Carbon Capture and Underground Storage for Climate Action
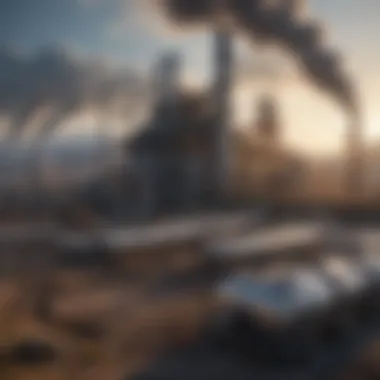
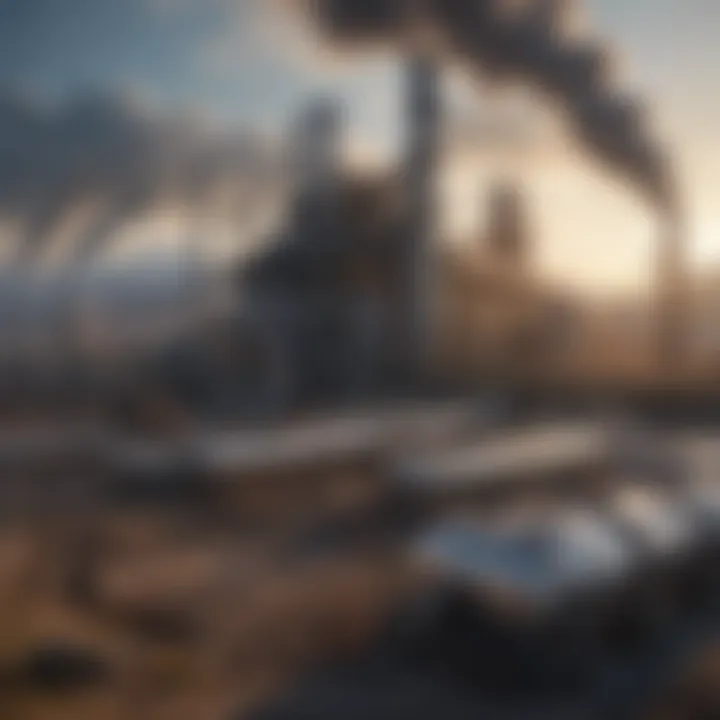
Intro
Carbon capture and underground storage (CCUS) is a pivotal technology in the global efforts to combat climate change. As the threat of global warming intensifies, the need for innovative solutions becomes ever more critical. This technology aims to reduce carbon dioxide emissions directly from the sources before they reach the atmosphere, offering a lifeline to industries that are hard to decarbonize, such as cement and steel manufacturing.
By capturing carbon dioxide and storing it underground, this approach not only mitigates greenhouse gas emissions but also creates possibilities for sustainable future energy solutions. This article aims to delve into the methodologies, challenges, and long-term implications of CCUS, providing a comprehensive overview for students, researchers, educators, and professionals alike.
Methodologies
Understanding carbon capture and underground storage requires knowledge of the methodologies used in research and implementation. These methodologies fall primarily into two categories: the techniques for capturing carbon dioxide and the methods for its effective storage underground.
Description of Research Techniques
The research techniques employed in carbon capture can be broadly classified into three main types:
- Post-combustion capture: This method involves capturing carbon dioxide from flue gases after fossil fuels have been burned. It is commonly used in power plants.
- Pre-combustion capture: Here, carbon is removed before burning the fuel, typically through gasification. This technique is effective for industries where hydrogen production is essential.
- Oxy-fuel combustion: This method uses pure oxygen instead of air to burn fuel, resulting in a flue gas that is primarily carbon dioxide, making it easier to capture.
These techniques are essential for understanding how to enhance carbon dioxide removal efficiency without disrupting industrial processes.
Tools and Technologies Used
Various tools and technologies play a crucial role in the CCUS process:
- Absorption systems: These systems use chemical solvents to absorb carbon dioxide from exhaust gases.
- Membrane technologies: They utilize selective membranes to separate carbon dioxide from other gases.
- Cryogenic separation: This involves cooling the gas mixture to separate carbon dioxide based on its boiling point.
Each tool presents unique advantages and challenges, providing researchers with options to tailor their approach based on specific industry needs and environmental considerations.
