Exploring Angiogenesis Tube Formation Assay
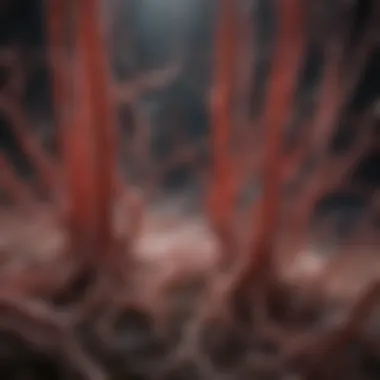
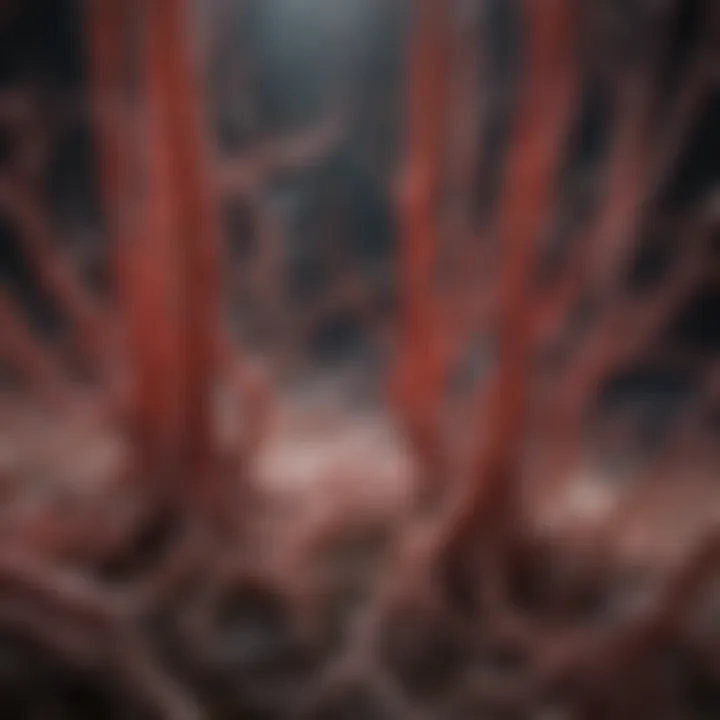
Intro
The investigation of angiogenesis, the intricate process of new blood vessel formation, has gained considerable attention for its significance in both health and disease. One of the most employed techniques to study this phenomenon in vitro is the angiogenesis tube formation assay. This method provides researchers with a powerful tool to observe and measure the capability of endothelial cells to form tubular structures that mimic blood vessels. By capturing the dynamics of this process, scientists can glean insights into various physiological conditions, ranging from developmental biology to pathologies such as cancer and cardiovascular diseases.
Throughout this article, we will delve into the methodologies underpinning this assay, explore the specific tools and technologies utilized, and discuss the theoretical implications of the findings. The focus will be on creating a holistic understanding of how the angiogenesis tube formation assay can illuminate the pathways of blood vessel development, as well as its broader implications across various fields of research.
Methodologies
In the realm of experimental biology, understanding the mechanics of angiogenesis necessitates a clear grasp of the methodologies employed in tube formation assays. These methodologies often determine the reliability and validity of the findings derived from such experiments.
Description of Research Techniques
The angiogenesis tube formation assay typically begins with the utilization of a matrix, such as Matrigel, which provides a favorable environment for endothelial cells to grow and interact. These cells are then seeded onto the gel in a controlled manner. Over the course of several hours to days, researchers monitor and quantify the formation of tube-like structures that emerge. This dynamic process can be influenced by various factors, including growth factors and cytokines, which are often introduced into the culture media to mimic the physiological conditions that promote angiogenesis.
Another noteworthy technique involves live-cell imaging, allowing for real-time observation of the morphogenic changes that endothelial cells undergo. This can provide substantial insights into the timing and spatial organization of the tube formation process.
Tools and Technologies Used
To perform the angiogenesis tube formation assay successfully, several tools and technologies are essential:
- Matrigel or Collagen Gel: Serves as a scaffold for endothelial cell growth, providing the necessary extracellular matrix (ECM) components.
