Exploring Biological Systems and Mechanisms: A Comprehensive Overview
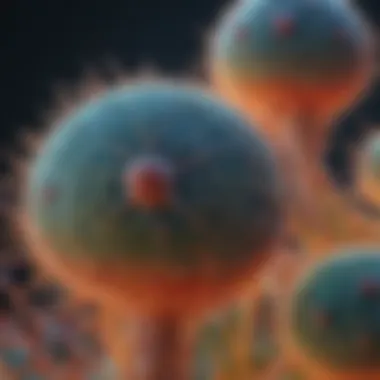
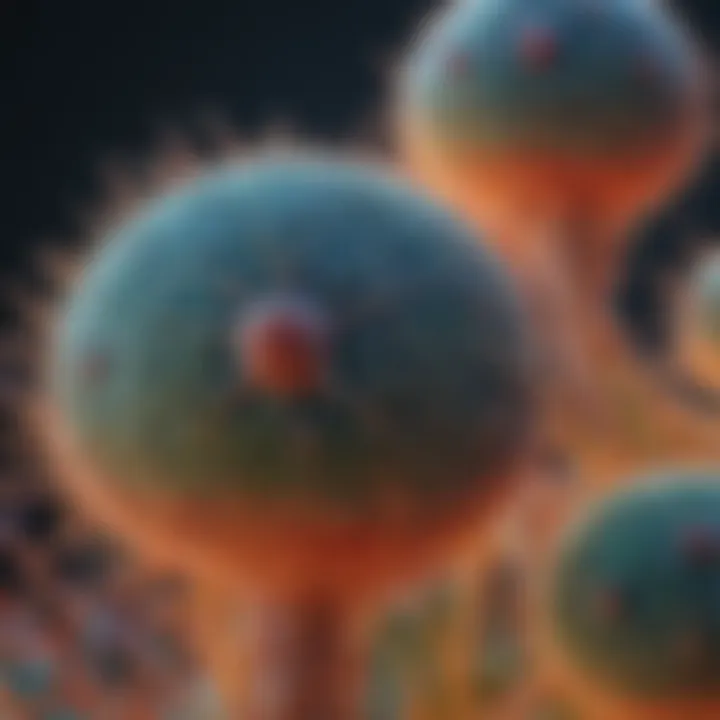
Intro
The study of biological systems and mechanisms is a multidimensional field that continues to evolve. It bridges various disciplines including cell biology, genetics, and computational science. This comprehensive overview aims to present a nuanced understanding of the structures and processes that define life.
Biological systems can be described as organized assemblies of molecules, cells, and organisms. These systems display complex interactions that help sustain life and ensure its continued evolution. Researchers have utilized various methodologies to investigate how these systems function at multiple levels, from the molecular to the ecological.
Methodologies
Description of Research Techniques
The exploration of biological systems employs a range of research techniques that are critical for the generation of quality data. The common methodologies include:
- Molecular Cloning: This technique is used to make copies of DNA fragments, which help in understanding the roles of genes.
- CRISPR-Cas9: A technology for gene editing that allows for precise alterations in the genome of organisms, facilitating studies in genetic functions.
- Proteomics: This field focuses on the large-scale study of proteins, particularly their functions and structures. This is crucial for understanding cellular mechanisms of action.
Researchers also rely on high-throughput sequencing techniques that provide insights into genomic sequence and expression patterns. These enable detailed analyses of how diverse biological systems adapt and function under varying conditions.
Tools and Technologies Used
Modern biological research heavily incorporates advanced technologies. Some vital tools include:
- Mass Spectrometry: Used for analyzing protein interactions and structures.
- Flow Cytometry: A laser-based technology for counting, sorting, and analyzing cell characteristics.
- Imaging Techniques: Methods like fluorescence microscopy and electron microscopy allow for visualization of cellular structures in real-time.
These tools significantly enhance the accuracy of data collected during research, driving a better understanding of biological mechanisms.
Discussion
Comparison with Previous Research
The relationships between the methodologies employed today and those used in earlier times illustrate significant advancements. Traditional methods focused primarily on observational techniques, whereas contemporary approaches integrate extensive computational analysis. This progress reflects a broader trend towards understanding biological systems in terms of interactions and networks rather than isolated components.
Theoretical Implications
The integration of systems biology with emerging technologies offers theoretical implications for multiple disciplines. By melding biological insights with computational models, researchers can predict how systems react to various stimuli. Such models can streamline drug development processes and environmental impact studies, enhancing the relevance of theoretical insights in real-world applications.
"Understanding the underlying mechanisms in biological systems yields a foundation for innovative applications across various fields, from healthcare to environmental science."
Overall, the examination of biological systems and mechanisms provides a rich area for research, with implications that stretch far beyond traditional biology. It invites an interdisciplinary approach that holds promise for future breakthroughs.
Prelims to Biological Systems
The concept of biological systems is at the core of understanding living organisms and their interactions with the environment. This section provides a foundation for exploring the myriad of ways life is organized, from the microscopic cellular structures to complex ecosystems. The study of biological systems enables researchers to comprehend the intricate networks that sustain life.
