Chromatin Remodeling: Mechanisms and Cellular Impact
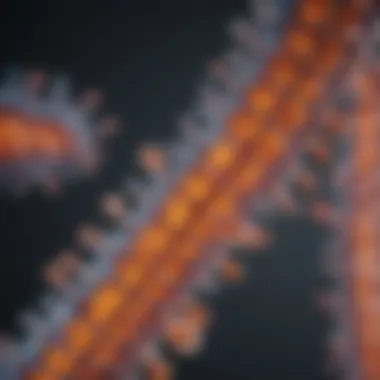
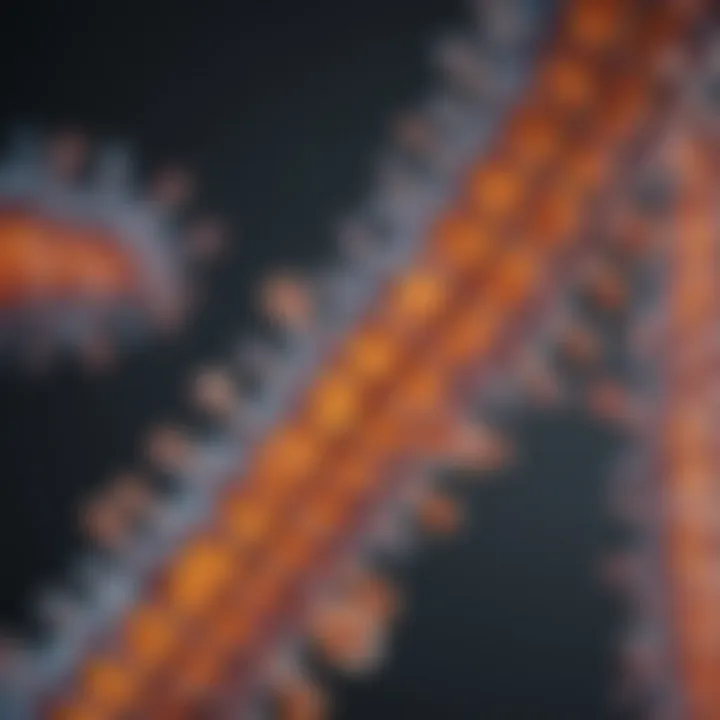
Intro
In the grand tapestry of cellular biology, the regulation of gene expression plays a pivotal role in determining how cells respond to various stimuli and execute their functions. A critical player in this arena is chromatin remodeling—a complex and dynamic process that alters the structure and accessibility of DNA. The significance of chromatin remodeling cannot be overstated, as it underpins essential cellular functions like gene activation, silencing, and DNA repair. This intricately woven narrative explores the mechanisms behind chromatin remodeling, its various complexes, and their implications across different biological contexts.
Recent studies have shone a light on the vital role of chromatin dynamics not merely in development but also in various diseases. These findings underline the importance of understanding how disturbances in this process can lead to pathological conditions. By examining chromatin remodeling from multiple perspectives—mechanisms, implications, and research methodologies—we aim to provide a comprehensive overview that caters to students, researchers, educators, and professionals alike. The journey into the realm of chromatin remodeling will illuminate its intricacies and shed light on its profound impact on cellular functions.
Methodologies
Delving into the science behind chromatin remodeling involves a variety of research techniques and tools that have been refined over the years. Understanding the tools and methodologies used in this field can offer profound insights into how chromatin structure is manipulated and regulated.
Description of Research Techniques
- Chromatin Immunoprecipitation (ChIP): This technique is commonly used to study protein-DNA interactions. By using specific antibodies, researchers can isolate portions of the chromatin that are bound by proteins, allowing for the identification of modifications and their functional relevance.
- Next Generation Sequencing (NGS): This technology has revolutionized our understanding of chromatin remodeling by providing high-throughput sequencing capabilities. NGS enables researchers to analyze the entire genome’s chromatin landscape and observe how it varies under different conditions.
- ATAC-Seq (Assay for Transposase-Accessible Chromatin with high-throughput sequencing): This is a powerful method to evaluate chromatin accessibility across the genome. By using a transposase enzyme, ATAC-Seq identifies regions of open chromatin, shedding light on active regulatory elements related to gene expression.
Tools and Technologies Used
Research in this area relies heavily on various technologies. Some prominent tools include:
- CRISPR/Cas9 Systems: Widely recognized for its gene-editing capabilities, CRISPR can also be applied in chromatin studies to manipulate chromatin architectures intentionally.
- Fluorescence In Situ Hybridization (FISH): This method allows researchers to visualize specific DNA sequences in the context of chromatin, helping to correlate structure and function within the nucleus.
- Mass Spectrometry: Employed for identifying post-translational modifications on histones, mass spectrometry provides insight into how chromatin remodeling complexes modify histone proteins.
Understanding these methodologies is crucial, as they provide a frame of reference for evaluating the chromatin landscape's complexities and their repercussions in cellular function.
Discussion
The ongoing discourse around chromatin remodeling brings to mind previous research efforts that have laid the groundwork for present-day studies. One of the key aspects of discussing chromatin remodeling is to highlight how modern techniques have improved upon traditional methods.
