In-Depth Analysis of cDNA Libraries in Molecular Biology
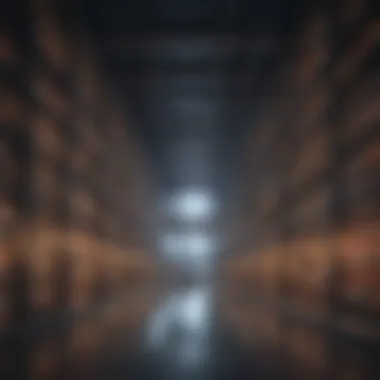
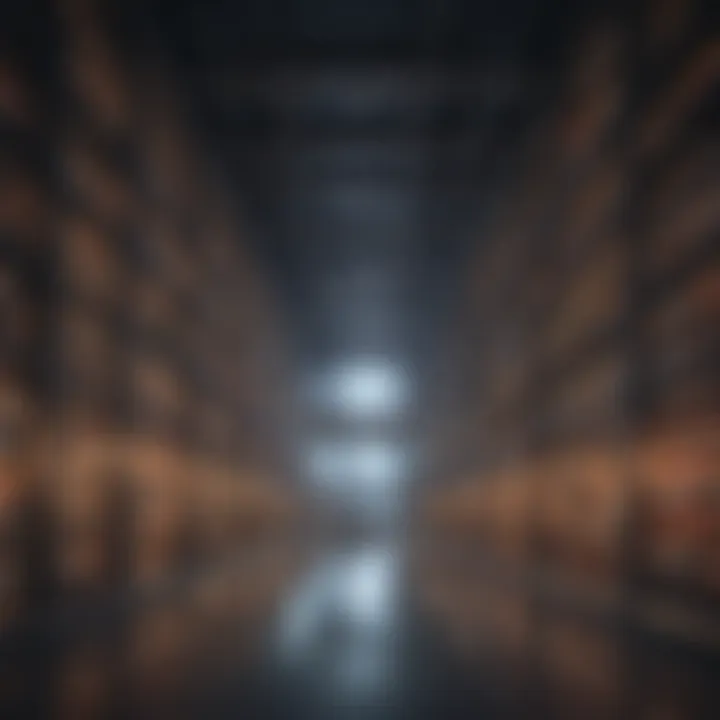
Intro
In the realm of molecular biology, complementary DNA (cDNA) libraries serve as a powerhouse of information. These libraries, which consist of blends of DNA that are complementary to the mRNA expressed in a particular tissue at a given time, are pivotal for understanding gene expression and functionality. As research in genetics and genomics accelerates, the necessity for these libraries cannot be understated. From elucidating the complexities of gene interactions to facilitating drug discovery, cDNA libraries hold the key to numerous advancements in life sciences. In this exploration, we will delve into how these libraries are constructed, their pivotal applications, and the challenges faced by researchers in this intricate field.
Methodologies
Description of Research Techniques
Crafting a cDNA library involves a series of meticulous steps that ensure the accurate representation of mRNA populations. The first pivotal step is the isolation of mRNA from the desired cells or tissues. Once the mRNA is obtained, it undergoes the reverse transcription process which converts the mRNA into cDNA using the enzyme reverse transcriptase. This enzyme effectively synthesizes complementary DNA strands that mirror the mRNA sequence, creating a pool of cDNA.
But that's not where the story ends. The cDNA then needs to be amplified and cloned into vectors, which can successfully harbor the DNA for future analyses. This often employs cloning techniques such as the use of plasmids or phages. Each of these choices can significantly impact the performance of the library in downstream applications, from sequencing to expression studies. A simple diagrammatic approach can often elucidate this complex process more clearly.
Tools and Technologies Used
The arena of cDNA library construction has witnessed revolutionary progression in technology. Here are some essential tools and methods commonly utilized:
- Poly(A) selection: This technique captures mRNA molecules that possess a polyadenylated tail. It enables the enrichment of high-quality mRNA necessary for cDNA synthesis.