- Incubator with Controlled Atmosphere: Maintains conditions conducive to cell survival and function, typically involving stable temperature, humidity, and COā levels.
- Fluorescent Microscopy: Supported by imaging software, this is used for detailed visualization and analysis of tube formation.
- Flow Cytometry: Sometimes utilized for analysis of cell populations and their responses to various stimuli.
By comprehensively employing these tools, researchers can gather pertinent data that contributes to understanding blood vessel formation.
"Angiogenesis is not just about making new blood vessels; it is a dance of cells and signals that shapes how tissues receive nutrients and oxygen."
Discussion
Comparison with Previous Research
The angiogenesis tube formation assay has evolved from earlier methodologies focused solely on quantitative analysis. Modern approaches, which include advances in imaging and live-cell tracking, have helped scientists compare and contrast findings across multiple studies. Previous research often lacked the nuanced understanding that contemporary techniques now bring to the forefront, allowing for a richer interpretation of how various factors contribute to blood vessel development.
Theoretical Implications
The insights gained from the angiogenesis tube formation assay not only enrich basic research but also carry implications for translational studies. Understanding the mechanisms governing angiogenesis is instrumental for therapeutic interventions in diseases characterized by abnormal blood vessel formation. Therefore, the exploration of the assay provides a critical bridge between laboratory findings and clinical applications.
Preface to Angiogenesis
Angiogenesis is the biological process through which new blood vessels form from pre-existing vessels. This intricate process is not just a fascinating aspect of vascular biology; it serves as a fundamental mechanism that sustains life. Understanding angiogenesis allows researchers to unlock answers to various biological enigmas and disease states. When we delve into our topicāangiogenesis tube formation assaysāwe recognize these assays as pivotal tools in studying this complex phenomenon. They enable scientists to visualize and quantify the processes involved in blood vessel formation, making them indispensable for both basic research and clinical applications.
This introduction provides a roadmap for grasping the significance of angiogenesis in normal physiological functions and its role in diseases. You might be asking why is this relevant? To put it plainly, it all boils down to how essential blood vessels are for transporting oxygen and nutrients, not only in healthy tissue but also in variable states of disease. A clear understanding of angiogenesis paves the way for innovative treatments in diverse fields like cancer, diabetes, and cardiovascular diseases.
As we proceed, we will discuss the definition of angiogenesis, emphasizing its mechanisms and impact on various bodily systems.
Overview of Angiogenesis Assays
Angiogenesis assays serve as a cornerstone in the exploration of blood vessel formation, shedding light on how new vessels originate from existing ones. This is not merely important for understanding oxygen delivery to tissues but also plays a crucial role in various biological processes, such as wound healing and tumor growth. Within this section, weāll unravel the nuances of angiogenesis assays in terms of their types, strengths, and limitations.
Types of Assays
Various methodologies are available to study angiogenesis, each with inherent strengths and drawbacks based on the context of their application. Below, weāll discuss three fundamental types of assays: ex vivo, in vitro, and in vivo models, exploring their relevance and unique contributions to angiogenesis research.
Ex Vivo Models
Ex vivo models involve using tissues removed from an organism to investigate angiogenic processes. One key characteristic of these models is that they maintain a more natural physiological environment compared to isolated cells. For instance, a common method involves using aorta rings from rodents, where researchers can observe the sprouts forming in a controlled setting, allowing them to replicate natural conditions.
Having said that, these models are generally regarded as beneficial because they allow for interactions between various cell types and the extracellular matrix that wouldn't occur in pure cell culture. The unique feature of ex vivo models lies in their ability to retain the original architecture of blood vessels, making them particularly good at mimicking in vivo conditions. However, one disadvantage is that they tend to be time-consuming, and the variability can be high due to the differences between specimens.