Comparison with Previous Research
In the earlier days, studying chromatin was akin to peering through a foggy window. The advent of high-resolution techniques like ChIP and NGS has clarified much of the haziness. Previous literature relied more heavily on indirect measures of chromatin structure and function, but the ability to directly observe changes introduces a new level of precision.
Theoretical Implications
The implications of chromatin remodeling stretch far beyond mere cellular housekeeping. They raise intriguing questions about genetic regulation, developmental biology, and disease mechanisms. For instance, the intricate dance between accessibility and compaction of chromatin could provide clues into how certain diseases, such as cancer, exploit these mechanisms to promote tumorigenesis.
Moreover, understanding the chromatin landscape is crucial for advancing therapeutic strategies. Modulating chromatin remodeling processes may reveal new avenues for drug development and treatment protocols, particularly in diseases where genetic regulation goes awry.
The exploration of chromatin dynamics offers invaluable insights that link cellular function with broader biological phenomena, underlining its significance in both health and disease.
In essence, the journey through chromatin remodeling marks a fascinating intersection of molecular biology and therapeutic advancement, providing depth and context for ongoing studies in this vital area of research.
Preamble to Chromatin Remodeling
Understanding how chromatin remodeling works is like putting together a complex puzzle. Each piece, from DNA and histones to the proteins that assist in modifying chromatin, plays an integral role. This introduction provides insight into why chromatin remodeling is crucial for gene expression, DNA repair, and even the cellular response to environmental changes. When we grasp the mechanisms behind chromatin dynamics, we can start to connect the dots between cellular functions and the many biological processes that follow.
Definition and Significance
Chromatin remodeling refers to the reshaping of chromatin structure to allow access to the underlying DNA for various cellular functions. Simply put, if chromatin is the tightly wrapped packaging of DNA, chromatin remodeling is the act of loosening that package so that the cell can read and utilize the genetic instructions contained within.
The significance of chromatin remodeling transcends mere structural changes. It's essential for regulating gene expression, determining how genes are turned on or off in response to various stimuli. For example, when a cell receives signals to grow, chromatin remodeling mechanisms act instantaneously, remodeling the chromatin to make specific genes accessible for transcription. This process not only ensures that genes are expressed when needed but also prevents errors that may lead to diseases such as cancer. Thus, understanding this mechanism aids in grasping not just general biology but also the underpinnings of many pathologies.
Historical Perspective
Chromatin remodeling is not a recent discovery; its exploration spans decades. In the early days of molecular biology, the focus was primarily on DNA sequences, but as the field evolved, researchers began to recognize the importance of chromatin structure.
The initial studies in the 1970s and 1980s identified histones as key players in DNA packaging. As more advanced techniques emerged, such as chromatin immunoprecipitation, scientists could observe chromatin dynamics in real-time, opening up new avenues of research. Notably, the early 2000s marked a pivotal point when various chromatin remodeling complexes, like the SWI/SNF complex, were discovered, underscoring the diverse and essential roles these components play. Today, we stand at the forefront of chromatin research, diving deeper into how these complexes interact, influence cellular fates, and potentially provide therapeutic targets for diseases.
"The ability of cells to modulate chromatin structure is a key determinant in both normal development and the pathogenesis of diseases."
In essence, the journey of chromatin remodeling research illustrates an evolving understanding of life at the molecular level. By continuing to explore this field, we can uncover more intricate layers of how cells operate, adapt, and respond to their environment.
The Structure of Chromatin
Understanding the structure of chromatin is essential for appreciating its fundamental role in cellular function. Chromatin's unique architecture, comprising DNA and proteins, is not just a passive backdrop; rather, it actively participates in the regulation of gene expression, DNA replication, and repair. By delving into the specific components and organization of chromatin, one can grasp how these elements contribute to cellular activities and respond to various stimuli, whether during development or in the context of disease.
Components of Chromatin
Chromatin primarily consists of DNA wrapped around proteins known as histones. Here’s a closer look at these elements:
- DNA: The genetic material that contains the instructions for the development and function of living organisms. The winding of DNA around histones is crucial for fitting it into the nucleus.
- Histones: These are small proteins that help condense DNA into a compact form called nucleosomes. There are five main types of histones – , A, B, , and . Each type serves specific roles in maintaining the structural integrity and functionality of chromatin.
- Non-Histone Proteins: Aside from histones, various other proteins are involved in the dynamic processes of chromatin remodeling. These include transcription factors and chromatin remodeling complexes that facilitate the accessibility of DNA to regulatory proteins, impacting gene expression.
- Non-Coding RNAs: Recent discoveries highlight the role of non-coding RNAs in chromatin structure. These molecules can influence histone modification and chromatin arrangement, painting a complex picture of gene regulation beyond traditional coding sequences.
The interplay of these components determines not only the structural organization of chromatin but also its functional capabilities, making it a focal point of research in cellular biology.
Nucleosome Organization
Nucleosomes are considered the fundamental units of chromatin structure. Each nucleosome consists of a segment of DNA coiled around a core of histone proteins, resembling "beads on a string" when visualized.