- Size selection using gel electrophoresis: This tool helps ensure that only those cDNA fragments of a desired size range are retained, filtering out less relevant sequences.
- Next-generation sequencing (NGS): A critical tool for both characterizing cDNA libraries and conducting high-throughput gene expression profiling.
- PCR amplification: Widely employed for amplifying cDNA, ensuring that there’s sufficient quantity for subsequent experiments.
Advancements in automation technology have also significantly streamlined these procedures, allowing for higher throughput while reducing the likelihood of human error.
"Understanding the methodologies behind cDNA library creation is essential for grasping their applications in gene expression analysis.”
Discussion
Comparison with Previous Research
Looking at historical trends, cDNA libraries have undergone transformations that are nothing short of remarkable. Early attempts to create these libraries were cumbersome and yielded inconsistent results. However, with the advent of more sophisticated techniques and tools, the reliability and accuracy of cDNA libraries have dramatically improved. This upward trajectory in quality has propelled numerous discoveries in the field, impacting how we understand genetic regulation and expression.
Theoretical Implications
The creation and utilization of these libraries also present theoretical implications pertinent to molecular biology. Understanding how genes are expressed in response to specific stimuli allows researchers to construct models of gene regulation. Such models can inform future hypotheses and experimental designs, paving the way for innovative research in gene therapy and personalized medicine. As the field progresses, the symbiotic relationship between technology and theoretical frameworks becomes ever more pronounced.
Each section of the cDNA library journey contributes to a richer understanding of genetic material, revealing more layers to the multifaceted world of molecular biology.
Prologue to cDNA Libraries
In the realm of molecular biology, the exploration of complementary DNA (cDNA) libraries represents a significant leap into understanding gene expression, functionality, and the interplay of genetic material within living organisms. This introduction serves not only as a gateway to the complex techniques involved in cDNA library construction but also highlights its vital role in advancing our comprehension of various biological phenomena. Researchers and students alike benefit tremendously from delving into this essential aspect of modern genetics, as it lays the groundwork for subsequent discoveries in genomics, transcriptomics, and biotechnology.
Definition and Overview
A cDNA library is essentially a collection of cloned cDNA fragments that serve to represent the mRNA present in a particular tissue at a specific time. Unlike genomic DNA, which contains both coding and non-coding regions that can complicate analysis, cDNA libraries focus solely on the expressed genes of an organism. This focus allows scientists to capture a snapshot of gene activity, offering insights into how cells respond to various stimuli or developmental cues. The creation of cDNA libraries opens doors to a myriad of applications, including the study of gene expression patterns, the identification of novel genes, and the investigation of genetic disorders.
Key Features of cDNA Libraries:
- Specificity: Only the expressed genes are represented, providing relevance to the biological context.
- Flexibility: Libraries can be constructed from various tissues, species, or conditions.
- Utility: They are used in functional genomics, gene discovery, and more.
Historical Context and Development
The concept of cDNA libraries emerged in the 1970s, paralleling advancements in the understanding of DNA and RNA. The initial process of reverse transcription, where RNA is converted to cDNA, was developed to analyze mRNA. Researchers like Walter Gilbert and H. Robert Horvitz laid the groundwork for the methods that would soon become standard practice in genetics.
Over the years, techniques for constructing cDNA libraries have evolved, reflecting improvements in technology and a deeper understanding of genetic mechanisms. Early cDNA libraries were primitive in nature, often encountering issues such as incomplete representation of transcripts and bias towards more abundant mRNAs. However, advancements in cloning vectors and amplification techniques have significantly enhanced the quality and usability of these libraries. The emergence of high-throughput methods and next-generation sequencing later revolutionized how researchers approached cDNA libraries, making it quicker and easier to characterize and quantify the genetic material they house.
"The understanding of cDNA libraries has not only led to genomic discoveries but has also paved the path for innovations in personalized medicine and therapeutics."