Discussion
Comparison with Previous Research
Understanding advancements in CCUS technologies requires a look at previous studies. Research has demonstrated significant improvements in capture efficiency and reductions in costs over the years. Earlier methods were often energy-intensive and economically unviable. Recent innovations focus on lower energy consumption and better integration into existing industrial infrastructures, making CCUS a more feasible option for reduction of emissions.
Theoretical Implications
Theoretical implications extend to how we view carbon management in the broader context of climate strategies. CCUS could potentially allow for the continued use of fossil fuels while mitigating emissions, thus acting as a bridge during the transition to more sustainable energy systems. By adopting this dual approach, we may better achieve a balance between energy needs and environmental responsibilities.
Carbon capture and underground storage represent not only a technological evolution but also a strategic necessity in the climate change mitigation discourse.
Preamble
The issue of climate change is pressing and compels urgent attention. One of the critical strategies to mitigate its effects is carbon capture and underground storage. This process helps reduce the amount of carbon dioxide released into the atmosphere, a major greenhouse gas contributing to global warming. The importance of carbon capture lies not only in its potential to decrease CO2 concentrations but also in aligning with global climate goals.
As atmospheric levels of carbon dioxide continue to rise, innovative technologies are becoming central to environmental sustainability efforts. Carbon capture and storage can play a pivotal role in various sectors, including power generation and industrial activities. It allows for the continued use of fossil fuels while addressing their environmental impact, which is crucial during the transition towards renewable energy sources.
Moreover, effective carbon capture can serve as a bridge between current energy systems and future low-carbon futures. Through this article, we delve into the mechanics of carbon capture technologies, the importance of selecting appropriate underground storage sites, and regulatory frameworks shaping these practices.
This article seeks to provide a comprehensive understanding of carbon capture and underground storage, detailing significant technological advancements, exploring various methodologies, and addressing financial and public perceptions surrounding this essential aspect of climate change mitigation. By unpacking these complexities, the article aims to inform students, researchers, educators, and professionals about the multifaceted nature of addressing climate change.
The Importance of Carbon Capture
Carbon capture plays a vital role in the global effort to mitigate climate change. It presents a tangible solution to reduce the levels of carbon dioxide (CO2) in the atmosphere, which is one of the main greenhouse gases responsible for global warming. Capturing CO2 from industrial processes and power generation can significantly lower emissions, thus helping in the fight against climate change.
The urgency of addressing carbon emissions is not just an environmental issue; it is also a matter of public health, economic stability, and sustainability. As nations grapple with the impacts of climate change, the importance of carbon capture becomes increasingly clear. It offers several benefits:
- Reduction of Atmospheric CO2: By capturing and storing CO2, we can directly address the high concentration of this gas in the atmosphere.
- Support for Industry: Many industries contribute significantly to carbon emissions. The implementation of carbon capture technology allows them to continue operating while committing to reducing their environmental footprint.
- Compatibility with Existing Infrastructure: Many capture technologies can be integrated into existing power plants and industrial facilities. This integration reduces the need for complete overhauls of current systems.
- Economic Opportunities: Developing carbon capture technologies can create new jobs and stimulate economic growth through investments in research and infrastructure.
Recognizing these factors, many governments and organizations are now investing resources into developing and deploying carbon capture technologies as part of a larger strategy to combat climate change.
Understanding Carbon Emissions
Understanding carbon emissions is critical for grasping the significance of carbon capture. CO2 is emitted primarily through burning fossil fuels for energy as well as through other processes like cement production and waste management. The amount of CO2 released into the atmosphere has steadily increased over decades, contributing to global warming and climate-related disasters.
Emissions can be categorized into three scopes:
- Scope 1: Direct emissions from owned or controlled sources.
- Scope 2: Indirect emissions from the generation of purchased electricity, steam, heating, and cooling consumed by the reporting company.
- Scope 3: All other indirect emissions that occur in a companyโs value chain.
