation responsible for the disease. Early clinical trials have yielded encouraging initial results, suggesting that gene therapy might become a viable option in the future.
- CRISPR-Cas9 Technology: This innovative approach utilizes gene editing to directly alter genetic sequences involved in sickle cell disease. Early studies are exploring its effectiveness in changing or repairing the faulty gene.
- Medications: Certain drugs are being investigated for their potential to increase fetal hemoglobin levels in patients, which may reduce sickling episodes. Hydroxyurea is one such medication, which has been shown to reduce pain crises and may also prevent organ damage.
Current research in sickle cell anemia is focused on both improving existing treatments and finding new curative options.
The drive towards combining these advanced therapies presents a hopeful frontier for research. It is important to continue monitoring ongoing trials and studies that may offer new insights into curative options for the disease.
In summary, treatment options for sickle cell anemia consist of managing symptoms and exploring promising curative strategies. As research evolves, the hope for a more definitive treatment is becoming more tangible.
Research and Advances
The field of research concerning sickle cell anemia is critical for understanding the nuances of this complex disease. Advancements in genetic and biochemical research enable a more profound insight into the mutation responsible for sickle cell anemia. The implications of these findings stretch beyond treatment; they affect clinical practices, public health approaches, and patient education. The benefits of ongoing research include the refinement of diagnostic tools, enhanced treatment strategies, and the development of novel therapies that can improve the quality of life for those affected.
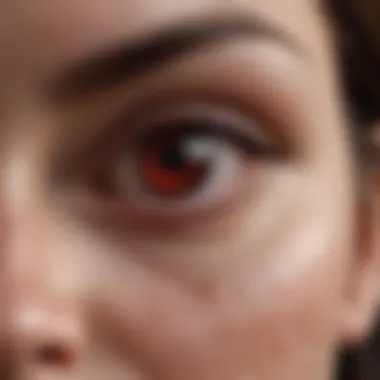
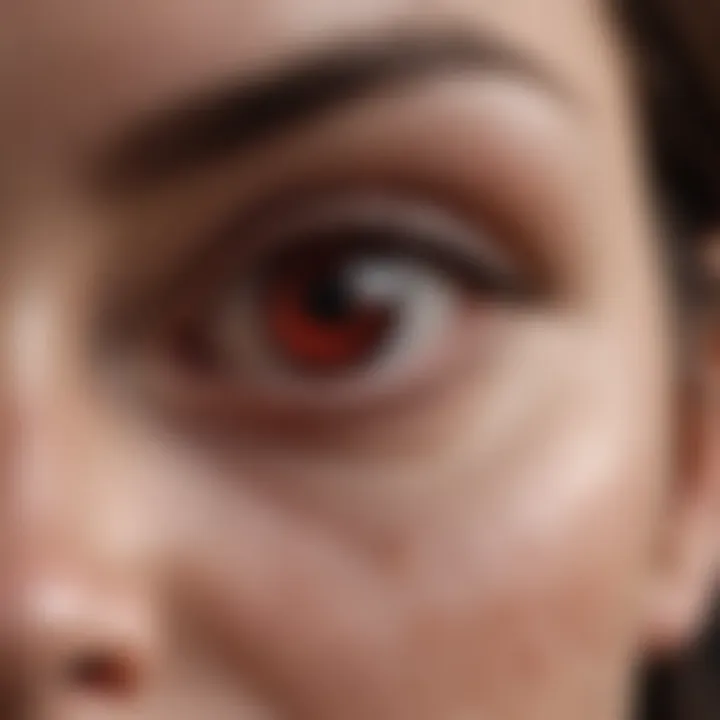
Current Research Trends
Recent studies focus on various aspects of sickle cell anemia. One significant trend is exploring gene therapy as a potential curative approach. Researchers are investigating how techniques such as CRISPR-Cas9 can be utilized to modify the gene responsible for producing abnormal hemoglobin. This has provided promising results in initial studies, suggesting that it may be possible to correct the underlying mutation, offering a permanent solution for patients.
Another area receiving attention includes the enhancement of hydroxyurea treatment. Hydroxyurea has been found to increase fetal hemoglobin levels, reducing sickle cell crises. Ongoing trials are considering how to optimize its use, particularly in combination with other drugs. This combined approach aims to maximize patient benefits and minimize side effects.
Furthermore, there is a growing interest in identifying biomarkers that can predict the severity of the disease. Understanding biological indicators can lead to more personalized medicine approaches, allowing healthcare providers to tailor treatments based on individual patient profiles. By understanding which patients are likely to face severe complications, clinicians can take proactive measures to manage their health effectively.
Future Directions in Treatment
The future of treatment for sickle cell anemia holds much promise. Gene therapy, as mentioned, is at the forefront of research. There are ongoing clinical trials that explore various methodologies of gene editing to provide long-lasting relief from symptoms and complications. Should these trials prove successful, they may redefine how sickle cell anemia is treated globally.
Additionally, research into small molecular agents that can stabilize hemoglobin structure is gaining traction. These agents could potentially prevent red blood cells from sickling by improving the properties of hemoglobin itself. Considering the fundamental nature of the hemoglobin mutation in sickle cell anemia, these innovations could lead to breakthroughs in management.
Finally, public health strategies may evolve significantly due to research findings. Community outreach and education around early diagnosis and prevention of complications can be enhanced through data-driven approaches. Focusing on health equity will ensure that afflicted populations receive adequate care and have access to emerging therapies.
The confluence of genomic advancements and innovative treatments will pave the way for a new era in managing sickle cell anemia, moving towards more effective and personalized healthcare solutions.
Broader Implications
Understanding the implications of sickle cell anemia goes beyond the individual patient experience. The disease serves as a crucial model for studying genetic disorders and their effects on public health systems worldwide. An awareness of the broader ramifications of sickle cell anemia can inform genetic research, public health initiatives, and healthcare policies.