Through this historical lens, it becomes evident that cDNA libraries have provided invaluable tools for scientists, allowing for the dissection of complex biological questions and fostering significant advancements in molecular biology and related fields.
Methodologies in cDNA Library Construction
The creation of cDNA libraries is a cornerstone technique in molecular biology as it allows researchers to delve into the complex world of gene expression. This section discusses critical methodologies employed during the construction of these libraries, shedding light on the importance of each step. From total RNA extraction techniques to the screening of clones, the entire process demands precision and an understanding of the underlying biology. Proper methodologies not only enhance the quality of the cDNA libraries but also directly impact the outcomes of subsequent experiments.
Total RNA Extraction Techniques
Before one can even think about constructing a cDNA library, it’s crucial to start with high-quality RNA. Total RNA extraction is the first step, and methods vary widely, each with its pros and cons. Techniques such as TRIzol extraction, silica column-based methods, and magnetic bead-based approaches come to mind.
- TRIzol Reagent: This is a classic method that effectively separates RNA from DNA and proteins. While it’s quite effective, the process can be a bit cumbersome and might require further purification steps.
- Silica Columns: Popular for their ease of use, these methods often deliver clean RNA quickly. However, they might not always extract the full spectrum of RNA species present.
- Magnetic Beads: These are increasingly being used for their speed and efficiency, allowing the extraction of RNA in relatively short timeframes.

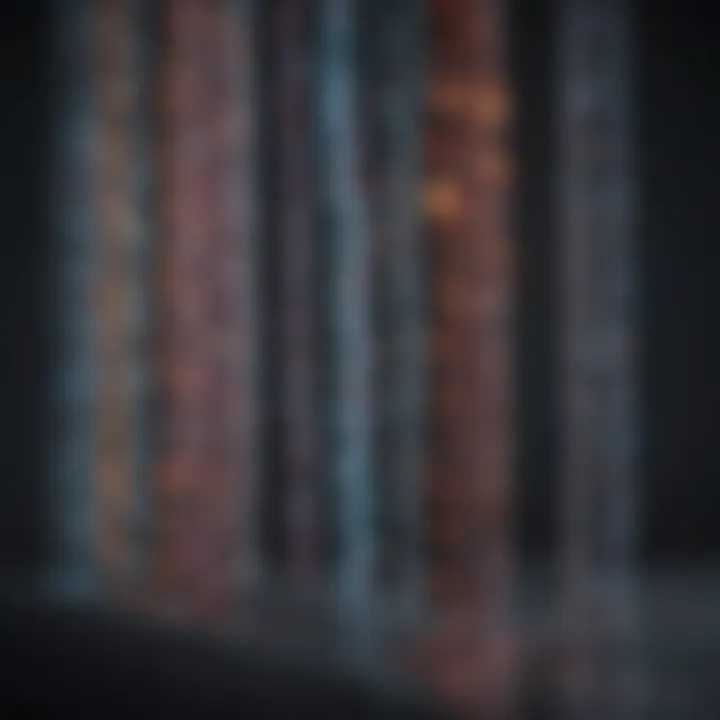
Each technique has nuances worth considering based on the sample type and downstream applications.
Reverse Transcription Processes
Once RNA is obtained, the next step is to synthesize complementary DNA through reverse transcription. This process is what gives cDNA its name, and it’s where the real magic happens. Reverse transcriptase enzymes, often derived from retroviruses, convert RNA templates into cDNA efficiently.
Important factors to consider:
- Enzyme Selection: Different reverse transcriptases have unique properties. Some work better with specific RNA lengths or types, and researchers must choose accordingly to optimize yield.
- Temperature and Buffer Conditions: These parameters can affect the enzyme activity and stability, thereby influencing the overall yield of cDNA.
The efficiency of reverse transcription can significantly impact the quality of the cDNA library created. If this step is not done properly, all of the downstream applications may be compromised.