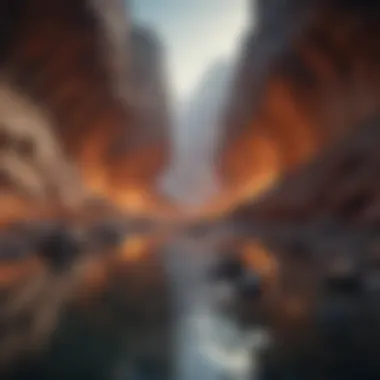
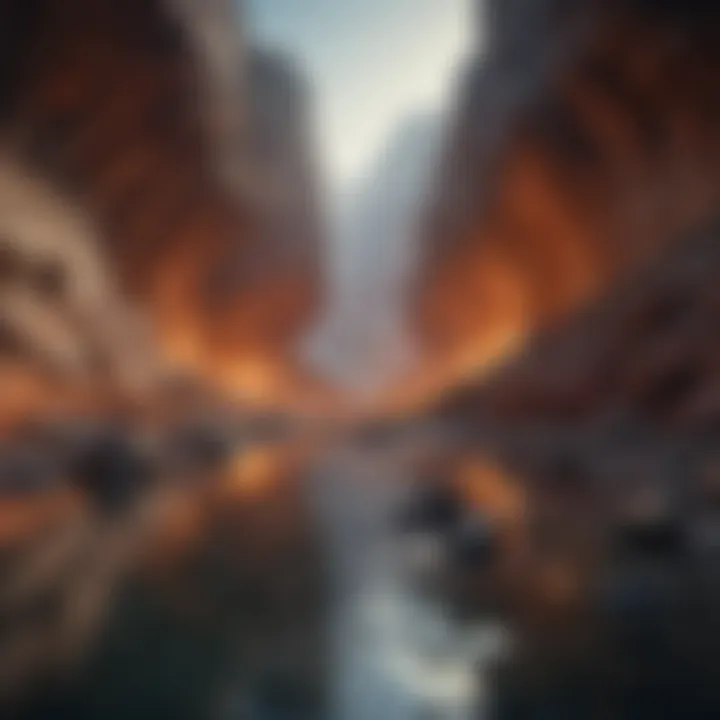
Understanding these categories helps organizations identify not just where emissions are coming from, but also the potential areas for intervention through carbon capture.
Link to Climate Change
The connection between carbon emissions and climate change is straightforward. Increased concentrations of CO2 and other greenhouse gases lead to a rise in global temperatures. This phenomenon impacts weather patterns, sea levels, and overall planetary health. Consequently, carbon capture seeks to dampen this trend by reducing the volume of CO2 released into the atmosphere.
The World Meteorological Organization reports that since the late 18th century, the global average temperature has risen by about 1.1 degrees Celsius. This increase is closely interpolated with the rising levels of atmospheric CO2, which has moved from approximately 280 parts per million (ppm) in pre-industrial times to over 400 ppm today.
To combat these effects, carbon capture is not merely beneficial; it is essential. As public policy evolves to mitigate climate change, carbon capture technology offers one of the most promising strategies in reducing overall greenhouse gas emissions.
Technologies for Carbon Capture
The technologies for carbon capture are significant components of climate change mitigation strategies. By capturing carbon dioxide (CO2) emissions before they enter the atmosphere, these technologies address a key driver of global warming. The effective implementation of carbon capture technologies can lead to cleaner industrial processes and lower greenhouse gas levels. These innovations are crucial in the transition to a more sustainable economy.
Post-Combustion Capture
Post-combustion capture refers to methods where CO2 is removed from the flue gases produced after fossil fuels are burned. This technology is prevalent because it is applicable to existing power plants without substantial modifications. The primary challenge faced in post-combustion capture is the energy requirement for the separation process. Monoethanolamine is often used as an absorbent; it captures CO2 through a chemical reaction, forming a stable compound. Although effective, the heat needed to regenerate the absorbent is significant and can reduce the overall efficiency of the power plant. Therefore, advances in materials and processes aim to lower these energy demands while maintaining high capture rates.
Pre-Combustion Capture
Pre-combustion capture involves gasifying fossil fuels to create a synthesis gas, primarily consisting of hydrogen and carbon monoxide. The subsequent reaction converts carbon monoxide into CO2, which can then be captured. This approach has the advantage of producing hydrogen, which can be used as a clean fuel. The conversion process requires specific infrastructure, meaning it is currently more suitable for new power plants rather than retrofitting existing ones. Ultimately, pre-combustion capture can provide efficiency gains and a reduction in emissions, but it demands substantial investment in technology and facility construction.
Oxy-Fuel Combustion
Oxy-fuel combustion is an innovative technology that burns fossil fuels in pure oxygen instead of air. This method results in flue gases predominantly composed of CO2 and water vapor, simplifying the capture process since it eliminates nitrogen from the combustion gases. The primary challenge with oxy-fuel combustion lies in producing the required oxygen at a scale that is both economically and technically viable. This technology holds promise for increasing the efficiency of power generation while significantly reducing emissions, but significant research and development are still needed to optimize performance and cost.
