3D Hydrogel Cell Culture: Advancements and Applications
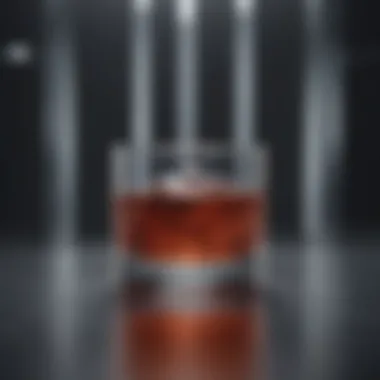
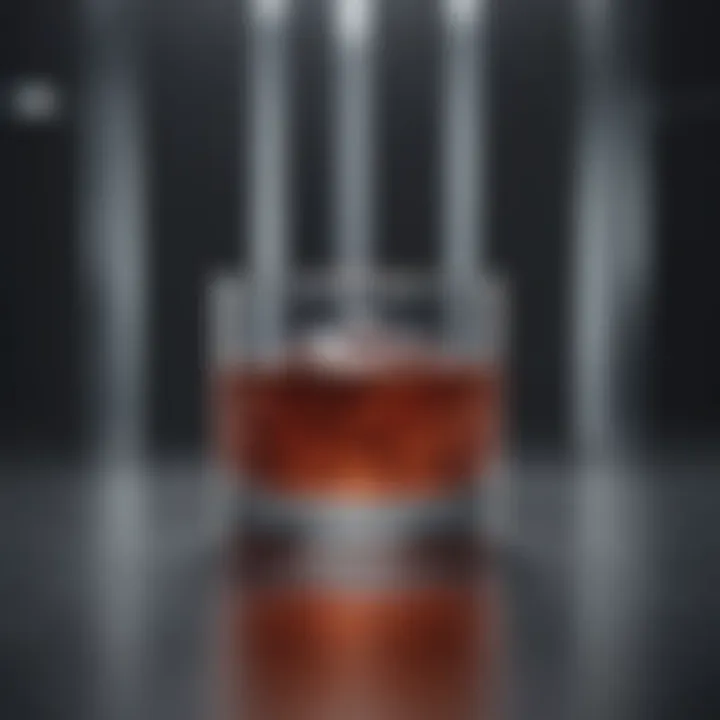
Intro
The field of biological research and tissue engineering has seen significant enhancements due to the advent of three-dimensional (3D) hydrogel cell culture techniques. This novel approach offers a more physiologically relevant environment compared to traditional two-dimensional cultures, which can be limited in mimicking the complexity of human tissues. The integration of hydrogels into cell culture has opened new avenues for studying cellular behavior, drug responses, and regenerative processes. It is vital for students, researchers, and professionals to understand the intricacies of this technology and its impact on various scientific disciplines.
3D hydrogels are biocompatible polymers that can retain a large volume of water, providing a matrix that supports cellular growth and differentiation. The ability to tailor their biochemical properties allows for specific applications in regenerative medicine, cancer research, and drug development. As the research landscape evolves, investigating the mechanisms of cell signaling and interaction becomes ever more critical, and hydrogels provide the ideal platform for such explorations.
In this article, the discussion will focus on the methodologies, advantages, and current challenges in implementing 3D hydrogel cell culture technologies. The analysis aims to provide a comprehensive overview that not only highlights the advancements but also reflects on the future implications for medicine and bioengineering.
Methodologies
Description of Research Techniques
At the core of 3D hydrogel cell culture lies a variety of research techniques that enable the generation of complex tissue models. Techniques such as 3D bioprinting, electrospinning, and photopolymerization are commonly employed to fabricate hydrogel scaffolds that can support various cell types.
- 3D Bioprinting: This advanced technique allows for precise placement of living cells within a hydrogel matrix. Multiple energy sources, such as lasers or inkjet printers, can be utilized to create layered structures that mimic native tissues.
- Electrospinning: This method involves the application of a high-voltage electric field to produce nano-fibrous scaffolds from polymer solutions, which can then be infused with hydrogels to enhance cellular attachment and growth.