Cloning Procedures and Vector Selection
After synthesizing cDNA, the next step is to insert it into cloning vectors. Here, selection of the right vector is crucial. Vectors serve as vehicles for cDNA, enabling replication and expression in a host organism.
- Plasmids: Often used for cloning due to their ability to replicate independently within host cells. Plasmids such as pUC19 have been standard choices for many experiments.
- Bacteriophages: These can be more potent in carrying larger fragments of cDNA. Lambda vectors, for example, can accommodate large inserts, allowing for more comprehensive libraries.
It’s necessary to take into account the possibility of inserting multiple cDNA fragments without losing essential genetic information. This step requires careful planning and precision to ensure successful cloning.
Screening and Characterization of Clones
Once the cloning process is complete, the next stage involves screening the transformed cells to identify successful clones. This is an increasingly vital phase where researchers gauge the diversity of sequences within their library. Different screening methods can be employed:
- Colony PCR: A rapid way to screen colonies for insertions by amplifying them directly from colony samples. This method allows for efficient evaluation of large numbers of clones.
- Hybridization Techniques: These can be used if specific sequences are known, leveraging labeled probes to find a match within the clone library.
Characterizing these clones based on their sequences later assists in determining functionality and diversity, aiding in downstream applications like functional genomics or expression studies.
In the creation of cDNA libraries, each methodological aspect plays a critical role in ensuring the integrity and utility of the library created.
Overall, mastering these methodologies lays the groundwork for successful applications of cDNA libraries in diverse research areas, enriching our understanding of gene expression and regulation.
Types of cDNA Libraries
Understanding the various types of cDNA libraries is essential for anyone looking to grasp the subtleties of gene expression studies and functional genomics. Each type offers distinct advantages and considerations, shaping the way researchers approach their inquiries in molecular biology. The choice of cDNA library impacts the research outcomes, serving as a foundation for insightful discoveries about gene functions and their interactions in diverse biological processes. This section will delve into three primary types of cDNA libraries—normalized, unnormalized, and amplicon libraries—exploring their unique characteristics and applications.
Normalized cDNA Libraries
Normalized cDNA libraries are designed with one primary aim: to enhance the representation of low-abundance transcripts. In simpler terms, normalization is the process of systematically adjusting the amount of each type of cDNA, ensuring that rarer genes are not drowned out by more abundant ones. This technique is particularly beneficial when exploring complex tissues or conditions where subtle gene expression differences are critical to understanding the biological questions at hand.
The methodology often involves steps like hybridization and subtraction to reduce the representation of highly expressed genes, resulting in a library that provides a more balanced view of the transcriptome. Researchers might employ normalized cDNA libraries when aiming to identify novel transcripts or when working with samples that contain a plethora of highly expressed RNAs—the proverbial needle in the haystack.
- Advantages of Normalized cDNA Libraries:
- Improved detection of low-expression genes
- More accurate profiling of transcriptomes
- Facilitation of discovery in complex biological systems
Though normalization has its benefits, it isn't without challenges. There's a risk that over-normalization can lead to the loss of important information about abundant transcripts, which can be crucial under certain experimental contexts.
Unnormalized cDNA Libraries
On the flip side, unnormalized cDNA libraries retain the original representation of cDNA, including all transcripts, regardless of their abundance. These libraries can be invaluable, especially when the aim is to capture the complete picture of transcript expression in a given sample. This full representation allows researchers to analyze highly expressed genes and examine their roles in various biological processes or diseases.
While they might not tackle the issue of low-abundance transcripts as effectively as their normalized counterparts, unnormalized libraries shine in studies focused on dominant gene expressions, like in cancer genomics, where highly expressed oncogenes might hold the key to understanding tumor biology.
- Benefits of Unnormalized cDNA Libraries:
- Comprehensive representation of transcriptome
- Useful in studies focused on dominant gene expression
- Easier to identify highly expressed genes, allowing for robust functional studies
That said, unnormalized libraries may lead to biases during analysis, especially where low-abundance transcripts are overlooked, potentially skewing research findings.