Defining Biological Systems
Biological systems can be defined as organized groups of interacting entities, ranging from molecules to organisms. Each system operates under specific principles of organization, function, and regulation. These systems are composed of various components, which interact dynamically to maintain homeostasis. The ability to define these systems allows scientists to categorize biological entities based on their shared characteristics.
Biological systems include:
- Cellular Systems: the basic building blocks of life
- Tissue Systems: groups of similar cells working together
- Organ Systems: collections of organs that perform a collective function
- Ecosystems: communities of living organisms interacting with their physical environment
Understanding how these systems operate highlights the complexity of biological processes. The definition provides a framework for further exploration into each component's role and importance.
Importance of Study in Biology
Studying biological systems is essential for multiple reasons. First, it helps in unraveling the complexities of nature and the interdependencies among various life forms. Recognizing these relationships leads to significant advancements in fields such as medicine, ecology, and biotechnology. Knowledge gained from biological systems can guide conservation efforts, providing insights into ecosystem management and stability.
Moreover, the study of biological systems informs about:
- Health Innovations: Discoveries in cellular mechanisms may lead to new treatments and therapies.
- Environmental Sustainability: Understanding ecological interactions can enhance conservation strategies.
- Biotechnological Advancements: Applications like synthetic biology rely on principles of biological systems to engineer organisms for specific purposes.
Historical Context of Biological Research
The history of biological research reveals a progression of thought and methodology that has shaped modern biology. From early anatomical studies in ancient civilizations to the discovery of DNA’s structure in the 20th century, various milestones mark significant advancements. Each historical phase has contributed to our current understanding of biological systems.
- Early Studies: Fundamental observations of living organisms laid the groundwork for biology.
- Development of Microscopy: With the invention of the microscope, the cellular foundation of life was unveiled, leading to cellular biology.
- Genetics Revolution: The understanding of heredity and genetic information transformed the approach towards biological research and medicine.
The historical context provides valuable lessons about the evolving nature of biological research. It highlights how each discovery builds upon previous knowledge, allowing for a more comprehensive understanding of life itself.
"The study of biological systems illuminates the interconnectedness of life, aiding in significant advancements for society."
Components of Biological Systems
Understanding the components of biological systems is essential for deciphering the complex interplay of life forms and their environments. Each system, from the cellular level to the interactions among diverse organisms, contributes significantly to the overall functioning of ecosystems. Studying these components fosters insights into the structure and behavior of living entities, impacting various fields such as medicine, ecology, and biotechnology.
Biological systems can be viewed through the lens of organization. This fosters a systematic appreciation of how entities at different levels interact, maintaining a balance that is critical to sustaining life. Insights drawn from this knowledge are applicable to vital areas, including disease management, biodiversity conservation, and biotechnological innovations.
Cells as the Basic Units of Life
Cells serve as the foundational building blocks of all living organisms. They exhibit incredible complexity and specialization, functioning as the site of various critical processes. Each cell contains organelles, each with specific roles—mitochondria for energy production, ribosomes for protein synthesis, and the nucleus for housing genetic materials.
Every living organism, whether a single-celled bacterium or a multicellular mammal, relies on cells' functionality. They not only replicate and sustain life but also communicate with each other through signaling pathways. The understanding of cellular processes is pivotal, offering insights into mechanisms of disease, development, and aging. Cells also respond to external stimuli, adapting to changes in their environment, which are essential for survival.
Tissues and Organ Systems
Tissues are groups of similar cells working together to perform a specific function. They can be classified into four primary types: epithelial, connective, muscle, and nervous tissues. Each tissue type plays a crucial role in maintaining the body's integrity and functionality. For instance, muscle tissue facilitates movement, while connective tissue provides structural support.
Organ systems are a collective of organs that work in unison to execute complex functions. For instance, the respiratory system is responsible for gas exchange, while the digestive system processes food for nutrients. Understanding the configuration and interdependence of these systems is vital in both health and disease. Anomalies in tissue or organ function can lead to a cascade of effects that disrupt the body's homeostasis, prompting the need for medical intervention and research.
Organisms and Ecosystem Interactions
Each organism plays a distinct role within an ecosystem. Producers, consumers, and decomposers represent the dynamic interactions among various species. Producers, such as plants, are crucial as they convert solar energy into chemical energy through photosynthesis, forming the base of the food chain. Consumers, which include herbivores and carnivores, contribute to energy transfer within ecosystems.