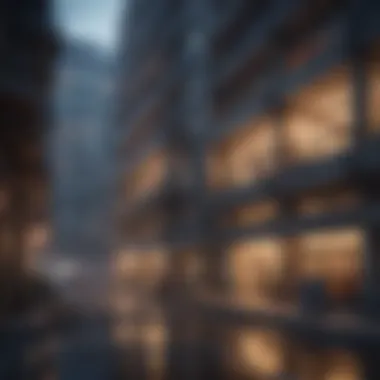
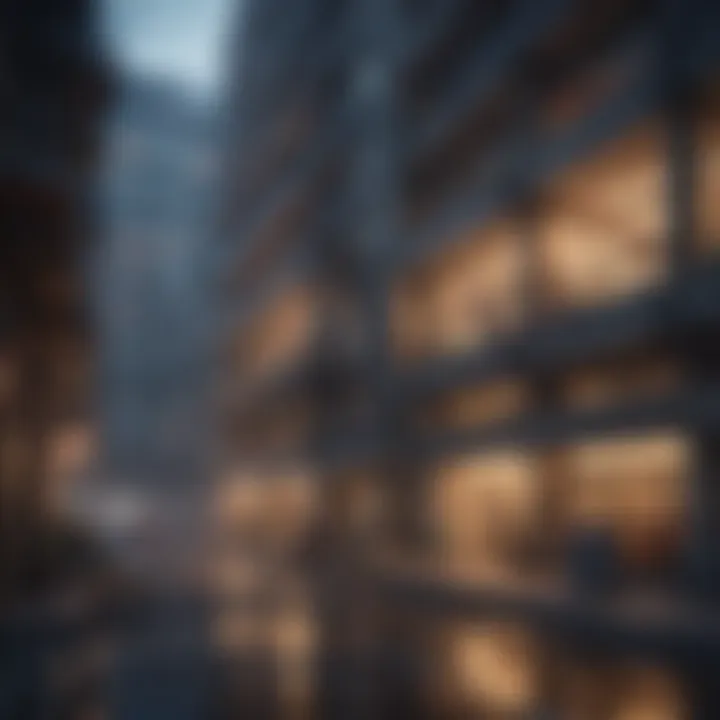
- Formation of Nucleosomes: The process involves approximately 146 base pairs of DNA wrapped around an octamer of histone proteins (two each of A, B, , and ). This configuration serves as a primary level of DNA packaging, allowing for considerable compaction within the nucleus.
- **Histone **: This linker histone binds to the nucleosome and helps facilitate the formation of higher-order structures, enabling further condensation of the DNA. ’s role is key in regulating nucleosome spacing and overall chromatin structure.
- Higher-Order Structuring: Nucleosomes are further organized into fibers, commonly referred to as chromatin fibers, which exist in two states: euchromatin and heterochromatin. Euchromatin is less condensed and generally associated with active gene transcription. In contrast, heterochromatin is tightly packed, often silencing gene expression.
A greater understanding of nucleosome organization clarifies how chromatin can dynamically transition between these states, which profoundly influences gene activity and cellular responses.
"Nucleosomes serve as crucial sites where DNA accessibility and gene regulation occur, making them pivotal in the orchestration of cellular activities."
The study of chromatin structure is not just an academic exercise; it has far-reaching implications in biotechnology, medicine, and the understanding of complex biological systems.
Mechanisms of Chromatin Remodeling
Understanding the mechanisms behind chromatin remodeling is crucial for grasping how genetic information is regulated within a cell. These processes ensure that the DNA can be accessed when needed, impacting gene expression and cellular function. Chromatin remodeling is not just a behind-the-scenes act; it plays a starring role in various cellular activities, allowing cells to adapt to changing environments and maintain homeostasis.
ATP-Dependent Remodeling Complexes
ATP-dependent remodeling complexes act like the machinery of chromatin, using energy derived from ATP to reposition nucleosomes on DNA. This repositioning can control access to specific genes, thus affecting their expression. Among the most notable complexes are the SWI/SNF and ISWI families, each with respective enzymes that facilitate DNA accessibility and transcriptional regulation.
The importance of these complexes lies in their ability to dynamically alter chromatin structure. This capability means they can open up or pack down chromatin based on cellular needs, making them vital players in developmental processes and responses to external cues.
"In the game of gene regulation, ATP-powered machines hold the keys to unlock or lock the doors to genomic information."
Histone Modifications
Histone modifications are another critical component of chromatin remodeling. Changes to the chemical structure of histones—proteins around which DNA winds—can either promote or inhibit gene expression. The types of modifications include acetylation, methylation, and phosphorylation, and each plays a unique role in shaping chromatin structure and function.
Acetylation
Acetylation generally promotes a more open chromatin structure. This process involves adding an acetyl group to histones, effectively neutralizing their positive charge. The result is a relaxed chromatin state, which is more accessible to transcription factors and other regulatory proteins. Acetylation is often seen as a desirable modification because it typically correlates with active gene expression.
The unique feature of acetylation is its reversible nature. Enzymes known as histone deacetylases can remove acetyl groups, allowing for fine-tuned regulation of transcriptional activity. However, excessive acetylation can lead to issues in gene regulation, making the balance crucial.