In Vitro Models
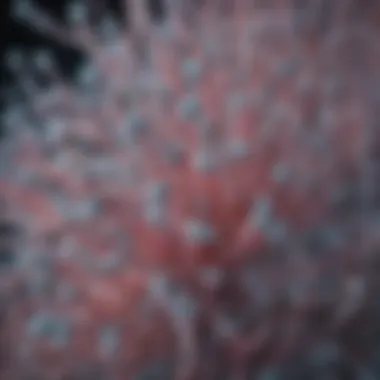
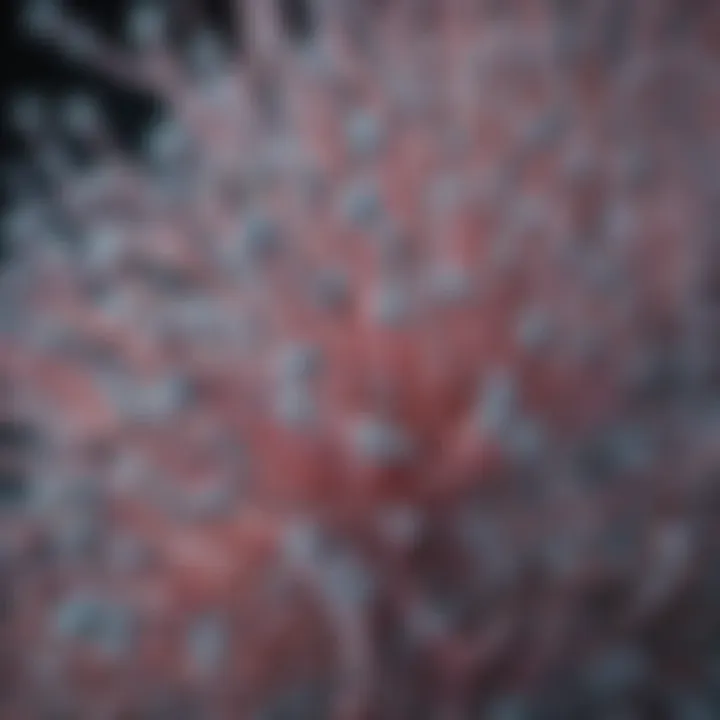
In vitro models focus on studying cells in a dish, employing established endothelial cell lines or primary cells to examine angiogenesis. A standout feature of in vitro methods is simplicity ā they allow for easy manipulation of experimental conditions and controlled environments, enabling researchers to change one variable at a time.
These models are popular for screening drugs' effects on angiogenesis, thanks to their reproducibility and lower costs. For instance, assays like the Matrigel tube formation assay enable a straightforward observation of how endothelial cells form tube-like structures. However, one major limitation is that they may not fully capture the complexity of vessel formation found in living organisms. The lack of a supportive microenvironment can lead to results that do not translate well into realistic scenarios.
In Vivo Models
The in vivo models take the study of angiogenesis up a notch by employing live organisms, which introduces a wealth of biological complexity. Researchers often use mouse models to investigate how novel therapies might influence angiogenesis within the context of a living organism. The key characteristic of in vivo models is their capability to represent the full spectrum of biological interactions.
These models are incredibly beneficial for understanding how angiogenesis occurs in disease contexts, such as cancer. They allow for real-time assessment of therapeutic effects and are essential for validating findings from in vitro studies. The unique feature of in vivo models is their biological relevance, including physiological responses, systemic influences, and the interaction of the tumor microenvironment with blood vessels. Nevertheless, they come with their own set of disadvantages, such as ethical concerns, prohibitive costs, and the inherent variability in living systems.
Strengths and Limitations
Understanding the strengths and limitations of various assays allows researchers to choose the most fitting method depending on their specific research questions.
- Ex Vivo Strengths: Closer to physiological conditions, allow assessment of multiple cell types.
- In Vitro Strengths: Control over experimental variables; low cost and high reproducibility.
- In Vivo Strengths: Represents real biological processes with interactions across systems.
- Limitations: Time-consuming; specimen variability can skew results.
- Limitations: Lack of complexity; results may not reflect in vivo contexts.
- Limitations: Ethical concerns; costs are higher; substantial variability.
In sum, each assay type brings unique insights into the angiogenesis process. The careful selection of the right model is vital based on the questions being addressed and the resources available. With these understandings, researchers can harness the right tools to advance our knowledge of angiogenesis, ultimately driving forward both basic and translational research initiatives.