"Investments in carbon capture technologies are vital for achieving climate targets and promoting sustainable energy solutions."
In summary, each carbon capture technology offers unique benefits and challenges. The choice between post-combustion, pre-combustion, or oxy-fuel combustion will depend on various factors including cost, existing infrastructure, and emission reduction goals. Continued advancements and investments in these technologies are essential to meet global climate change objectives.
Underground Storage of CO2
Underground storage of carbon dioxide (CO2) is a critical component in the strategy to combat climate change. The aim is to prevent CO2 emitted from industrial processes and energy production from entering the atmosphere. By storing it deep underground, we ultimately reduce the greenhouse gases contributing to global warming. This section explores the geological formations suitable for storage, including saline aquifers, oil and gas reservoirs, and coal seams. It also looks at storage mechanisms that include structural trapping, capillary trapping, and dissolution trapping.
Geological Formations
Saline Aquifers
Saline aquifers are one of the most promising options for CO2 storage. These are underground layers of water that have a high concentration of salt. A significant advantage of saline aquifers is their vast capacity, far surpassing that of other reservoirs. The crucial characteristic of saline aquifers is their ability to dissolve CO2 in water, making them a long-term storage solution. This unique feature allows for large-scale CO2 storage, facilitating substantial reductions in atmospheric carbon. However, potential disadvantages include the need for extensive site characterization and the risk of groundwater contamination if not managed properly.
Oil and Gas Reservoirs
Oil and gas reservoirs represent another potential site for CO2 storage. These reservoirs already contain hydrocarbons, which indicates they can effectively seal gases. Their primary advantage is the existing knowledge of geology collected from long-term extraction activities. This familiarity provides a reliable framework for storing CO2. However, the challenge lies in identifying suitable reservoirs that have not been depleted, limiting their availability for widespread use.
Coal Seams
Coal seams offer a unique method of CO2 storage as well. They are porous and can absorb CO2, sometimes enhancing the extraction of methane. One of the key benefits of this approach is the dual use of coal seams for energy production and carbon storage. However, the effectiveness depends on the coal's permeability and the coal seam's geographic location. Monitoring these sites may also require additional resources to ensure safety and efficiency.
Storage Mechanisms
Structural Trapping
Structural trapping is one of the primary methods of keeping CO2 underground. This process depends on the natural geological formations acting as barriers, preventing the upward migration of CO2. Structural trapping is significant because it offers a passive method of containment. This mechanism allows for stable long-term storage, yet its effectiveness heavily relies on the integrity of the surrounding rock formations. Over time, there could be concerns about geological stability, requiring ongoing assessments.
Capillary Trapping
Capillary trapping is another essential mechanism that helps in CO2 storage. It involves the process of CO2 being retained in the pores of rocks due to surface tension. The uniqueness of capillary trapping is its ability to occur in diverse geological formation types. It provides an added layer of security by immobilizing CO2 within the rock's microstructure. However, the main disadvantage is that its effectiveness can vary based on the rock's properties, which requires careful analysis.
Dissolution Trapping
Dissolution trapping refers to the process where CO2 gets dissolved into the brine water found in geological formations. This mechanism is particularly advantageous because it helps with long-term storage and reduces the risks of leakage. Additionally, as CO2 dissolves, it can lead to mineralization, transforming into solid carbonate minerals over time. The downside is that it takes time for the CO2 to dissolve effectively, delaying the unfavorable impacts of emissions.
Effective underground storage of CO2 is paramount in the fight against climate change, creating opportunities for safe and permanent disposal methods.
Assessment of Storage Sites
The assessment of storage sites is crucial in the discourse of carbon capture and underground storage. Its role lies in ensuring that carbon dioxide (CO2) is safely and effectively sequestered underground. Proper assessment allows us to mitigate risks associated with leaks and environmental damage. This section will analyze specific criteria necessary for evaluating potential storage sites, along with the monitoring processes involved to verify safety and effectiveness.