An essential consideration is the burden that sickle cell anemia places on healthcare resources. There is a significant need for early diagnosis and proactive management of the disease to mitigate its health complications, which can range from painful crises to severe organ damage. Public health programs must account for this when allocating resources, especially in regions with a high prevalence of the disease.
Moreover, as researchers continue to delve into the genetic underpinnings of sickle cell anemia, valuable insights emerge that can be applied to other genetic disorders. For instance, understanding how mutations translate into clinical manifestations opens avenues for novel therapeutic strategies. It highlights the need for genetic counseling that can help families understand their risks, particularly in communities where sickle cell anemia is common.
"Addressing genetic disorders like sickle cell anemia offers a pathway to improve public health outcomes and reduce health disparities globally."
From an educational perspective, sickle cell anemia underscores the importance of integrating genetics into medical training. Health professionals equipped with knowledge about genetic conditions can make better-informed decisions in patient care. This, in turn, can lead to improved health outcomes for patients and their families.
Finally, insights gained from sickle cell anemia research can foster empathy and understanding within society. Through advocacy and awareness programs, individuals and communities can work together to dismantle stigma surrounding genetic diseases, encouraging a more supportive environment for those affected. Thus, the implications of sickle cell anemia extend well into the realms of policy, education, and societal attitudes, all of which are crucial for fostering a holistic approach to public health.
Sickle Cell Anemia and Public Health
Sickle cell anemia presents a distinct challenge to public health, particularly in countries with a significant population of individuals carrying the sickle cell trait. The disorder is not merely a clinical issue; it also has extensive social and economic ramifications. The public health burden is evident in increased hospital admissions, longer hospital stays, and the need for continuous healthcare support.
Governments and health organizations must implement comprehensive screening programs at birth to identify affected individuals early. Early intervention can drastically reduce the severity of complications associated with the disease. Additionally, public awareness campaigns are vital to educate communities on the genetic nature of the disorder. This helps to promote informed health choices among affected families and communities.
Moreover, the intersection of sickle cell anemia and economic factors cannot be overlooked. Patients often face challenges in accessing quality care due to financial limitations. This emphasizes the responsibility of public health systems to provide affordable treatment options, including pain management and preventive care.
- Key Strategies for Public Health Initiatives:
- Enhance newborn screening programs.
- Facilitate access to genetic counseling.
- Improve collaboration between healthcare providers.
- Increase funding for research on sickle cell anemia.
Lessons from Sickle Cell Anemia Research
The research surrounding sickle cell anemia has yielded critical lessons that extend beyond the disorder itself. One key takeaway is the importance of genetic research in understanding disease mechanisms. The detailed study of hemoglobin mutations not only aids in the comprehension of sickle cell anemia but also provides a framework for exploring other genetic conditions.
Furthermore, sickle cell research has illustrated how advancements in gene therapy and editing technologies such as CRISPR could potentially transform treatment options. These methodologies hold promise for correcting the faulty gene responsible for the disease, which may lead to curative approaches in the future.
Another valuable lesson is the importance of community engagement in research. Patients’ and families' input is crucial for shaping studies that genuinely address their needs and experiences. As a result, initiatives that involve patients in the research process are likely to produce more relevant and impactful outcomes.
Additionally, sickle cell anemia has highlighted the necessity of international collaboration in public health. Countries affected by the disease often share similar challenges. Therefore, sharing knowledge and resources can enhance diagnostic and treatment capabilities globally.
In summary, the insights drawn from the study of sickle cell anemia contribute to a deeper understanding of genetic disorders and their treatment. These learnings offer direction for future research endeavors and public health policies aiming to address genetic conditions holistically.
Closure
The conclusion of this article serves as a pivotal moment for synthesizing the multitude of aspects surrounding sickle cell anemia. Sickle cell anemia, while a specific genetic disorder, opens a wider dialogue on the implications of genetic mutations. Having explored the underlying DNA mutation that results in altered hemoglobin, it becomes evident how such a seemingly small change—specifically a single nucleotide substitution—can have significant implications for an individual’s health.
Summarizing Key Points
In summary, the DNA mutation responsible for sickle cell anemia highlights aspects of genetics, pathology, and treatment.
- Mutation Specifics: The mutation leads to the production of abnormal hemoglobin, known as hemoglobin S. This leads to distorted red blood cell shapes, which affects circulation and causes various complications.
- Genetic Inheritance: Understanding the autosomal recessive inheritance pattern is crucial. This means that both parent must pass on the defective gene for a child to be affected.
- Healthcare Impact: Clinically manifesting symptoms include pain crises and increased susceptibility to infections, both of which have broad implications for healthcare strategies and resource allocation.
This concise re-statement emphasizes the critical topics covered and establishes a clear connection between genetic understanding and its clinical significance.
Implications for Future Research
The implications of research on sickle cell anemia extend beyond immediate clinical management. They shape future investigations in several key areas:
- Gene Therapy: Ongoing research aims to develop gene editing techniques like CRISPR to correct the genetic defect at its source. This represents a promising frontier.
- Drug Development: Studies continue into pharmacological solutions that can manage symptoms more effectively or potentially reverse the disease process by targeting hemoglobin S.
- Public Health Initiatives: Improved screening methods and awareness programs can help identify at-risk populations, thereby enhancing early interventions.
With continued advancements in genomics and molecular biology, it is crucial to expedite research efforts. The lessons learned from sickle cell anemia can further inform genetic research and treatment modalities for other genetic disorders. As the understanding of DNA mutations evolves, the promise of better therapeutic strategies becomes an achievable goal, underscoring the importance of ongoing inquiry into this genetic condition.