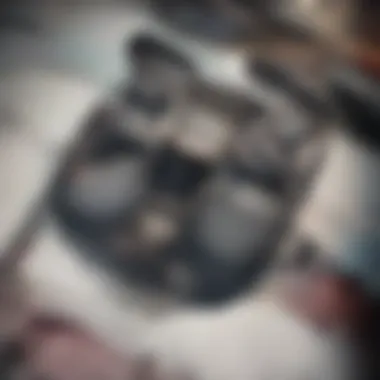
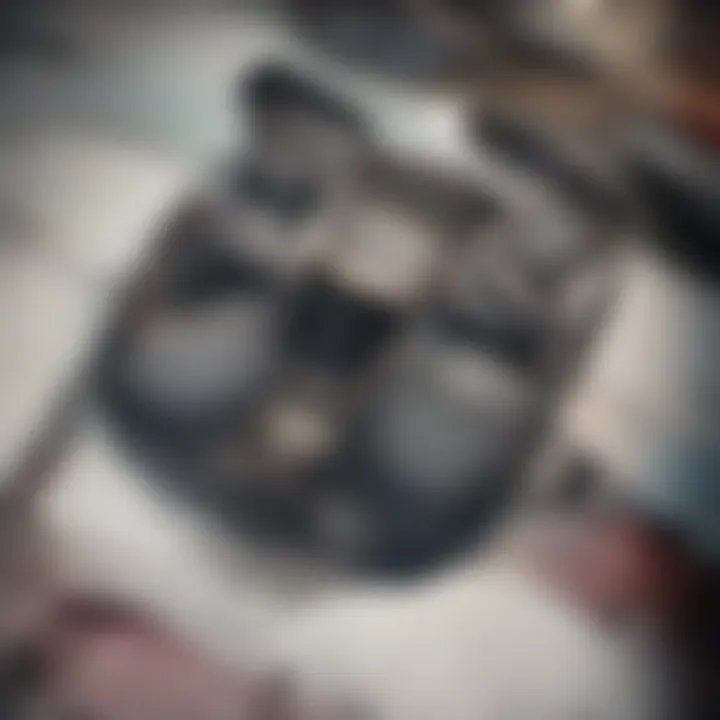
Furthermore, decomposers recycle nutrients back into the environment, supporting plant growth. The interactions among these organisms and their environments define ecosystem health and functionality. Understanding these relationships is essential for ecological conservation efforts, biodiversity assessment, and managing natural resources effectively.
"Understanding biological systems requires a holistic approach that considers all levels of organization, from cells to ecosystems."
Molecular Mechanisms in Biology
Molecular mechanisms are fundamental to understanding the processes that dictate life at a cellular level. They provide insights into how cells function, communicate, and respond to changes in their environment. This section elucidates the various types of biomolecules and their contributions to biological systems. Understanding these mechanics is crucial for advancements in research and technology in biology.
The Role of Biomolecules
Biomolecules are the building blocks of life. They encompass proteins, nucleic acids, lipids, and carbohydrates, each playing pivotal roles in cellular function and maintaining life.
Proteins
Proteins are complex molecules made up of amino acids. Their structure is directly linked to their function, acting as enzymes, hormone regulators, and structural components. They initiate and regulate biochemical reactions within the cell. One distinctive characteristic of proteins is their ability to fold into intricate shapes, which determine their functionality. This property makes proteins a beneficial choice in understanding molecular mechanisms. They are essential for catalyzing metabolic reactions and facilitating cellular communication. However, the complexity in their folding can also make them sensitive to environmental changes, which may lead to loss of function.
Nucleic Acids
Nucleic acids come in two forms: DNA and RNA. They store and transmit genetic information. DNA serves as a blueprint for the synthesis of proteins through processes such as transcription and translation, which is critical for gene expression. Nucleic acids are highly stable molecules, making them a popular choice in biological research and biotechnology. Nevertheless, they can be subject to mutations, which can affect genetic information and lead to various diseases.
Lipids
Lipids perform several vital functions, including forming cellular membranes and acting as energy storage molecules. Their unique structure allows them to be hydrophobic, which is crucial for forming cell membranes that separate cellular compartments. This characteristic makes lipids a valuable component of biological systems. They facilitate signaling processes through molecules like hormones. However, their variability in structure can complicate their study, particularly in understanding their roles in metabolism and health.
Carbohydrates
Carbohydrates are primarily energy sources in biological systems. They are categorized into simple and complex forms, such as sugars and starches. One key feature of carbohydrates is their ability to form structural components, like cellulose in plants. This makes carbohydrates a prominent choice when discussing energy storage and supply. They also play roles in cell recognition and signaling. However, their rapid metabolism can sometimes lead to fluctuations in energy regulation, which can impact cellular functions.
Gene Expression and Regulation
Gene expression and regulation involve complex interactions between DNA, RNA, and proteins. These processes control the amount and timing of protein production, ensuring that the right proteins are made when needed. Understanding these dynamics is essential as it helps researchers determine how cells adapt to various conditions, which has implications in areas like cancer research and developmental biology.
Enzymatic Reactions and Metabolism
Enzymatic reactions are vital for metabolism, contributing to how cells convert substrates into energy. Enzymes function as catalysts, speeding up chemical reactions that would otherwise occur too slowly. Understanding metabolic pathways can aid in developing treatments for metabolic disorders and improving metabolic health.
Systems Biology Approaches
Systems biology represents a remarkable shift in how biological research is conducted, integrating various biological data with computational models. This interdisciplinary field is crucial as it allows for the study of complex interactions within biological systems rather than examining individual components in isolation.
Understanding Biological Interactions
Understanding biological interactions is essential in predicting how different elements of a biological system respond to changes. Cells, tissues, and organ systems do not function in isolation. Their interactions are characterized by a network of biochemical pathways. These pathways often involve feedback loops and can exhibit non-linear behaviors, making them challenging to study with traditional methodologies. Systems biology adopts a holistic view, enabling researchers to explore these interactions in detail.