Principles of Tube Formation Assays
Understanding the principles behind tube formation assays is integral for grasping their significance in angiogenesis research. These assays simulate the conditions under which endothelial cells form tubular networks, mimicking the in vivo environment. This scientific approach unveils critical insights into blood vessel development, which has repercussions in various fields such as cancer treatment, wound healing, and cardiovascular disease. The key benefit of tube formation assays is their ability to provide a controlled environment for dissecting the mechanisms of angiogenesis at a cellular level.
Biological Mechanisms of Tube Formation
The biological mechanisms governing tube formation are inherently complex. Endothelial cells are key players in this process. When exposed to specific growth factors, such as vascular endothelial growth factor (VEGF), these cells undergo a series of coordinated responses which include proliferation, migration, and differentiation. They align and coalesce into tube-like structures, facilitating the formation of a new vascular network.
The involvement of the extracellular matrix, which provides structural support and biochemical signals, is also critical. It not only influences endothelial behavior but also interacts with various angiogenic factors, creating a fine-tuned environment conducive to tube formation.
In essence, grasping these biological mechanisms allows researchers to manipulate conditions effectively, tailoring experimental setups to meet specific research objectives.
Cell Types Involved
Endothelial Cells
Endothelial cells sit at the heart of tube formation assays due to their unique characteristic of forming blood vessels. They are a beneficial choice for these assays because they are responsive to angiogenic stimuli, a property that's paramount for eliciting the desired outcomes in experiments. One unique feature of endothelial cells is their ability to undergo morphological changes; they can transition from a quiescent state to an active form that facilitates tube formation in response to angiogenic signals.
However, this characteristic can also lead to disadvantages if not controlled properly, as overactivation may result in aberrant tube structures. Moreover, the necessity for appropriate culture conditions must always be kept in mind, as their behavior can be drastically different depending on the substrate or the presence of supporting cells.
Support Cells
Support cells, such as pericytes and fibroblasts, play a pivotal role in tube formation assays as well. They provide additional signals that help stabilize the nascent vascular structures. These cells are characterized by their ability to aid in the maturation and maintenance of blood vessels, enhancing the functionality of the formed tubes.
The inclusion of support cells in tube formation assays is regarded as a popular choice because they contribute to a more realistic model of in vivo angiogenesis. Their unique feature lies in their secretory profiles, which release growth factors and extracellular matrix components that actively influence endothelial cell behavior. On the flip side, the complexity they introduce can make data interpretation more challenging, necessitating careful experimental design.
"The integration of various cell types in tube formation assays can mimic the physiological conditions closely, drawing a fine line between experimental success and complexity."
In summary, the principles of tube formation assays extend beyond just the mechanics of endpoint outputs. They encompass a multifaceted understanding of the cellular actors involved, how those cells interact, and the environmental factors that influence their behavior. Each component plays a significant role in advancing our knowledge of angiogenesis and related diseases.
Experimental Design and Protocol
In the realm of biomedical research, a meticulously crafted experimental design is indispensable. When it comes to the angiogenesis tube formation assay, a well-thought-out protocol ensures not only the validity of the findings but also their reproducibility. This section will outline significant components that researchers need to consider when designing and executing their experiments.
The first element to assess is the choice of cell types and treatment conditions. Selecting the appropriate endothelial cellsāas these cells are pivotal in forming tubular structuresācan influence the outcome of the assay dramatically. For instance, using HUVECs (human umbilical vein endothelial cells) offers unique advantages due to their robust angiogenic capabilities. Furthermore, treatment with specific growth factors or inhibitors can provide insight into the dynamics of tube formation, yielding valuable data for analysis.