Amplicon Libraries
Amplicon libraries are a specialized form that involves the amplification of specific regions of the genome or cDNA sequences through techniques like PCR. These libraries can be particularly useful when exploring specific genes of interest or when validating the expression levels of targeted genes from a larger pool of transcripts.
Researchers often turn to amplicon libraries for high-throughput sequencing applications or when they want to focus on generating data for specific sequences, such as in targeted exome sequencing or metagenomics studies. The targeted nature of these libraries means that they can yield high-resolution data about the selected genes, aiding researchers in dissecting genetic variations and their implications in diseases.
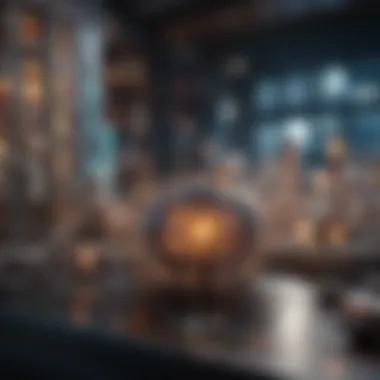

- Key Characteristics of Amplicon Libraries:
- Targeted amplification of specific sequences
- High-resolution data on gene expression
- Enables fine-tuning of research to focus on specific areas of interest
In summary, every type of cDNA library has its unique place within the landscape of molecular biology research. Choosing the right library depends largely on the research objectives, the biological question at hand, and the specific characteristics of the sample being studied.
"Selecting the proper type of cDNA library is akin to choosing the right tools for a craftsman; the outcomes rely heavily on the right fit for the job."
Applications of cDNA Libraries
cDNA libraries serve as a cornerstone for various molecular biology pursuits, bridging gaps in our understanding of gene function and molecular interactions. Their importance isn't just theoretical; they have real, tangible applications that can drive biological research forward. From gene expression profiles to the quest for novel genomic discoveries, cDNA libraries are crucial in yielding insights that shape our comprehension of complex biological systems. Let's explore these applications further to reveal their significance and contributions.
Gene Expression Studies
One of the primary applications of cDNA libraries is in the realm of gene expression studies. By creating a library that reflects the mRNA population from specific tissues or conditions, researchers can track which genes are active under certain physiological circumstances. This approach allows for comparative studies between healthy and diseased tissues to discern expression differences that may point to underlying pathologies.
The utilization of cDNA in gene expression analysis allows researchers to detect and quantify specific mRNA transcripts, facilitating the identification of overexpressed or underexpressed genes that play roles in disease processes. For example, in cancer research, cDNA libraries are instrumental in identifying genes linked to tumorigenesis. Ultimately, by unearthing these transcriptional landscapes, scientists can better understand the genetic foundations of diseases, which can lead to targeted therapies.
Functional Genomics
Functional genomics takes a step further by seeking to understand the roles of genes and their interactions within biological systems. cDNA libraries play an integral part here since they can be used to study the function of unknown genes by expressing them in model organisms, such as yeast or bacteria. When these libraries are transfected into host cells, researchers can observe the phenotypic outcomes – how the host cells respond to the introduced genes.
This practical application of cDNA libraries offers profound insights into gene function, suggesting potential pathways that might not be apparent through sequence analysis alone. Moreover, they help illuminate gene networks and regulatory mechanisms, important for grasping how genes communicate and contribute to cellular functions.
Discovery of Novel Genes
The discovery of novel genes remains a vital frontier in genomics, and cDNA libraries are often the key to unlocking this potential. By sequencing the cDNA inserts from a library, scientists can identify previously unknown transcripts, expanding the known gene repertoire. This is particularly important in lesser-studied organisms or conditions where sequences may diverge significantly from well-characterized species, making traditional genomic methods less effective.