By utilizing high-throughput data generation techniques, systems biology can unveil the intricate relationships within various biological systems. This framework can lead to new insights into disease progression and other biological phenomena that may not be evident through reductionist approaches.
Integration of Computational Models
The integration of computational models has transformed systems biology into a powerful tool for interpreting biological data. These models simulate complex biological processes, allowing researchers to visualize and predict cellular behavior under different conditions. The models range from simple mathematical representations to complex simulations involving artificial intelligence.
Such quantitative analysis enhances understanding of cellular functions and interactions at both micro and macro levels. It also facilitates the validation of experimental results. As different models can be created and modified easily, researchers can conduct "what-if" scenarios to assess potential outcomes of different biological interventions. As a result, there’s a marked improvement in efficiency and accuracy of biological research.
Applications of Systems Biology
- Disease Modeling: Disease modeling within systems biology allows for a comprehensive understanding of complex diseases. Researchers can create models that simulate disease progression, providing insights into mechanisms behind conditions like cancer and diabetes. This approach is beneficial as it aids in identifying potential therapeutic targets and predicting treatment responses. A significant characteristic of disease modeling is its ability to synthesize information from various sources, enhancing the overall understanding of pathology. However, results can vary due to the complexity of biological systems and the variability inherent in biological data.
- Synthetic Biology:
Synthetic biology focuses on designing and constructing new biological parts and systems. It is an essential element of systems biology as it pushes the boundaries of biological functions. Researchers can engineer organisms with new capabilities, leading to advancements in biofuel production, pharmaceuticals, and agricultural practices. The key benefit of synthetic biology is its ability to create novel functionalities not found in nature. However, ethical concerns surrounding genetic modifications pose challenges in its application and public acceptance. - Pharmacogenomics:
Pharmacogenomics is the study of how genes affect individual responses to drugs. This application of systems biology holds promise for personalized medicine approaches. By understanding genetic variations, treatments can be tailored to individuals, improving efficacy and reducing adverse effects. The primary characteristic of pharmacogenomics is its focus on the interactions between drugs and genetic makeup, making it a highly relevant and beneficial area of study. Nevertheless, challenges include the complexity of gene-drug interactions and the need for extensive validation of findings before clinical applications are made.
The integration of systems biology into these fields not only improves our understanding but also paves the way for innovative solutions in health care and biotechnology.
Experimental Techniques in Biological Research
The study of biological systems greatly relies on experimental techniques. These methodologies are critical for understanding complex biological processes. They allow scientists to manipulate variables and observe outcomes, thus providing insights into cellular functions and interactions. Each method serves unique purposes, making them essential tools in biological research. This section evaluates significant experimental techniques that scientists employ today, including molecular cloning, CRISPR gene editing, and high-throughput screening.
Molecular Cloning Techniques
Molecular cloning is a fundamental technique in biology. This process involves creating copies of specific DNA fragments. By doing this, researchers can study genes and proteins in detail. Molecular cloning allows for the introduction of new genetic material into organisms. This contributes significantly to genetic engineering and functional genomics.
Some techniques in molecular cloning include the use of restriction enzymes and vectors. Restriction enzymes cut DNA at specific sequences. This precision enables scientists to insert genes of interest into plasmid vectors. Plasmids are circular DNA used to propagate genetic material inside host cells.
The advantages of molecular cloning include:
- Targeted gene study: Scientists can focus on individual genes.
- Protein production: Cloning allows for large-scale production of proteins, important for therapeutic applications.
- Gene therapy research: It facilitates the exploration of genetic diseases through precise manipulation.
However, there are also considerations. Researchers need to ensure that the cloned genes are expressed properly in host organisms. Selecting appropriate vectors and hosts is crucial.
CRISPR and Genome Editing
CRISPR, or Clustered Regularly Interspaced Short Palindromic Repeats, represents a transformative approach in genome editing. This tool provides a precise method for altering gene sequences within organisms. It functions through a guide RNA that directs the Cas9 enzyme to specific locations in the DNA. Once there, Cas9 can cut the DNA, enabling various modifications, such as inserting or deleting sequences.
The impact of CRISPR in biological research is profound. Its uses include:
- Gene knockout experiments: This helps in studying gene function by observing the effects of gene loss.
- Disease models: CRISPR can create models for genetic diseases, aiding in research.
- Agricultural advancements: It allows for genetic improvements in crops, offering better yields.
Nonetheless, ethical considerations surround CRISPR. Concerns include unintended genetic consequences and implications in human genome editing. Researchers must navigate these issues carefully.
High-Throughput Screening
High-throughput screening (HTS) is a method that enables the simultaneous testing of thousands of compounds for biological activity. This technique is critical for drug discovery. HTS allows researchers to identify promising candidates rapidly, streamline the drug development process, and minimize costs.
HTS relies on automation and advanced technologies, such as robotics and data analysis software. This efficiency enhances the discovery of new pharmacological agents. Its applications include:
- Lead compound identification: Screening libraries of compounds helps find potential drugs.
- Biochemical assay development: Researchers can assess how compounds interact with biological targets.
- Phenotypic screening: Testing compounds on live cells allows observation of complex biological responses.
Although HTS is powerful, it requires extensive data analysis. The results must be interpreted carefully, as false positives can lead to erroneous conclusions.