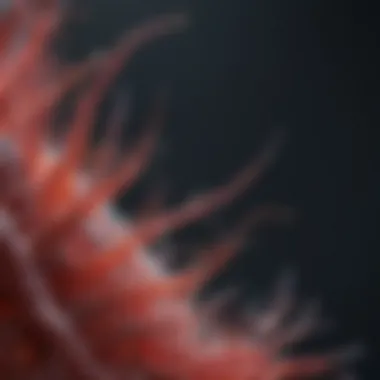
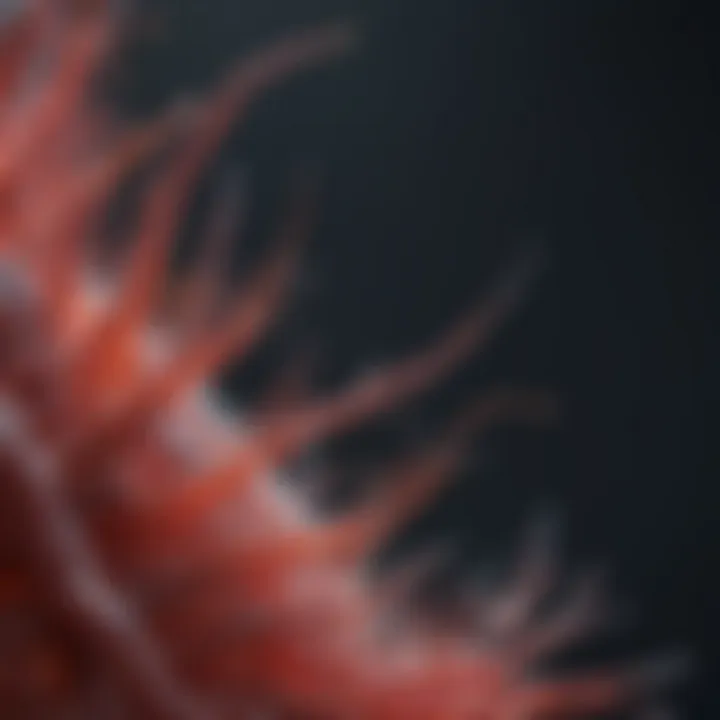
Another essential consideration involves the experimental controls. Itās vital to include negative controlsālike cells without any added stimuliāto understand the baseline behavior of the cells under investigation. Positive controls, on the other hand, should consist of known angiogenic stimuli, which assist in validating the assay's functionality.
Lastly, while preparing the assay, replicates play a crucial role in ensuring statistical relevance. Having multiple wells for each condition can help discern variability and confirm results are not merely coincidental phenomena. Thus, the design phase acts as the backbone of the angiogenesis tube formation assay, delineating clear pathways to achievement.
Preparation of Matrigel
Before diving into the nitty-gritty of the assay, the preparation of Matrigel warrants careful attention. Matrigel, a gelatinous protein mixture secreted by mouse sarcoma cells, simulates the extracellular matrix where blood vessel growth occurs. Proper handling of Matrigel is critical, as its properties can change based on temperature and time.
First and foremost, keep Matrigel chilled prior to use; it is recommended to store it at -20 °C and thaw it on ice. When ready to use, place the Matrigel on ice to maintain its liquid state, as it begins to solidify at 37 °C. Users often face the challenge of too thick or too thin layers, depending on their experimental needs. For tube formation, a layer about 200 µL per well in a 24-well plate is typically sufficient.
Matrigel should be distributed evenly in each well to ensure consistent conditions. Let it polymerize for about 30 minutes at 37 °C before introducing cells for the best outcome. This preparation phase is pivotal, forming the essential groundwork for successful tube formation.
Cell Culture Techniques
With the Matrigel prepped, the next step involves cell culture techniques. Growing endothelial cells under optimal conditions not only elevates their functionality but also prepares them for the rigors of the assay. The culture must provide a conducive environment, including the right nutrients and growth factors.
Begin by thawing the cells carefully to avoid shock. Use a gentle method, like slowly warming them in a water bath to achieve a 37 °C equilibrium. Once thawed, agitate the cells gently and culture them in a medium rich in growth factors, such as EGM-2, which provides factors needed for endothelial cell proliferation and tube formation.
Depending on the design of the experiment, cells may also need serum-free conditions for particular studies to minimize background signaling. As the cells grow, keep them under strict monitoring, rotating plates occasionally to ensure uniform exposure to necessary nutrients.
Further, ensuring that cells are at the correct density while seeding on Matrigel is vital. Ideal confluency is around 70%-80%, which optimally balances survivability and activity without causing overcrowding that could lead to erroneous results in the assay.