Methylation
Methylation has a more complex role, as its effects can vary depending on the location and number of methyl groups added. Typically, it is associated with transcriptional repression when methyl groups are added to specific lysine residues on histones. However, some methyl modifications can also promote gene activity, illustrating the duality of this mechanism.
Methylation's notable characteristic is its stability; once a methyl group is attached, it tends to remain throughout cellular divisions, effectively serving as a cellular memory. This stability is beneficial for maintaining gene expression patterns during development. On the downside, aberrant methylation patterns are frequently linked to various diseases, highlighting the importance of precise control in this area.
Phosphorylation
Phosphorylation, involving the addition of phosphate groups to histones, plays a pivotal role in processes like DNA damage repair and transcriptional activation. This modification can alter protein interactions and behavior and is often associated with signaling pathways that respond to environmental changes.
The key characteristic of phosphorylation is its temporal nature. Unlike other modifications, it often occurs in response to cellular signals, making it a rapid means of regulating gene expression. While broadly advantageous, inappropriate or excessive phosphorylation can disrupt normal cellular functions, leading to risks such as unchecked cellular growth.
Non-Coding RNAs in Chromatin Dynamics
Non-coding RNAs, including microRNAs and long non-coding RNAs, have emerged as significant players in chromatin dynamics, influencing the structure and modifications of chromatin. They can guide specific histone modifications by recruiting various chromatin remodeling complexes, serving as regulatory molecules that dictate the nuclear landscape.
As we delve into the role of non-coding RNAs in chromatin dynamics, their influence extends beyond mere structural changes. They participate in gene silencing and activation, providing another layer of regulatory control. By modifying chromatin accessibility, non-coding RNAs help ensure that genes are expressed only when necessary, reflecting the cell's environmental context.
In summary, navigating the mechanisms of chromatin remodeling reveals a landscape rich with intricate interactions and dynamic processes that underpin gene regulation, cellular responses, and ultimately, organismal development.
Chromatin Remodeling During Development
Chromatin remodeling acts as a pivotal player during the development of multicellular organisms. It's akin to the conductor of an orchestra, ensuring each section plays its part harmoniously. The complexity of development hinges on the ability of chromatin to package DNA yet allow accessibility to specific regions when needed. This process not only orchestrates when genes are turned on or off but also tailors cellular functions to the unique requirements of different tissues.
Role in Embryogenesis
During early embryogenesis, chromatin remodeling plays a crucial role in establishing the groundwork for future cellular fate. From the zygote onward, the genome undergoes tremendous changes as it must rapidly respond to a myriad of signals. The architecture of chromatin, influenced by remodeling complexes, facilitates the transition from a fertilized egg to a fully developed organism.
"In the dance of embryogenesis, chromatin acts as the flexible stage, adapting to the needs of each performance."
Each cell must remember when its lineage diverges, guide developmental processes, and clear paths for transcription. Key complexes such as SWI/SNF and ISWI reshape chromatin, ensuring necessary genes are accessible when needed. Moreover, the dynamic nature of histone modifications during these early phases is crucial. These modifications, including phosphorylation and acetylation, mark specific genes, effectively serving as flags indicating which pathways will be pursued as the embryo develops.
Cellular Differentiation
As embryonic development unfolds, cellular differentiation becomes essential. Here, chromatin remodeling ensures that stem cells can give rise to various specialized cell types. For instance, a stem cell destined to be a neuron must silence genes meant for a muscle cell, and this is where the magic of chromatin modulation shines.
The interplay between histone modifications and DNA methylation forms a complex regulatory network. This network ensures that once a cell commits to a specific lineage, the necessary genes are activated while the extraneous ones are repressed. Such precision underscores the importance of chromatin remodeling in guiding not only which genes are expressed, but also how they are expressed.
- Key points regarding chromatin remodeling during cellular differentiation include:
- Accessibility of specific gene regions.
- Dynamic histone modifications that signal gene activation or repression.
- The adaptability of chromatin structure to environmental cues, influencing cell fate decisions.
Overall, the synergy of chromatin remodeling during development ensures that cells appropriately respond to their intrinsic and extrinsic signals, ultimately determining their function and fate within the organism.