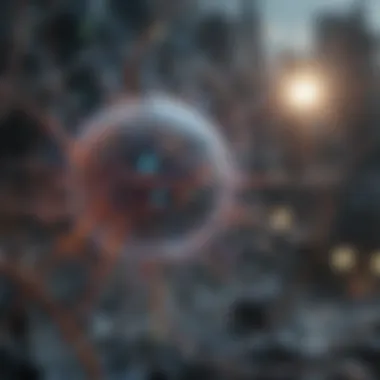
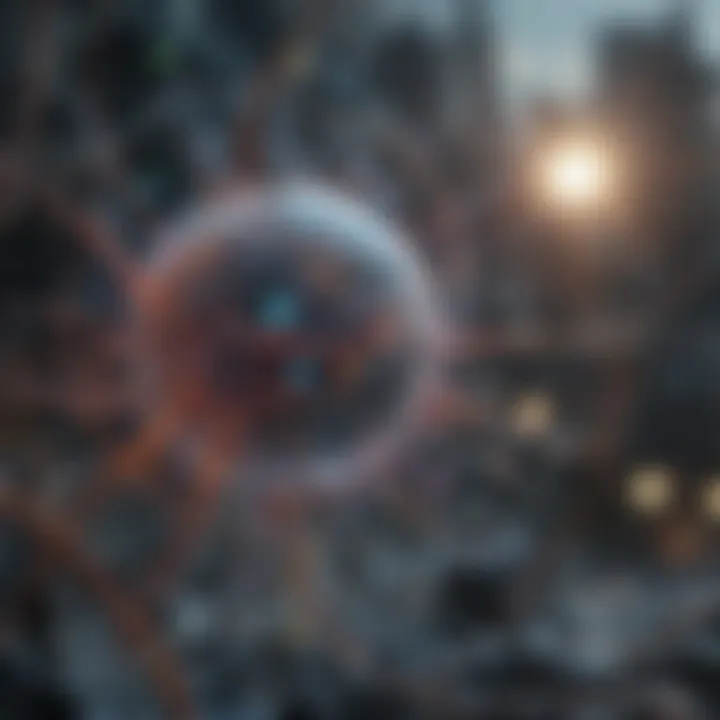
"The integration of advanced experimental techniques is vital to pushing the boundaries of biological research, transforming our understanding of life's mechanisms."
Emerging Trends in Biological Mechanisms
Emerging trends in biological mechanisms signify a significant leap in our understanding of various biological processes. The convergence of technology and biology has led to groundbreaking innovations, making it essential for researchers and professionals to stay updated on these developments. The integration of advanced tools and methodologies allows for a more nuanced study of biological systems. This section highlights the importance of new technologies and methods that contribute to the enhancement of biological research, offering numerous benefits in understanding complex biological systems.
Synthetic Biology Innovations
Synthetic biology emerges as one of the most exciting trends in biological research. It involves the design and construction of new biological parts, devices, and systems. This field aims to make biological research more predictable and efficient, thus pushing the boundaries of what can be achieved. Innovations in synthetic biology can lead to the production of biofuels, pharmaceuticals, and other bio-based materials through engineered organisms.
- Key techniques include the use of modular genetic elements, which can be assembled in various configurations to create desired functions.
- Examples of synthetic biology applications are seen in the development of microbial factories that can convert waste into valuable resources.
- Ethical considerations must also be addressed, as synthetic organisms are created, raising questions about their potential impact on natural ecosystems.
These innovations can transform industries and offer sustainable alternatives to traditional methods of production.
Biological Data Analytics
The ever-increasing amount of biological data necessitates sophisticated analytical techniques. Biological data analytics combines statistics, computer science, and biology to extract meaningful insights from vast datasets. With advancements in data collection methods, such as high-throughput sequencing, the capability to analyze complex biological information has improved significantly.
- Approaches in this field include machine learning algorithms that can predict biological outcomes based on genetic data.
- Benefits involve enhanced understanding of diseases, leading to improved diagnostic and therapeutic strategies.
- Challenges remain, particularly in ensuring data quality and establishing standardized methods for analysis.
Overall, data analytics represents a vital tool for interpreting the complexities of biological systems.
Nanotechnology in Biology
Nanotechnology is making a profound impact in various fields, including biology. It involves manipulating materials at the nanometer scale, which is about one-billionth of a meter. In biology, this technology is used for drug delivery, imaging, and even in developing new diagnostic tools. Nanoparticles can be designed to target specific cells, thereby improving the efficacy of treatment.
- Applications include targeted cancer therapy, where nanoparticles deliver drugs directly to tumor cells, minimizing damage to healthy tissue.
- Research in this area contributes to better understanding cellular processes at the nanoscale, opening doors for innovative treatments.
- Regulatory issues also arise, as the safety and long-term effects of nanoparticles in medical applications need careful evaluation.
Through these emerging trends, the field of biology is evolving rapidly, paving the way for novel applications and a deeper understanding of life itself. The intersection of technology and biology creates a promising horizon for researchers and practitioners alike.
"Staying abreast of these emerging trends is crucial for anyone involved in biological research."
As we navigate this evolving landscape, the implications for future studies in biological mechanisms are profound.