Assay Setup and Incubation
Now that cells are prepared correctly, itās time to delve into assay setup and incubation. To begin, each well containing polymerized Matrigel should be populated with the appropriate cell number. It is often suggested to use a density of about 1 x 10^5 cells per well for a 24-well plate, depending on the cell line and other experimental conditions.
After adding the cells, itās advantageous to introduce any experimental treatments at this stageālike growth factors or inhibitorsāto modulate their functions. Assays may also need to include controls at this point to ensure meaningful comparisons.
Following setup, incubate the plates at 37 °C in an incubator that provides a controlled humidity environment. Observe the cells at specific time intervals, commonly at around 4, 8, 12, and 24 hours, depending on the expected time frame for tube formation based on previous studies. As cells begin to form structures, consistency in observation is key, documenting changes that signal budding or elongation.
Good experimental practice emphasizes the need to capture visual data through photographs or time-lapse imaging. This approach assists in the quantitative analysis of the tube structure later on.
By the end of this phase, the groundwork will have been laid for data collection and analysis, closing the loop on the critical steps of the angiogenesis tube formation assay.
Data Analysis and Interpretation
Data analysis and interpretation play a pivotal role in the angiogenesis tube formation assay, serving as the cornerstone for dissecting the intricate dynamics of blood vessel development. After completing the experimental procedures, researchers are faced with the task of extracting meaningful conclusions from often complex data sets. This phase is essential, as a rigorous analysis can not only highlight the efficacy of angiogenic responses but also inform future studies and therapeutic developments.
Quantification of Tube Formation
Quantifying tube formation is more than just tallying up numbers. It involves a series of methods to accurately measure the length, density, and branching of the tubes formed during the assay. Each measurement can provide insights into how well an endothelial cell culture mimics in vivo angiogenesis.
Some of the common techniques employed include:
- Image Analysis: Using software like ImageJ, researchers can digitally analyze images of the tube networks formed. This software allows for precise measurements of total tube length and node counts.
- Manual Counting: Although more labor-intensive, manual counting can sometimes yield data that automated systems might miss, particularly in complex networks.
- Statistical Approaches: Various statistical tests, such as ANOVA, can help in validating the significance of observed changes across different experimental conditions.
Overall, quantification not only enhances the rigor of the assay but also elevates its reliability in conveying angiogenic potential.
Assessment of Angiogenic Factors
Understanding the factors that invigorate or inhibit angiogenesis is crucial for interpreting tube formation results. Two primary types of angiogenic factors commonly assessed are growth factors and matrix components.
Growth Factors
Growth factors are typically signaling proteins that stimulate cell proliferation and differentiation, and they play a vital role in the angiogenesis tube formation assay.
Some key growth factors include:
- Vascular Endothelial Growth Factor (VEGF): VEGF is often regarded as the primary growth factor in angiogenesis, being a key player in promoting the survival and proliferation of endothelial cells. Its high specificity for activating endothelial cells makes it a preferred choice in research settings.
- Fibroblast Growth Factor (FGF): FGF has been noted for its ability to enhance mitogenic activity across various cell types, not just endothelial cells. This broad functionality lends it great importance in angiogenic studies.