Chromatin Remodeling and Gene Expression
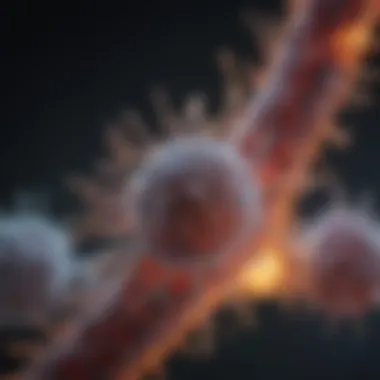
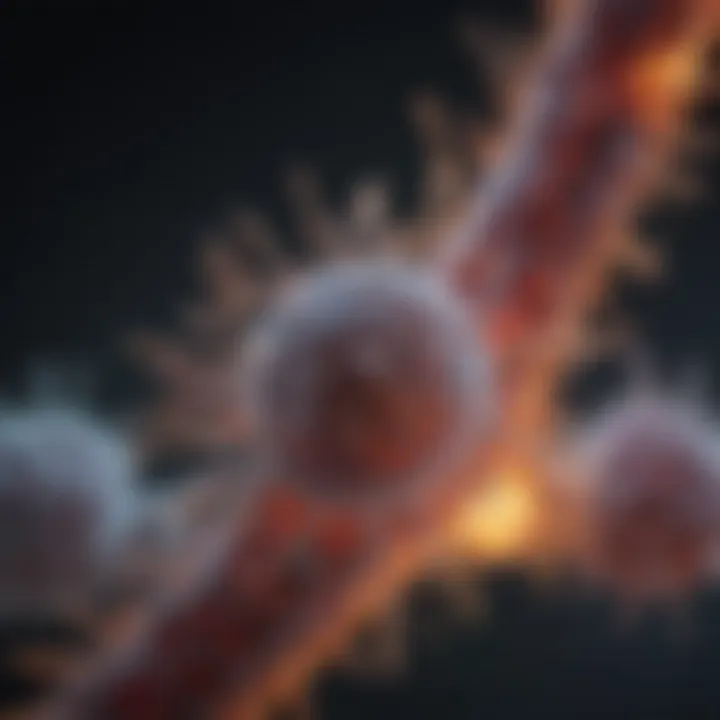
The intricate relationship between chromatin remodeling and gene expression is a cornerstone in understanding cellular functions. Chromatin, the complex of DNA and protein, is not merely structural; it plays a dynamic role in regulating access to genetic information. In simpler terms, think of chromatin as a library where genes are books. The process of chromatin remodeling shifts these books around, making some easy to find while others remain tucked away. This intricate dance directly impacts which genes are active or silenced at any given moment.
The importance of chromatin remodeling in gene expression lies in several key elements:
- Accessibility of DNA: Chromatin can be tightly packed or loosely organized. When it's tightly packed, transcription machinery cannot access the DNA to initiate gene expression. Remodelers facilitate this access.
- Regulatory Mechanisms: Various signaling pathways interact with chromatin remodelers to communicate cellular needs. For example, during stress, certain genes may need quick activation, requiring rapid chromatin reconfiguration.
- Epigenetic Modifications: Modifications like methylation or acetylation that occur during chromatin remodeling affect gene expression outcomes, providing a long-term influence that goes beyond immediate cellular responses.
In addition to these factors, understanding the implications of chromatin remodeling on gene expression can offer insights into various biological processes, including development and adaptation to environmental changes.
Transcriptional Activation
Transcriptional activation is a fascinating aspect of how cells control gene expression. It involves changing the chromatin structure so that specific genes can be more accessible to transcription factors and RNA polymerase. Think of this as rolling up a thick curtain that lets the sunlight in—when chromatin remodeling occurs, the light of gene expression shines through.
Several players are involved here:
- Activator Proteins: These proteins bind to specific DNA sequences and recruit chromatin remodelers, setting the stage for transcription.
- Histone Acetylation: Enzymes known as histone acetyltransferases add acetyl groups to histones, loosening their grip on DNA. This action opens up the chromatin structure, encouraging transcriptional activity.
- Chromatin Remodeling Complexes: These molecular machines shift nucleosomes—the fundamental units of chromatin—to make the DNA more accessible for transcription.
During this dance of activation, chromatin dynamics entangle with various signaling networks, allowing cells to quickly adapt gene expression in response to internal and external cues.
Transcriptional Repression
On the flip side, transcriptional repression is essential for controlling gene expression. It ensures that unnecessary genes remain silent, maintaining cellular efficiency and identity. Imagine a librarian who knows when to close the books on topics that are not currently relevant. Similarly, chromatin remodeling can be directed to compact the chromatin structure to prevent unwanted transcription.
Key factors in transcriptional repression include:
- Repressor Proteins: These proteins can bind to DNA and actively recruit repressive chromatin remodeling complexes.
- Histone Methylation: The addition of methyl groups to histones can lead to tighter wrapping of DNA, making transcription machinery unable to access genes. This is often a mark of gene silencing.
- Non-Coding RNAs: These molecules contribute to transcriptional repression by guiding chromatin-modifying complexes to specific genomic regions.
Repression is often just as nuanced as activation, balancing the cellular needs and ensuring that pathways not needed at any point do not distract from the primary focus of the cell.
"The way genes are expressed isn't just about what's in the DNA, but about how accessible that DNA is for transcription—a story of remodeling, restructuring, and regulation."
In sum, understanding how chromatin remodeling influences both transcriptional activation and repression provides crucial insights into how cells function, adapt, and evolve in response to their internal and external environments. These processes are vital not only for normal cellular physiology but also play significant roles in various diseases, making this area of research increasingly critical.
Impact of Chromatin Remodeling on Cellular Processes
Chromatin remodeling serves as a cornerstone in maintaining not just the integrity of the genome, but also in orchestrating numerous cellular activities. Given its dynamic nature, chromatin plays an instrumental role in regulating processes like gene expression, DNA repair, and overall cellular health. By understanding how chromatin remodeling impacts these cellular processes, we can better grasp the underlying mechanisms that govern cell function and respond to both internal and external stimuli.