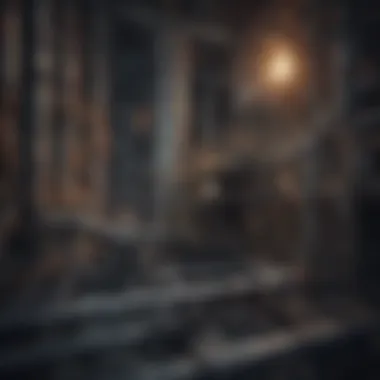
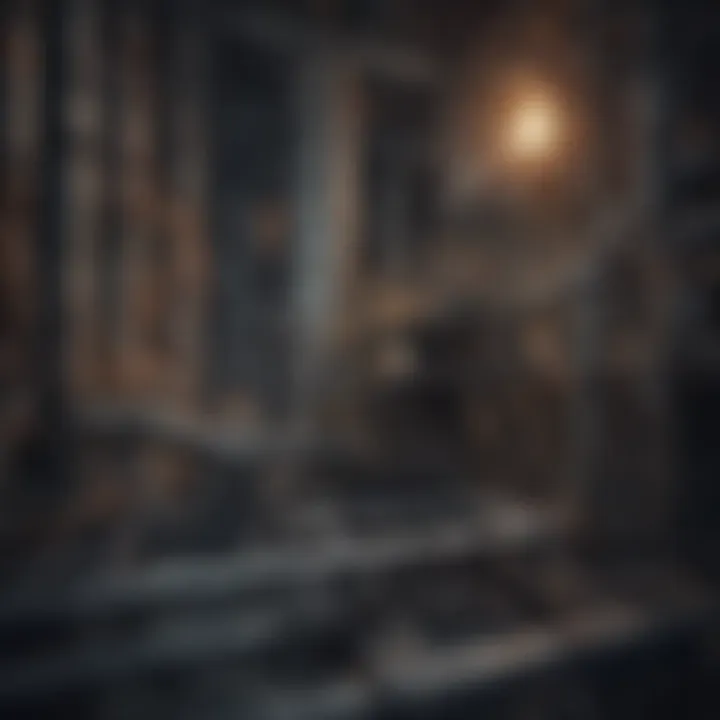
Site Selection Criteria
Depth and Integrity
Depth and integrity are vital for ensuring the secure storage of carbon dioxide. The depth of a storage site is important because it determines pressure conditions, which influence the behavior of CO2 underground. A key characteristic of suitable depths is they usually range from 800 meters to 3,000 meters. This depth range is beneficial as it assures higher pressure, maximizing CO2 solubility in geological formations.
A unique feature of depth is how it relates to geological integrity. Deeper formations often have a natural barrier, which limits the possibility of leakage. However, the challenge lies in accurately assessing the geological characteristics below the surface, which can be complex and variable.
Capacity
Capacity refers to the volume of CO2 that a geological formation can hold. This factor is critical for evaluating the long-term viability of a storage site. A key characteristic is the porosity and permeability of the rock formation. High-quality reservoirs can store significant amounts of CO2, making them favorable choices for long-term sequestration.
Capacity has its unique aspects; for instance, large saline aquifers can offer immense storage potential. However, high capacity also raises concerns regarding monitoring and potential pressure build-up, which can affect the geology surrounding the reservoirs.
Seismic Stability
Seismic stability assesses how a storage site will behave during seismic events. Understanding the geological framework's ability to withstand natural disturbances is vital in the context of safety and public acceptance. A key characteristic is the historical seismic activity in the region, which helps predict future behavior.
Seismic stability is beneficial because it underpins the credibility of storage operations. Unique features include conducting thorough seismic surveys before site selection. However, a disadvantage can arise from the difficulty in guaranteeing that minor seismic events won't occur, impacting regional geological integrity over time.
Monitoring and Verification
Monitoring and verification are indispensable in ensuring the effectiveness of carbon capture systems. They emphasize the need for consistent checks and balances once CO2 has been stored. This process involves tracking CO2 movement and assessing any potential leaks.
Baseline Surveys
Baseline surveys are the first step in a reliable monitoring plan. They establish the initial conditions of a storage site before CO2 injection begins. A key characteristic is collecting data on geological, hydrological, and geochemical properties.
The importance of baseline surveys cannot be overstated. They provide essential information for comparison in later stages. A unique feature is their role in establishing safety margins. However, extensive baseline surveys can incur significant costs and time delays before starting actual storage operations.
Injection Monitoring
Injection monitoring assesses the behavior of CO2 during the injection process. This monitoring is critical in ensuring that CO2 is absorbed correctly and safely without unexpected leaks. One key characteristic is the use of pressure and flow measurements to track CO2 migration underneath the surface.