Furthermore, cDNA libraries can reveal alternative splicing events and the presence of non-coding RNAs that might play roles in gene regulation and cellular processes. The identification of these novel genes can shed light on their evolutionary significance and may provide new targets for therapeutic interventions or advances in biotechnology.
"cDNA libraries not only empower us to understand gene expression but also pave the way for discovering treasures hidden within our genomes."
End
In sum, the applications of cDNA libraries span critical aspects of molecular biology, from studying gene expression patterns to unraveling the complexities of gene function and uncovering novel genetic material. Each application not only enhances our scientific toolkit but also serves as a beacon for future explorations in genetics and genomics. As science moves forward, cDNA libraries will undoubtedly continue to illuminate paths previously shrouded in mystery, solidifying their place as invaluable resources in the quest to decode the language of life.
Technological Advances in cDNA Library Creation
The realm of cDNA library construction has made remarkable strides in recent years due to technological advancements. These improvements not only streamline the processes involved but also significantly enhance the quality and richness of the data generated. The intersection of cutting-edge technology with molecular biology facilitates unprecedented opportunities in research and application. Researchers are now equipped with tools that increase throughput, decrease errors, and, crucially, allow for more detailed analyses of genetic material than ever before.
Next-Generation Sequencing Technologies
Next-generation sequencing (NGS) has revolutionized the approach to cDNA library construction and analysis. Traditional sequencing methods were often slow and costly, making them less suitable for large-scale studies. NGS changes the game by enabling massively parallel sequencing, effectively processing thousands, if not millions, of sequences simultaneously. This technology provides comprehensive insights into gene expression patterns, allowing scientists to identify not only known but also novel transcripts in a given sample.
The adoption of NGS in cDNA libraries delivers several benefits:
- High Throughput: Researchers can sequence an entire library in a fraction of the time traditional methods would require, which is crucial for high-volume projects.
- Cost Efficiency: With a decrease in the cost per base pair sequenced, research projects that once required significant budgets are now more accessible.
- Data Richness: NGS offers a wealth of data that can reveal subtle variations in gene expression and help in identifying rare transcripts that might have been overlooked previously.
However, the use of NGS is not without its challenges. One notable concern is the analysis of the vast data sets generated. A typical NGS output necessitates sophisticated bioinformatics tools and expertise to interpret the results meaningfully. Thus, the combination of advanced sequencing technologies and skilled data analysts is essential for making the most out of this method.
Automation in Library Construction
As the demand for cDNA libraries grows, the need for streamlined, efficient methods has become apparent. Automation in cDNA library construction has emerged as a key player in addressing these pressures. By integrating robotic systems and sophisticated software, laboratories can minimize human error and enhance the reproducibility of results.
Automation protocols can oversee numerous steps in library preparation, which include:
- RNA Extraction: Automated systems can significantly increase the speed and consistency of total RNA extraction processes.
- cDNA Synthesis: Robotic liquid handling systems can accurately dispense reagents and manage multiple samples simultaneously, reducing user variability.
- Cloning Steps: Automated instruments can facilitate the transformation of competent cells or the packaging of libraries into vectors, thus expediting the cloning stage.
These advancements ensure that researchers focus their efforts on data interpretation and downstream analysis rather than labor-intensive procedures. While the initial investment in automation technology can be significant, the long-term benefits in terms of efficiency and quality control can outweigh these costs.
"With advances in automation, labor in the lab becomes more about making critical decisions rather than executing repetitive tasks."
In summary, technological advances in cDNA library creation are reshaping the landscape of molecular biology. The integration of next-generation sequencing and automation systems not only enhances the libraries themselves but also promotes a more nuanced understanding of gene functions and interactions. As these technologies continue to evolve, they promise to unravel even more complexities of biological systems, paving the way for future discoveries.