Case Studies in Biological Research
Case studies in biological research serve as a cornerstone for practical applications in the field. They allow researchers to investigate complex biological systems in real-world contexts and provide insights that laboratory studies may not cover. This section emphasizes the significance of case studies for understanding biological mechanisms and emphasizes their utility in both academic and clinical settings.
Model Organisms in Research
Model organisms play a critical role in biological research. These organisms provide simplified systems that can be used to answer complex questions in biology. Understanding their advantages can significantly enhance the scope of research, leading to potential breakthroughs.
Drosophila
Drosophila melanogaster, commonly known as the fruit fly, is a widely used model organism. Its rapid life cycle and genetic similarity to humans make it invaluable for genetic studies. One key characteristic of Drosophila is its manageable size and the ease of handling in a laboratory setting. Researchers can produce offspring quickly, facilitating large-scale genetic experiments.
A unique feature of Drosophila is its well-characterized genome, which has been sequenced and extensively annotated. This characteristic allows for precise genetic manipulation, making it easier to observe the effects of specific genes. However, one disadvantage could be its limited ability to replicate certain mammalian system phenomena. Nonetheless, its contributions to genetics and developmental biology remain unparalleled.
Mouse Models
Mouse models represent another cornerstone in biological studies. Mus musculus is highly favored due to its anatomical and physiological similarities to humans. This similarity allows researchers to draw more relevant conclusions regarding human health and disease processes. A significant aspect of mouse models is their capacity for genetic modification, a feature often utilized in studying disease pathogenesis.
One unique attribute of mouse models is their capacity to mimic human diseases, such as diabetes and cancer, facilitating the development of therapeutic strategies. While the manipulation of genetic backgrounds is a powerful tool, ethical considerations regarding their use do raise some concerns. Nevertheless, research involving mouse models yields substantial insights that are beneficial for advancements in medicine.
Zebrafish
Zebrafish, or Danio rerio, have become increasingly prevalent in biological research due to their transparency and rapid development. One notable characteristic is their external fertilization and developmental accessibility, allowing observation of embryonic development in real-time. The advantages of using zebrafish include the ability to conduct high-throughput screening of drugs and genetic mutations.
Zebrafish also possess a unique feature in their regenerative capabilities, which presents opportunities to study healing and tissue regeneration processes. Nonetheless, their use can sometimes be limited in organismal complexity, as the results may not directly translate to mammalian biology.
Field Studies and Their Impact
Field studies provide significant real-world data that laboratory experiments cannot replicate. These studies are crucial for understanding species interactions, population dynamics, and ecological relationships. The insights gained from field studies inform conservation efforts and ecological management. They help bridge the gap between theoretical models and practical applications.
Clinical Trials and Translational Research
Clinical trials are a critical step for validating biological discoveries in patient populations. They transform basic research into applicable treatments, providing crucial data on safety and effectiveness. Translational research serves as a process to apply findings from basic science to enhance human health. This segment of research often highlights the importance of collaboration across disciplines to drive innovation and improve patient outcomes.
Interdisciplinary Connections
Interdisciplinary connections in biological research play a critical role in enriching our understanding of complex biological systems. By integrating knowledge and methods from diverse fields, researchers can approach problems with a more comprehensive perspective. This synergy between disciplines enhances both theoretical frameworks and practical applications in biology.
Biology and Chemistry
The relationship between biology and chemistry is foundational to understanding biological processes. Biological systems are fundamentally chemical in nature. The behavior and interactions of biomolecules, such as proteins and nucleic acids, are best explained through chemical principles.
Key points in this interplay are:
- Biochemical Pathways: Understanding these pathways requires knowledge of chemical interactions. Enzymes, for example, act as catalysts to speed up chemical reactions essential for cellular functions.
- Drug Development: The field of medicinal chemistry highlights how chemical knowledge can lead to the creation of more effective pharmaceuticals. Understanding how drugs interact at the molecular level can improve treatment efficacy and reduce side effects.
In summary, chemistry provides the tools necessary to explore the molecular basis of life, making it indispensable in biological research.
Biology and Physics
Physics plays an equally significant role in biological systems. Many biological processes can be understood through the principles of physics, such as thermodynamics and mechanics. The application of these principles leads to new insights into how organisms function.
Important aspects include:
- Biophysics: This subfield explores the physical principles underlying biological systems. For instance, studying the mechanics of cell division informs our understanding of cancer biology.
- Biomechanics: Understanding how organisms move relies on physics. The design of bioinspired technologies, like robotic limbs, shows how insights from biology and physics can create real-world applications.
The collaboration between biology and physics deepens our comprehension of the physical limits and capabilities of living organisms.