One important aspect of chromatin remodeling is its influence on DNA repair mechanisms. When DNA strands become damaged due to factors such as environmental stress or replication errors, timely repair is crucial. Chromatin remodeling acts as the gatekeeper, facilitating accessibility to the repair machinery. This involves altering nucleosome positioning to provide the required space for repair proteins to do their job. Failing to properly remodel chromatin can lead to accumulation of mutations, contributing to various diseases, including cancers.
Furthermore, chromatin remodeling also plays a significant role in cell cycle regulation. The cell cycle is a tightly coordinated series of events that lead to cell division and replication. Specific phases of this cycle necessitate precise adjustments in chromatin structure for proper gene expression. For instance, during the transition from G1 to S phase, chromatin remodeling complexes are recruited to initiate DNA replication. Without this remodeling, cells may either replicate abnormally or fail to divide altogether.
In summary, the impacts of chromatin remodeling on cellular processes are profound and far-reaching. By facilitating DNA repair and regulating the cell cycle, chromatin remodeling not only ensures the preservation of genomic integrity but also underpins healthy cellular function.
"The intricate dance of chromatin proteins is fundamental to how cells adapt and respond to their environment, showcasing the delicate balance between function and structure."
DNA Repair Mechanisms
Chromatin remodeling is pivotal in DNA repair mechanisms. When DNA experiences breaks or other forms of damage, chromatin undergoes structural changes that enable nucleases and repair proteins to access the affected areas. The recruitment of ATP-dependent chromatin remodeling complexes, such as the SWI/SNF family, ensures that these critical sites are swiftly and effectively addressed.
Several key elements are involved in the process:
- Histone modifications, such as phosphorylation and ubiquitination, serve as signals for the recruitment of repair factors.
- Chromatin structural changes provide access to the exposed DNA, allowing repair complexes to function effectively.
- Non-coding RNAs also play a role in orchestrating these repair activities, acting as regulators of chromatin dynamics.
Overall, effective chromatin remodeling is essential for robust DNA repair processes, ensuring cells can maintain fidelity and prevent malignancy.
Cell Cycle Regulation
Cell cycle regulation is yet another area greatly influenced by chromatin remodeling. During different phases of the cell cycle, chromatin must take on different configurations to facilitate or hinder gene expression. For example, prior to DNA synthesis in the S phase, chromatin transitions to a more relaxed state, which allows the necessary genes for DNA replication to be expressed.
Key factors include:
- Cyclins and cyclin-dependent kinases (CDKs) work in conjunction with chromatin remodelers to transition between phases.
- Euchromatin versus heterochromatin states modulate access to replication origins during S phase.
- Checkpoint proteins monitor chromatin status to prevent cells from unwarranted division, maintaining cellular integrity.
Chromatin Remodeling in Disease
Chromatin remodeling plays a critical role in numerous diseases, highlighting its significance well beyond basic cellular function. Understanding how chromatin dynamics can influence disease pathways unveils a new realm of therapeutic avenues. Chromatin, the protein-DNA complex, isn't just a structural entity; it is a dynamic player, whose alterations can have profound implications in diverse disorders like cancer and neurological diseases.
Cancer Biology
Cancer represents an uncontrolled alteration of cell growth, often linked to changes in gene expression that stem from disrupted chromatin remodeling. The role of chromatin remodeling factors in tumorigenesis cannot be underestimated. When the normal function of these factors is compromised, gene silencing or activation can lead to the expression of oncogenes or the silencing of tumor suppressor genes.
Some key points include:
- Mutated Chromatin Remodelers: Certain mutations in chromatin remodeling genes such as SWI/SNF complexes have been found in various cancers. These mutations lead to alterations in tumorigenic pathways, which can worsen the disease prognosis.
- Therapeutic Targets: Understanding the specific chromatin alterations in individual tumors paves the way for personalized medicine approaches, targeting the chromatin landscape directly. For instance, the targeting of BRG1, a component of the SWI/SNF complex, has shown promise in preclinical studies for a range of cancers.
- Epigenetic Regulation: In the context of cancer, epigenetic modifications, such as DNA methylation and histone modifications, play pivotal roles. Abnormal patterns of these modifications can lead to gene expression changes typical of cancer cells, enhancing aggressive behavior and resistance to therapies.
"The pervasive role of chromatin remodeling in cancer underscores the need for deeper investigations to delineate its pathways and interactions."