Challenges in Creating cDNA Libraries
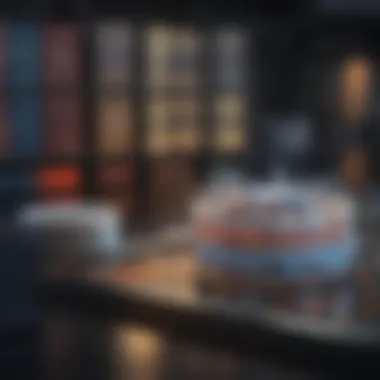

Creating complementary DNA (cDNA) libraries is a multifaceted venture that brings along a unique set of challenges that must be navigated with precision. The significance of addressing these challenges can't be overstated, as they bear direct implications on the efficacy of the libraries produced. Understanding these hurdles offers insights into both the potential pitfalls and the avenues for improvement in methodologies and technologies employed in the realm of molecular biology.
Technical Limitations and Biases
When delving into the technical aspects of cDNA library construction, various limitations can arise that may skew results. One prominent concern is the uneven representation of different mRNA species. Typically, abundant mRNA transcripts can mask less abundant ones, leading to a library that fails to fully capture the diverse landscape of gene expression.
For instance, in constructing libraries from tissue samples, certain highly expressed genes can dominate the cDNA synthesis process. This can result in an overwhelmingly biased representation that could conceal rare or tissue-specific transcripts that may be critical for understanding the biological context.
Further compounding this issue, the efficiency of reverse transcription varies among different polyadenylated RNAs. As a result, some transcripts may be underrepresented while others appear in disproportionate amounts.
"In the world of cDNA libraries, balance is key, but achieving that balance often poses a herculean task."
To mitigate these biases, researchers often utilize normalization techniques. Yet, these methods come with their own sets of limitations and can introduce their own biases. In normalizing cDNA libraries, one might inadvertently amplify certain transcripts, thus skewing the representation once again.
Data Analysis and Interpretation Issues
The road doesn’t end with the creation of a cDNA library. The subsequent data analysis presents its own distinct challenges. Researchers are faced with the intricate task of interpreting complex datasets, which often come laden with noise and variation. Each step, from sequencing results to quantifying expression levels, requires a careful touch to sidestep potential pitfalls.
Simply put, even if a cDNA library successfully captures gene expression, making sense of the resulting data can be like finding a needle in a haystack. Misinterpretations can stem from a lack of appropriate controls or software tools that fail to account for technical variations between samples.
Moreover, it’s not enough to merely generate data; researchers must also address the biological relevance of their findings. Experimental biases, such as library-preparation artifacts, can confound results further, rendering it essential for scientists to maintain a critical perspective throughout their interpretations.
Navigating these challenges requires a blend of statistical acumen and biological insight. Without a solid grasp of both, what could be groundbreaking research may simply become a mere collection of inconclusive results.
Future Directions in cDNA Library Research
The exploration of future directions in cDNA library research is not merely an academic exercise; it is critical to advancing the boundaries of molecular biology. As we venture further into understanding gene expression and the intricate pathways of biological functions, recognizing potential enhancements in cDNA library methodologies becomes paramount. This section highlights specific elements such as the integration of emerging technologies and the prospects for personalized medicine. These advancements promise not only to refine our existing techniques but also to reshape our approach to genomic studies.
Integration of Emerging Technologies
The integration of new technologies presents a significant opportunity to upgrade the process of constructing cDNA libraries. Tools such as CRISPR-Cas9, single-cell RNA sequencing, and bioinformatics have started to take center stage. For instance, single-cell RNA sequencing allows researchers to observe gene expression at the single-cell level, significantly increasing the resolution of data that cDNA libraries can provide. This enables scientists to uncover heterogeneity within populations of cells, offering a more detailed understanding of cellular functions.
Moreover, emerging computational techniques are revolutionizing data processing and analysis. Traditionally, analyzing cDNA library data could feel like searching for a needle in a haystack. However, advanced machine learning algorithms are enhancing the efficiency of this process, enabling researchers to quickly filter through vast amounts of genetic information and extract meaningful insights. Here’s how these technologies are changing the game:
- Precision: Enhanced accuracy in capturing gene expression profiles.