Injection monitoring is a beneficial process. It helps identify any changes in fluid movement early on. A unique feature is the adaptability it offers, as monitoring technology can improve over time. However, this requires ongoing investment, which can be seen as a disadvantage for some operators.
Long-term Surveillance
Long-term surveillance focuses on ensuring the safety of stored CO2 over years or decades. A critical aspect is verifying that the geology surrounding the storage remains stable. This involves periodic monitoring and assessment of the site.
Long-term surveillance is beneficial because it builds trust with stakeholders and the general public. A unique aspect includes the integration of advanced technology like remote sensing for ongoing evaluation. Disadvantages may include costs and a continuous obligation to maintain observation and reporting processes over many years.
Adequate assessment of storage sites ensures the safe long-term containment of CO2, safeguarding both the environment and public confidence in carbon capture initiatives.
Regulatory and Policy Framework
The regulatory and policy framework surrounding carbon capture and underground storage is vital for the successful implementation and growth of these technologies. Effective regulations ensure safety, environmental protection, and promote public trust in these projects. They guide stakeholders by providing clear expectations and allow for standardization across various regions.
A robust regulatory framework encourages investment in carbon capture and storage (CCS) technologies. Both private and public entities seek assurance that their financial commitments are safeguarded by established laws and guidelines. Policies that promote accountability and transparency play a crucial role, particularly in the context of climate change and environmental stewardship.
Moreover, a comprehensive approach to regulation fosters international cooperation. Climate change is a global issue, and solutions require a collective effort. Therefore, international guidelines and national regulations must align, creating a consistent global response to carbon emissions. This alignment nurtures innovation, as nations can share best practices and technologies that have proven effective.
"A strong regulatory framework not only protects the environment but also catalyzes investment in innovative technologies for a sustainable future."
International Guidelines
International guidelines for carbon capture and storage focus on best practices and safety measures. Organizations such as the Intergovernmental Panel on Climate Change (IPCC) and the International Energy Agency (IEA) provide frameworks that nations can adopt. These guidelines address critical aspects such as the assessment of potential sites for storage, tracking and monitoring of stored carbon, and protocols for risk management.
These international directives encourage countries to develop their regulatory measures tailored to their unique geological and economic conditions, while maintaining a commitment to global climate goals. Addressing issues like long-term storage liability and potential leakage of CO2 is crucial, as is the need for regular reporting on emissions reductions achieved through CCS. Such practices help maintain rigorous scientific standards and contribute to the credibility of carbon capture initiatives.
National Regulations
National regulations are essential for the practical execution of international guidelines. Each country must translate these broader principles into localized laws and oversight frameworks that reflect their specific geological, political, and economic realities. This includes creating permits for capture and storage facilities, establishing safety protocols, and setting penalties for non-compliance.
Many countries have begun to draft laws that provide incentives for investing in CCS projects. These may include tax breaks or grants aimed at organizations that implement carbon capture technologies. Legal certainty helps attract investments by clarifying responsibilities and benefits associated with both public and private participation. Moreover, the establishment of national oversight bodies ensures that industry practices align with environmental and safety standards.
Economic Implications of Carbon Capture
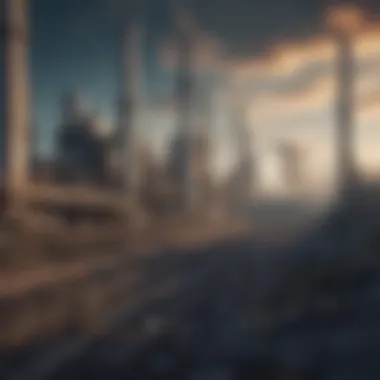
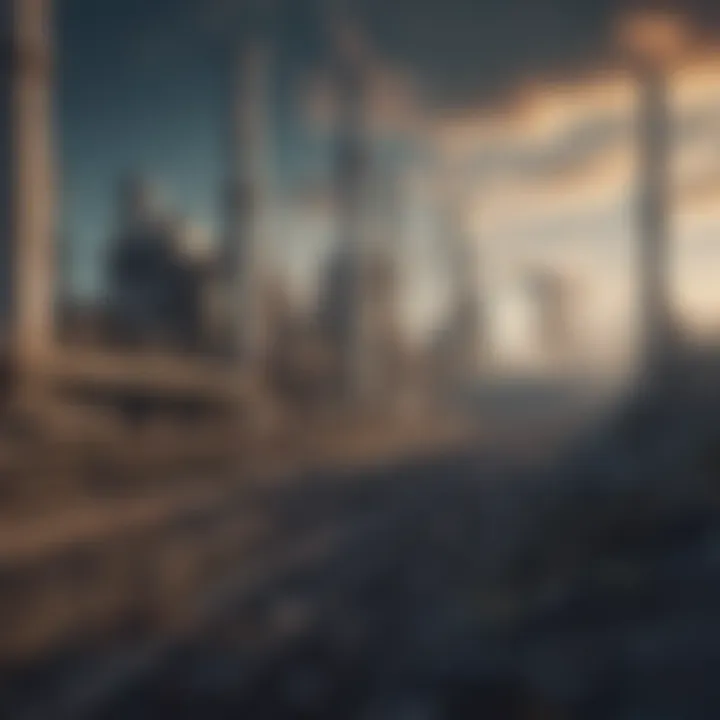
The economic implications of carbon capture are crucial in understanding its potential role in combating climate change. As countries and organizations strive towards achieving carbon neutrality, understanding the financial aspects while implementing carbon capture technologies becomes indispensable. Evaluating costs, potential financial incentives, and overall impacts will provide insights into the feasibility and sustainability of adopting these technologies on a broader scale.