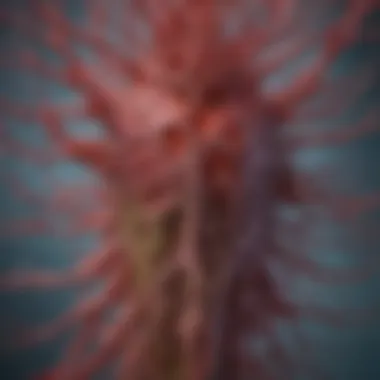
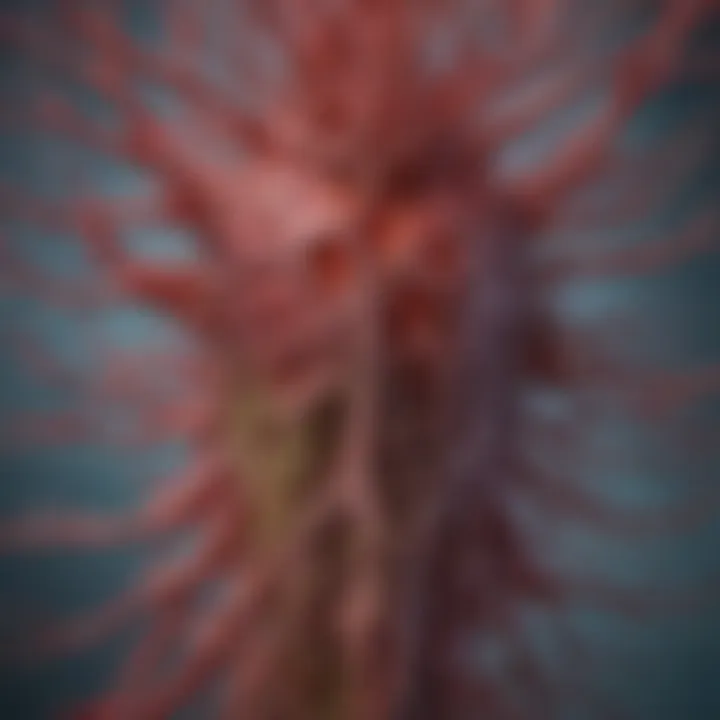
The unique feature of growth factors lies in their concentration dependence; a well-balanced interplay can lead to optimal tube formation. However, thereās a double-edged sword: excessive signaling can result in pathological angiogenesis, such as that seen in tumors.
Matrix Components
Matrix components refer to the extracellular matrix proteins that provide structural and biochemical support to surrounding cells. These components are critical to creating a conducive environment for angiogenesis.
Significant matrix components include:
- Fibronectin: Its ability to enhance cell adhesion and migration contributes to endothelial cell organization and tube morphogenesis, making it a staple in angiogenesis assays.
- Collagen: This protein not only acts as a scaffold but also plays a role in regulating cellular behavior during angiogenesis. Its adaptability to various forms, including types I, III, and IV, gives it remarkable utility in modeling.
The key characteristic of matrix components is their capacity to influence cellular behaviors through mechanical and biochemical cues. This enhances the overall relevance of the assay, although the challenge remains in mimicking the complex in vivo conditions.
The analysis of angiogenic factors holds the key to understanding the delicate balance between healthy and pathological angiogenesis.
Ultimately, the integration of data analysis and the assessment of angiogenic factors provides a holistic view of the angiogenesis tube formation assay, fostering an environment for groundbreaking research and actionable insights in the field.
Applications in Research
The angiogenesis tube formation assay serves as an indispensable tool in the field of biomedical research, particularly for studying angiogenesis mechanisms in diverse contexts. This assay not only sheds light on the formation of blood vessels but also helps elucidate various disease processes and therapeutic strategies. The significance of applying angiogenesis tube formation assays extends across several domains, making it a prominent choice for researchers aiming to understand both normal physiology and pathological conditions.
Cancer Research
In the realm of cancer research, the angiogenesis tube formation assay plays a pivotal role in unraveling the complexities of tumor growth and metastasis. Malignant tumors require a blood supply to nourish their rapid growth, making the evaluation of angiogenic processes essential. By monitoring how cancer cells influence endothelial cell behavior in these assays, researchers can pinpoint key angiogenic factors that drive aberrant vessel formation. This understanding can further facilitate the identification of potential therapeutic targets.
"Researching angiogenesis in cancer is akin to pulling at the threads of a vast tapestry; each thread reveals connections between tumor dynamics and vascular biology."
Moreover, the ability to assess the efficacy of anti-angiogenic drugsāmedications designed to inhibit blood vessel formationāmakes this assay invaluable. By comparing tube formation in treated versus untreated conditions, researchers obtain quantitative data on the effectiveness of these therapies, providing insights that can directly inform clinical applications.
Diabetes Studies
The implications of angiogenesis in diabetes research are profound, particularly in understanding complications such as retinopathy and peripheral vascular disease. The angiogenesis tube formation assay allows scientists to study the interplay between high glucose levels and endothelial cell function, highlighting how diabetes can disrupt normal vascular repair mechanisms.