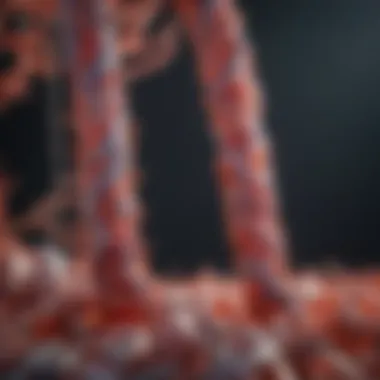
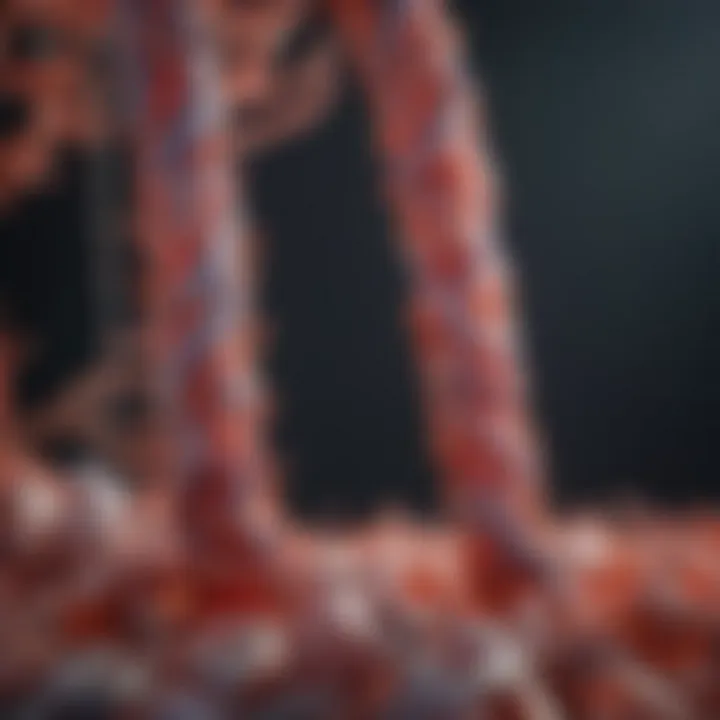
Neurological Disorders
Neurological disorders are another realm where chromatin remodeling exhibits significant implications. The brain is particularly sensitive to changes in chromatin structure because of its complex function and regulation. Anomalies in chromatin dynamics are implicated in neurodevelopmental disorders, neurodegeneration, and various psychiatric disorders.
Several notable aspects include:
- Developmental Implications: Disorders like autism spectrum disorders (ASD) have been associated with mutations in chromatin remodeling genes. These alterations affect brain development and can lead to long-lasting cognitive and behavioral differences.
- Synaptic Function: Chromatin remodeling influences the expression of genes crucial for synaptic plasticity, which is fundamental in learning and memory. Disruption in these processes may contribute to conditions like Alzheimer's disease.
- Drug Development: Just as in cancer, the investigation into chromatin dynamics opens doors for intervention strategies. Histone deacetylase inhibitors, for instance, have shown potential in enhancing cognitive function in some animal models of cognitive impairment.
In summary, chromatin remodeling emerges as a critical player in both cancer biology and neurological disorders, presenting itself as a compelling target for therapeutic intervention. Understanding its intricacies enables researchers to devise strategies that could potentially ameliorate or, better yet, prevent these diseases.
Chromatin Remodeling: Therapeutic Perspectives
Chromatin remodeling has emerged as a pivotal area of interest in therapeutic development, shedding light on how altering chromatin structure can influence gene activity and cellular behavior. Understanding these processes opens the door for novel strategies to tackle various diseases, particularly those stemming from aberrant gene regulation. By focusing on the nuances of chromatin dynamics, researchers are starting to tap into the potential of therapies that target chromatin remodeling mechanisms.
Targeting Remodeling Complexes
Chromatin remodeling complexes play a central role in modifying the structure of chromatin, thereby regulating access to DNA. These complexes utilize energy derived from ATP hydrolysis to reposition, eject, or restructure nucleosomes. By targeting these remodeling complexes, we can develop therapies aimed at correcting dysfunctions in gene expression associated with numerous diseases.
For example, some cancers have been linked to mutations in the components of chromatin remodeling complexes. Inhibiting the activity of specific complexes could restore proper gene expression patterns. Researchers have identified key complexes, such as the SWI/SNF family, which is involved in maintaining tumor suppressor functions. Understanding how these complexes operate provides insights into developing small molecules or biologics that can modulate their activity.
Additionally, approaches that inhibit excessive remodeling can foster an environment conducive to normal cellular function.
- Potential Strategies:
- Small molecules that inhibit ATPase activity in chromatin remodeling machineries.
- Gene editing technologies like CRISPR to restore normal remodeling complex function in diseased cells.
As we delve into targeting remodeling complexes, the challenge remains in selectively modulating these complexes without disrupting their essential roles in other cellular processes.
Histone Modifiers as Therapeutics
Histones are crucial proteins around which DNA winds, and their chemical modifications are fundamental in controlling chromatin accessibility and, subsequently, gene expression. These modifications, such as acetylation, methylation, or phosphorylation, can either promote or repress transcription depending on the context. Thus, histone modifiers represent a valuable avenue for therapeutic intervention.
Therapeutic agents that mimic or inhibit histone modifications have shown promise in preclinical models. For instance, inhibitors of histone deacetylases (HDACs) are used in some cancer treatments to promote the acetylation of histones, leading to a more open chromatin structure conducive to gene activation. This method highlights the potential for reviving beneficial gene expression profiles silenced in disease states.
Moreover, the use of histone methyltransferase inhibitors is gaining traction in specific contexts of cancer therapy, particularly in cases with known histone methylation abnormalities. By addressing these specific modifications, treatments might correct gene silencing associated with tumor growth.
"Modifying the modifiers—histone acetylation and methylation—can give researchers a foothold in the therapeutic realm of gene regulation."