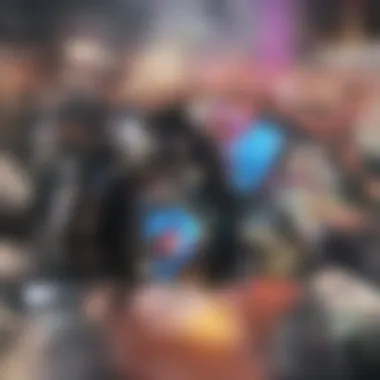
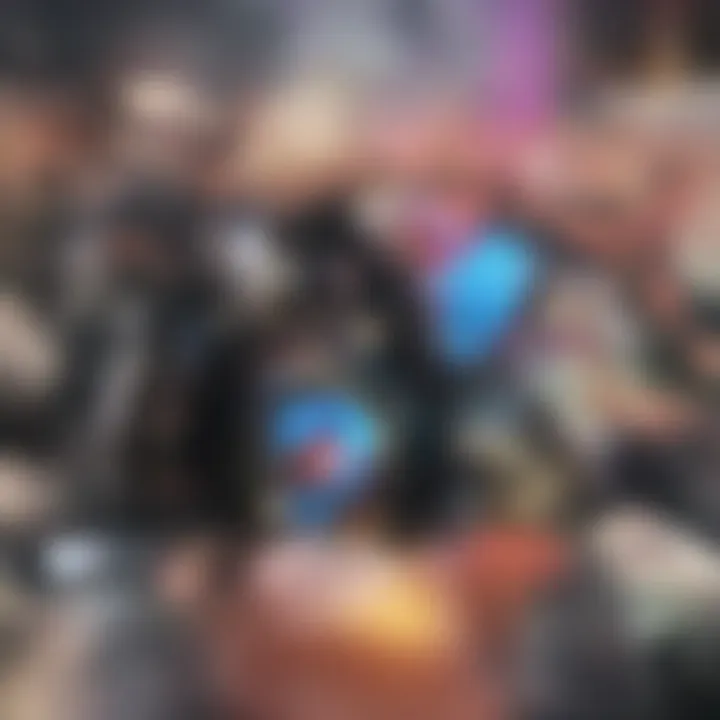
Biology and Computer Science
As technology evolves, the relationship between biology and computer science becomes increasingly crucial. Bioinformatics, a discipline that merges these two fields, emphasizes the analysis of biological data through computational tools.
Considerations in this connection are:
- Data Processing: The analysis of large datasets, such as genomic sequences, requires advanced computational methods. Knowledge of algorithms and software development is essential for modern biological research.
- Modeling Biological Systems: Computer simulations model complex biological processes, allowing researchers to predict behaviors that are difficult to observe directly in nature.
Ultimately, the integration of computer science enhances our ability to understand and manipulate biological information, leading to innovations in research and applications.
The interdisciplinary approach in biology fosters a richer understanding of complex systems and provides innovative solutions to scientific challenges.
Ethical Considerations in Biological Research
As biological research continues to evolve, it becomes increasingly necessary to address the array of ethical considerations that arise within this field. Ethical considerations in biological research encompass a range of issues, from animal welfare to the implications of genetic engineering and environmental effects. Engaging with these topics is not merely an exercise in compliance; it is crucial for fostering responsible scientific practice and public trust in research activities. The careful navigation of these ethical boundaries ultimately enhances the integrity and quality of biological studies.
Research Ethics and Animal Welfare
The treatment of animal subjects in biological research is a pivotal ethical concern. Researchers must uphold the principle of humane treatment, ensuring that animals are not subjected to unnecessary suffering. The Three Rs Principle—Replacement, Reduction, and Refinement—serves as a guiding framework:
- Replacement: Where possible, the use of alternative methods to replace live animals in research should be prioritized.
- Reduction: When animal use is unavoidable, researchers should minimize the number of animals used.
- Refinement: Procedures should be designed to minimize pain, suffering, or distress experienced by the animals.
This ethical obligation is not only rooted in compassion but also in legality, as many countries enforce strict regulations governing animal research. Fostering a culture of ethical consideration in this area will ultimately lead to more responsible and reliable research outcomes.
Genetic Engineering Ethics
Genetic engineering presents a unique array of ethical challenges that require careful scrutiny. Advances in technologies such as CRISPR have made it possible to edit genes with unprecedented precision and ease. However, this capability raises questions about the limits of human intervention in natural processes.
Among the primary concerns are:
- Long-term effects: Unintended consequences of genetic modifications can affect entire ecosystems or population dynamics.
- Human enhancement: The potential for genetic engineering to be used for non-therapeutic enhancements creates a moral dilemma about equity and access.
- Genetic discrimination: There are fears that genetic modifications could lead to forms of discrimination in social and economic contexts.
These ethical questions necessitate comprehensive discussions involving scientists, ethicists, policymakers, and the public to navigate the intricate landscape of genetic engineering responsibly.
Environmental Impact Assessments
With biological research increasingly intersecting with environmental concerns, it is essential to incorporate ethical considerations into environmental impact assessments. Understanding the potential ecological consequences of biological activities is vital for sustainable research practices. Researchers must evaluate:
- Impact on biodiversity: Activities like genetic modification of crops can lead to changes in local biodiversity, potentially harming native species.
- Ecosystem health: Assessing how research practices influence ecosystem dynamics helps prevent harmful environmental consequences.
- Community engagement: Involving local communities in discussions about environmental implications ensures that diverse viewpoints are considered.
By prioritizing ethical considerations in biological research, we not only protect lives and environments but also pave the way for responsible scientific progress.
Future Directions in Biological Research
The field of biological research is rapidly evolving, with advancements paving the way for innovative approaches and novel solutions to complex problems. Understanding these future directions is crucial. It reflects the ever-changing landscape of scientific inquiry and underscores the importance of staying informed about new developments.