Researchers often model various diabetic conditions to explore how altered metabolic states affect angiogenesis. This evaluation can lead to a better comprehension of how diabetic state influences wound healing and blood vessel regeneration. It brings clarity to therapeutic strategies aimed at improving vascular health among diabetic patients.
In addition, the assay can also assist in evaluating the potential benefits of new treatments aimed at restoring normal angiogenesis in diabetic individuals. Critical insights gleaned from these studies can guide clinical approaches to improve patient outcomes, targeting the underlying mechanisms that lead to diabetic complications.
Cardiovascular Research
Lastly, the implications of angiogenesis extend significantly into cardiovascular research. Abnormalities in blood vessel formation can lead to conditions such as atherosclerosis and myocardial infarction. The angiogenesis tube formation assay offers researchers a platform to examine endothelial dysfunction related to these conditions.
Through detailed analysis of tube formation under various conditions, researchers can identify outliers in angiogenic signaling pathways relevant to cardiovascular health. This information can help predict how different factors such as stress, inflammation, and lipid profiles might influence vessel stability and growth.
Furthermore, the assay can facilitate the investigation into regenerative medicine approaches for treating heart diseases. For example, exploring how stem cell therapies could promote healthy angiogenic responses in damaged cardiac tissues helps in crafting innovative treatment strategies.
Future Directions in Angiogenesis Research
Angiogenesis research stands at a pivotal junction in both basic science and its translational applications. With the ongoing evolution of technologies and an increasing understanding of disease mechanisms, the future of angiogenesis research offers strong potential for unraveling complexities of vascular biology. Each new discovery not only enhances our comprehension of physiological processes but also paves the way for innovative therapeutic strategies. Exploring future directions is an essential aspect of this article as it sheds light on emerging trends and possible applications that may significantly influence healthcare.
Technological Advancements
The landscape of angiogenesis research is being reshaped by a multitude of technological advancements. High-throughput screening technologies, for instance, now allow researchers to test numerous compounds in parallel, identifying potential angiogenic agents with unprecedented speed. This advancement is critical in drug discovery, as it can drastically reduce the time taken to bring new therapies to clinical trials.
Additionally, advances in imaging techniques, such as in vivo imaging and three-dimensional imaging, enable scientists to observe angiogenesis in real time. These tools provide insights into how blood vessels form under different physiological and pathological conditions. Such imaging capabilities have the potential to enhance our understanding of tumor microenvironments and the intricate interplay between cancer cells and angiogenic processes.
Moreover, the integration of bioinformatics and computational modeling provides a powerful platform for simulating angiogenesis. By leveraging big data analysis, researchers can analyze vast arrays of data to predict the angiogenic potential of novel compounds or to examine the effects of genetic modifications on vascular development. These technological leaps foster a comprehensive understanding of the mechanisms governing angiogenesis, which could lead to new therapeutic avenues.
Potential Therapeutic Approaches
The insights gained from angiogenesis research are forming the backbone of potential therapeutic approaches, especially in the realms of cancer treatment, wound healing, and cardiovascular diseases. One promising avenue is the development of anti-angiogenic therapies, which aim to inhibit the growth of blood vessels in tumors, starving cancer cells of the nutrients they require to proliferate. While several anti-angiogenic drugs have been approved for clinical use, ongoing research seeks to refine these therapies, improve their efficacy, and reduce side effects.
On the flip side, promoting angiogenesis can also have therapeutic benefits. For instance, in cases of ischemic diseases, where blood flow to tissues is compromised, stimulating angiogenesis could help restore blood supply and facilitate healing. Experimental therapies that encourage the formation of new blood vessels could significantly improve outcomes for patients suffering from conditions such as peripheral artery disease or cerebral ischemia.
Additionally, understanding the role of stem cells in angiogenesis opens yet another layer of potential treatments. Stem cells can differentiate into endothelial cells, responsible for forming blood vessels. Harnessing this potential could lead to regenerative therapies that repair damaged tissues through controlled vessel formation.
"Unlocking the future of angiogenesis will rely not just on understanding it better, but on how that understanding can be translated into real-world applications for treating human ailments."