Cost Analysis
Capital Costs
Capital costs refer to the initial investments required to establish carbon capture facilities and systems. A significant characteristic of capital costs is that they can vary considerably based on technology, scale, and location. For instance, the deployment of post-combustion capture systems often entails higher upfront investment, making it important for stakeholders to carefully assess the financial viability before committing resources. The unique aspect of capital costs is that they encompass not only the physical infrastructure but also technology licensing and integration into existing facilities. Despite the higher initial costs, investing in these technologies can yield long-term savings and environmental benefits. It is a salient point for this article as it highlights the importance of viewing capital costs as part of a broader strategy for climate action rather than just an immediate financial burden.
Operational Expenses
Operational expenses denote the ongoing costs associated with running carbon capture systems. This area is critical as it impacts long-term project sustainability. One key characteristic of operational expenses is that they can fluctuate based on energy prices and maintenance requirements. Operational efficiency needs to be maximized to ensure that projects remain financially viable over time. The unique feature of operational expenses lies in their sensitivity to technological advancements. Enhanced efficiency and innovation can significantly reduce these costs, making the deployment of carbon capture more attractive. Addressing operational expenses in this article is essential because it elucidates the financial challenges that could deter investment if not managed effectively.
Potential Financial Incentives
Exploring financial incentives can illuminate pathways for increased adoption of carbon capture technologies. Governments and organizations are increasingly offering incentives such as tax credits, subsidies, and grants to encourage investment in low-carbon technologies. These incentives can significantly reduce some of the initial and ongoing costs associated with carbon capture, making it more appealing to companies. Furthermore, carbon credits can be an indirect incentive, allowing companies to monetize their reductions in carbon emissions, thereby creating a potential revenue stream. Understanding these financial mechanisms is important for evaluating the broader economic landscape of carbon capture implementation.
"The future of carbon capture technologies can be enhanced through favorable economic policies and innovative financing solutions."
The economic aspects presented in this section underscore that carbon capture is not only an environmental imperative but also a financially strategic choice in many cases. By addressing both costs and incentives, stakeholders can make informed decisions about their investments in carbon capture and its role in combatting climate change.
Challenges and Limitations
The section on challenges and limitations is crucial for understanding the broader context of carbon capture and underground storage. It brings to light not only the technical elements but also the societal perceptions and misconceptions surrounding these technologies. Addressing these challenges is imperative for fostering public trust and facilitating the acceptance of carbon capture methods. It also assists policymakers and industry leaders in strategizing effective implementation plans.
Technical Challenges
Technical challenges pose significant barriers to the successful application of carbon capture technologies. One major issue is the efficiency of capture systems. Current systems often require large amounts of energy, which can reduce net emissions savings. For instance, post-combustion capture technologies might capture about 90% of carbon dioxide, but the energy demand for this process can be quite high. Additionally, the infrastructure needed for transportation and storage of captured CO2 can be complex and costly to develop.
Leakage is another concern, particularly in geological formations chosen for underground storage. A failure in storage integrity can lead to carbon dioxide escaping back into the atmosphere, undermining the purpose of the technology. Monitoring and verification methods, while improving, still require validation and advancement to ensure the long-term safety of storage sites.