Advancements in Biotechnology
Biotechnology stands at the forefront of biological research. Techniques such as CRISPR genome editing revolutionize how scientists approach genetics. These methods allow for precise modifications of DNA, offering potential cures for genetic diseases. Moreover, synthetic biology enables the design of new biological parts and systems. This innovation raises significant questions about the role of engineered organisms in our ecosystems.
"Biotechnology allows us to manipulate biological systems in ways previously thought impossible."
The role of biotechnology extends beyond medicine. It influences agriculture through genetically modified crops that can withstand pests and environmental stresses. This leads to greater food security in an increasingly populated world. However, it is essential to balance advancements with ethical considerations related to bioconcerns and public acceptance.
Personalized Medicine Approaches
Personalized medicine represents a shift from traditional one-size-fits-all healthcare to more tailored treatment strategies. By integrating genetic information with patient data, healthcare providers can offer customized therapies. This is particularly beneficial in treating cancers, where individual tumor profiles can guide treatment decisions.
The importance of bioinformatics arises here. Analyzing large datasets enables researchers to identify patterns and markers that inform treatment plans. However, challenges remain. Ethical concerns regarding data privacy and equal access to these advancements must be addressed to ensure fairness in personalized healthcare.
Sustainability in Biological Research
Sustainability is gaining prominence in biological research. The impact of research activities on ecosystems and biodiversity is under scrutiny. Researchers must consider the environmental ramifications of their work. This includes reducing waste in laboratory processes and emphasizing green chemistry principles.
Strategies for sustainable research include:
- Utilizing renewable energy sources in laboratories.
- Developing biodegradable materials for experiments.
- Implementing efficient waste disposal practices.
Understanding the sustainable practices can lead to more responsible scientific endeavors. This not only aids in the preservation of natural resources but also aligns biological research with global sustainability goals.
The End
The conclusion of this article serves to encapsulate the core insights derived from exploring biological systems and mechanisms. It is vital to recognize how interconnected and complex these systems are, reflecting the multitude of processes at work in natural environments. Understanding biological systems is not merely an academic exercise; it has far-reaching implications for numerous fields, including medicine, ecology, and biotechnology.
Key findings have highlighted the significance of cellular structures and the molecular mechanisms that underpin life. Proteins, nucleic acids, lipids, and carbohydrates play distinct yet interrelated roles. The systems biology approach, integrating computational models, stands as a revolutionary way to interpret these complexities, allowing for more accurate predictions in biological behavior.
Moreover, this article points to the crucial transition toward personalized medicine and sustainable practices in research. The ethical considerations discussed emphasize that as we gain insight into biological mechanisms, we must proceed with caution to ensure the welfare of both research subjects and the environment.
Summary of Key Findings
- Complex Interactions: Biological systems are characterized by intricate interactions between their components, affecting overall functionality.
- Molecular Role: The primary biomolecules—proteins, nucleic acids, lipids, and carbohydrates— each contribute fundamentally to biological processes.
- Systems Biology: This interdisciplinary approach merges biology with computational sciences, enhancing our capacity to understand and model biological systems.
- Innovations in Techniques: Advancements such as CRISPR have transformed genetic research, showcasing the rapid progress in methodologies.
- Ethical Dimensions: As research progresses, ethical implications surrounding animal welfare and environmental impacts remain of utmost importance.
Implications for Future Research
Future research must focus on several pivotal areas:
- Interdisciplinary Collaboration: Collaborations between biology and fields such as computer science and engineering can yield innovative solutions to biological challenges.
- Personalized Approaches: Continued exploration in personalized medicine will address unique patient needs, enhancing treatment efficacy.
- Sustainability Practices: Integrating sustainability in biological research is crucial to mitigating environmental impact while advancing knowledge.
- Ethical Considerations: An ongoing assessment of ethical guidelines will promote responsible research practices, balancing scientific advancement with moral responsibility.
Adopting these strategies could lead to significant breakthroughs in our understanding of biological systems, ultimately enhancing the quality of life and preserving the integrity of ecosystems.
Importance of Citing Relevant Literature
- Academic Credibility: Proper citations enhance the credibility of research work. Researchers demonstrate a thorough investigation of existing literature, thus validating their findings.
- Knowledge Dissemination: Citing works encourages the broader distribution of knowledge. By referencing pivotal studies, scientists facilitate access to valuable insights for their peers.
- Avoiding Plagiarism: Recognizing sources appropriately ensures that original authors receive due credit, safeguarding researchers from ethical pitfalls like plagiarism.
- Establishing Context: Literature helps situate new research within established scientific paradigms, aiding in the interpretation of results.
It is essential to follow established citation styles relevant to biological research, such as APA, MLA, or CSE. Each of these styles has specific rules regarding how references should be formatted, serving to standardize the presentation of academic work.
"Good research does not occur in a vacuum. It is a dialogue with previous work that inspires further inquiry."
Utilizing databases such as PubMed, Google Scholar, and others, researchers can access a plethora of articles relevant to their field. This access to literature allows for a more nuanced understanding of ongoing debates and advancements in biological systems.