- Speed: Faster processing of sequencing data, allowing for quicker experimental feedback.
- Heterogeneity Analysis: Facilitating the exploration of cell-to-cell variations that were previously overlooked.
These advances are not just theoretical; they are impacting tangible research outcomes and encouraging innovative applications of cDNA libraries across various fields, from basic science to therapeutic development.
Prospects for Personalized Medicine
As we look down the road, the prospects for personalized medicine driven by cDNA libraries are nothing short of transformative. The ability to tailor treatments based on an individual's unique genetic makeup is a cornerstone of modern medical advancements. cDNA libraries serve as a crucial resource for understanding the complex genetic underpinnings of diseases, which can lead to better-targeted therapies.
Why is personalized medicine so compelling? As we harness the insights gained from cDNA libraries, we stand to shift from a one-size-fits-all approach to a much more nuanced methodology. This could result in:
- Customized Treatment Plans: Clinicians can design therapies specific to a patient's gene expression profile, potentially increasing the efficacy of treatments while minimizing side effects.
- Proactive Healthcare Models: Instead of responding to disease, we may identify risks early, allowing for preventive measures tailored to genetic predispositions.
- Innovative Drug Development: By exploring novel genes discovered through cDNA libraries, researchers can target new pathways for drug discovery and development, addressing previously unmet medical needs.
Navigating the future of cDNA library research involves recognizing these confluences between technological innovation and clinical application. As both academic institutions and biotechnology companies lean into these methodologies, the horizon for research becomes richer and more promising than ever.
"Personalized medicine is the hallmark of modern healthcare, and cDNA libraries offer the insights required to usher this revolution into practice."
By fostering collaborations across disciplines and maintaining a keen eye on technological advancements, cDNA libraries can become powerful tools not just for research but for applying our discoveries directly to patient care.
Epilogue
The conclusions drawn from this comprehensive exploration of cDNA libraries showcase their pivotal role in advancing molecular biology. As the research landscape continually evolves, the importance of these libraries cannot be overstated. They serve not merely as biotechnological tools, but as critical gateways to understanding the intricate dance of genes and their expression.
Summary of Key Insights
Throughout the article, several key insights have emerged:
- Versatility: cDNA libraries are versatile in application, ranging from gene expression studies to functional genomics, encapsulating a broad spectrum of research disciplines.
- Construction Methodologies: The processes involved in library construction, from total RNA extraction to reverse transcription and cloning techniques, hinge on meticulous methodologies that influence the library's quality.
- Technological Advances: Recent technological advancements, including next-generation sequencing and increased automation, have streamlined library creation, enhancing accessibility and efficiency.
- Challenges: While significant strides have been made, challenges, particularly technical limitations and data analysis hurdles, remain an ongoing concern in cDNA library research.
Thus, it is clear that cDNA libraries lie at the intersection of technology and biology, serving as invaluable resources for researchers determined to decode the genetic underpinnings of life.
The Ongoing Relevance of cDNA Libraries
As we look ahead, the relevance of cDNA libraries persists, more than ever. They have opened doors for researchers to delve into the complexities of gene functions, the interplay of proteins encoded by these genes, and their roles in health and disease. With the trajectory of personalized medicine aiming to tailor treatments based on individual genetic make-ups, these libraries will increasingly provide foundational insights.
Moreover, the integration of emerging technologies, such as CRISPR and artificial intelligence, alongside traditional cDNA library methodologies, holds promise for even greater breakthroughs. In contexts where understanding gene regulation and expression is crucial, cDNA libraries will remain essential tools.
In summary, as the scientific community continues to navigate the multifaceted challenges within genomics and proteomics, the ongoing relevance and evolution of cDNA libraries will undoubtedly shape future research and applications alike.