Public Perception
Public perception remains a formidable challenge in the domain of carbon capture and underground storage. Skepticism surrounding the safety and effectiveness of these technologies can hinder their adoption. The general public often holds misconceptions about the risks associated with CO2 storage. For example, instances of natural gas leakage or catastrophic geological events can amplify fears. Ensuring transparency and providing clear, accessible information is critical for changing these perceptions.
Education plays a vital role in shaping public opinion. This sector needs to engage actively with communities to communicate both the benefits and the risks associated with carbon capture technologies. Continued dialogue with stakeholders, including local communities, environmental groups, and industry experts, can provide a balanced understanding of the long-term implications of carbon storage.
"Understanding both public concerns and technical limitations is essential in the push for widespread adoption of carbon capture technologies."
The challenges posed by technical limitations and public perception are intertwined. Overcoming technical obstacles can help build public trust, while addressing public concerns can stimulate further innovation in carbon capture technologies. Together, these elements will significantly influence the future effectiveness of carbon capture and underground storage strategies.
Future Directions in Carbon Capture
The field of carbon capture is on the verge of significant advancements. Future directions in this area are essential for the development of effective and efficient CO2 reduction methodologies. As global awareness of climate change increases, focus on innovative solutions will boost progress in combating this pressing issue. Advancements in technology and collaborative efforts will be instrumental in shaping the landscape of carbon capture.
Innovations in Technology
Emerging technologies in carbon capture offer great potential. Research and development are focusing on improving capture efficiency and reducing costs. Notable innovations include:
- Direct Air Capture: This method removes CO2 directly from the atmosphere. Companies, like Climeworks, are pioneering this technology. It could play a crucial role in achieving negative emissions.
- Advanced Absorption Materials: Novel absorbents are being developed to enhance CO2 capture rates. For instance, metal-organic frameworks are showing promise due to their high surface area.
- Membrane Separation Technologies: This technique uses selective membranes to separate CO2 from other gases. Companies like Membrane Technology and Research are leading advancements in this area.
These innovations can improve the efficiency of carbon capture systems and make them more economically viable. By investing in research, it is more likely to create scalable solutions that help mitigate climate change effectively.
Integration with Renewable Energy
Renewable energy plays a crucial role in the future of carbon capture. Integrating carbon capture technologies with renewable sources can enhance the sustainability of operations. This integration offers multiple benefits:
- Reduced Carbon Footprint: Utilizing renewable energy sources, such as solar or wind, can decrease the overall carbon emissions associated with carbon capture processes.
- Energy Efficiency: The electricity generated from renewable sources can power carbon capture systems, reducing reliance on fossil fuels and improving long-term sustainability.
- Circular Carbon Economy: By combining carbon capture with renewable energy, we can create a process that continuously captures CO2 while supporting a cleaner energy future.
This synergistic approach can effectively reduce greenhouse gas emissions and support long-term climate goals.
End
In summarizing the role of carbon capture and underground storage, it is crucial to recognize the multifaceted benefits these technologies present in the fight against climate change. This article outlines that capturing carbon dioxide emissions from various sources is not merely a technical solution but a vital component of a broader sustainability strategy. Effective integration of carbon capture technology allows industries to significantly reduce their greenhouse gas emissions, making it possible to continue operations while contributing to climate goals.
Enhancing Environmental Benefits
Carbon capture paired with underground storage plays an essential role in reducing the concentrations of carbon dioxide in the atmosphere. By securely storing captured CO2 in geologically suitable formations, we can minimize its impact on global warming. Thus, the long-term effectiveness of this strategy hinges on robust geological assessments and monitoring protocols to ensure safe storage.
"Carbon capture is not a silver bullet, but rather one piece of a larger puzzle needed to combat climate change."
Considerations for Implementation
Successful implementation requires navigating various considerations, including regulatory frameworks and economic viability. As this article indicates, regulations must evolve to support and incentivize the adoption of these technologies. Economic implications, such as capital costs and operational expenses, need careful analysis to render carbon capture financially viable for industries under pressure to reduce their carbon footprints.
Future Perspectives
Looking ahead, innovations in carbon capture technologies emerge as a key focus area. As technologies advance, their integration with renewable energy sources becomes increasingly feasible. This synergy holds the potential to transform how we think about energy production and carbon emissions simultaneously.