Potential therapeutic strategies might include:
- Histone Acetyltransferase (HAT) Activators: Enhancing HAT activity to promote gene expression.
- Selective Inhibitors of Histone Methyltransferases: Targeting excess methylation states linked to gene silencing.
Future Directions in Chromatin Research
Understanding the complexity of chromatin remodeling is crucial for advancing our knowledge of cellular mechanisms. In contemporary biology, the exploration of chromatin dynamics is not just an academic exercise; it has practical implications for health and disease. By delving into future directions in chromatin research, we can uncover new therapeutic avenues and enhance our understanding of cellular behavior.
Emerging Technologies
The field of chromatin research stands on the brink of significant transformation, largely driven by emerging technologies. High-throughput sequencing techniques, such as ATAC-seq (Assay for Transposase-Accessible Chromatin using sequencing), allow researchers to analyze the accessibility of chromatin regions across the genome with remarkable precision. This method provides a snapshot of chromatin states in vivo and can reveal insights about regulatory elements that govern gene expression.
Another promising advancement is the integration of CRISPR-based methodologies. CRISPR tools can be designed to target specific chromatin regions, enabling scientists to investigate the effects of chromatin modifications on gene regulation directly. By employing synthetically engineered strategies, researchers can manipulate histone marks and other modifications, a feat that could redefine our understanding of gene expression fidelity.
Finally, advancements in imaging technologies, like super-resolution microscopy, have revolutionized the visualization of chromatin in its native environment. These tools allow scientists to observe chromatin behavior in live cells, paving the way for deeper insights into the dynamic interactions among chromatin, transcription machinery, and other nucleoproteins.
Understanding Complex Interactions
In the quest to dissect chromatin remodeling further, understanding complex interactions between various biomolecules is crucial. Chromatin does not exist in isolation; it interacts constantly with proteins, RNAs, and other cellular components. Therefore, a future direction in research must also focus on the interplay between chromatin and other elements within the cell.
For instance, the role of non-coding RNAs, such as long non-coding RNAs (lncRNAs), in chromatin structure and function remains a hot topic. These molecules have been implicated in recruiting remodeling complexes to specific chromatin sites, thereby influencing gene expression patterns. A more nuanced understanding of these interactions could lead to breakthroughs in elucidating the underlying mechanisms of diseases, including cancer and genetic disorders.
Moreover, the study of metabolic states and their effects on chromatin dynamics is another fertile area for investigation. Metabolites can modify histones and influence chromatin accessibility. Understanding this connection could reveal how metabolic dysregulation contributes to pathologies.
Emerging frameworks that integrate various layers of biological information—such as genomics, proteomics, and metabolomics—will be critical in configuring a comprehensive view of chromatin remodeling mechanisms. By navigating this intricate web of interactions, researchers stand to not just enhance basic science but also provide significant insights for therapeutic applications.
Future breakthroughs in chromatin research hinge on innovative technologies and understanding the complex interplay of cellular factors, shaping both fundamental biology and potential therapeutic innovations.
Epilogue
The conclusion of this article brings to the forefront the significance of chromatin remodeling in cellular functions, underscoring its pivotal role in various biological processes. Chromatin remodeling not only affects gene expression but also plays a crucial part in DNA repair, cellular differentiation, and the response to environmental changes. Understanding these processes allows researchers and practitioners to appreciate the delicate balance within cellular mechanisms—where misregulation can lead to significant health issues, including cancer and neurological disorders.
Summary of Key Concepts
In wrapping things up, it's important to revisit the main points covered throughout the article. The central themes of chromatin remodeling include:
- Chromatin Structure: Understanding the fundamental composition of chromatin and how it organizes into nucleosomes is crucial for grasping the dynamics of gene regulation.
- Mechanisms: ATP-dependent remodeling complexes and histone modifications are the two primary mechanisms, highlighting the intricate ways cells adapt to their needs.
- Developmental Roles: The remodeling is vital in embryogenesis and cellular differentiation, helping to shape organism development from a single cell.
- Disease Implications: Missteps in chromatin dynamics can lead to degenerative diseases and cancers, making the study of these processes essential for future therapies.
- Therapeutic Targets: By targeting specific remodeling complexes and histone modifiers, we can explore new avenues in treatment approaches for diseases linked to chromatin dysregulation.
"Understanding chromatin remodeling is key for recognizing how cellular processes can go awry and lead to disease."
Implications for Future Research
Looking ahead, there are several exciting directions for future research in chromatin remodeling:
- Novel Technologies: Advancements in genome editing and imaging technologies offer promising tools for dissecting chromatin dynamics in live cells. High-resolution techniques could reveal real-time alterations in chromatin structure during processes such as transcription and repair.
- Broader Biological Context: Exploring chromatin remodeling's role across different organisms and conditions can shed light on evolutionarily conserved mechanisms and unique adaptations.
- Complex Interactions: There is a need to understand the interplay between chromatin remodeling, non-coding RNAs, and other cellular processes. This holistic view may unveil unknown regulatory pathways critical for maintaining cellular health.
- Therapeutic Applications: As treatment for diseases linked to chromatin aberrations becomes a focus, identifying specific inhibitors or activators that precisely target remodeling complexes could revolutionize therapeutic strategies.