- Photopolymerization: This process uses light to trigger the polymerization of a hydrogel in situ, allowing researchers to control the gelation process in real-time, facilitating the encapsulation of cells.
Tools and Technologies Used
The successful implementation of 3D hydrogel cell culture relies on various tools and technologies that support both research and application. Some essential equipment includes:
- Bioreactors: Specialized systems that provide a controlled environment for cell growth, supplying optimal nutrient delivery and waste removal. Popular brands include Eppendorf cell culture flasks and Sartorius bioreactors.
- Microscopy Technologies: Advanced imaging tools such as confocal and fluorescence microscopy enable researchers to visualize cell behavior and interactions within hydrogels.
- Characterization Tools: Techniques like rheology and scanning electron microscopy are crucial for evaluating the physical properties and structure of hydrogels. This is essential for understanding how these properties influence cellular response.
Discussion
Comparison with Previous Research
A comparative analysis with prior studies indicates that the shift from 2D to 3D cell culture systems has led to more accurate representations of in vivo environments. Previous research primarily focused on monolayer cultures, which do not accommodate the complexity of cellular interactions. In contrast, 3D cultures allow for enhanced cellular signaling pathways and physiological processes.
This transition has enhanced our understanding of various disease models, particularly in cancer research. Notably, studies have shown that cell behavior changes significantly when cultured in 3D environments, leading to improved drug response predictions. For instance, a study by Tuxworth et al. highlighted that hydrogels enable investigations into tumor microenvironments, which are pivotal for determining therapeutic approaches.
Theoretical Implications
The theoretical implications of 3D hydrogel technology extend far beyond current applications. As the understanding of cell behavior in hydrogels improves, this knowledge may pave the way for new therapeutic strategies in regenerative medicine. Improved models can facilitate drug screening processes, ultimately accelerating the timeline from preclinical studies to clinical trials. This has profound implications, particularly concerning patient-specific therapies and personalized medicine.
"The evolution of hydrogel technology is reshaping our comprehension of cellular dynamics, offering unprecedented insights into drug development and tissue engineering."
– Recent Research Publications, 2023.
Preface to 3D Hydrogel Cell Culture
3D hydrogel cell culture represents a pivotal innovation in the field of biological research and tissue engineering. This technique allows for the more accurate modeling of human tissues and organs, providing crucial insights that traditional two-dimensional cultures cannot offer. By creating a more biomimetic environment for cells, researchers can better understand complex cellular interactions and behaviors, which are necessary for advancements in regenerative medicine and drug development. The ability to mimic the extracellular matrix in 3D hydrogels enables scientists to explore physiological responses in a more relevant context, thus enhancing the quality and reliability of their findings.
The implementation of 3D hydrogel technology offers several benefits. First, it facilitates cell-cell and cell-matrix interactions, which are critical for maintaining cell viability and function. Many types of cells grow differently when placed in a 3D structure compared to flat surfaces. Second, 3D hydrogel cell cultures can be designed to release growth factors and other signaling molecules, further enhancing the ability of cells to thrive and communicate. Third, this technology promotes more uniform nutrient and waste exchange, resulting in healthier and more representative cell growth.
In this article, the importance of understanding 3D hydrogel cell culture will be elaborated through various sections. These include fundamental principles of hydrogel technology, types of hydrogels utilized, and their applications in various fields such as regenerative medicine and cancer research. By exploring the historical context alongside the advancements made in hydrogel formulations, the reader will appreciate the transformative potential of this technology in scientific research and clinical applications.
Definition and Overview
3D hydrogel cell culture involves the use of hydrogels—a network of polymer chains that can retain significant amounts of water—to support cell growth in a three-dimensional environment. Hydrogels can be composed of natural or synthetic materials and can be tailored to mimic specific physical and biochemical properties of the human extracellular matrix. This setup allows cells to grow, migrate, and differentiate in ways that are closer to their natural settings, as opposed to two-dimensional cultures that are limited and often lead to altered cell behaviors.
Hydrogels provide hydration and a suitable architecture for cells. Because these gels can also be bioactive, adding growth factors or specific molecules can further enhance cellular performance. This aspect of customizability makes hydrogels an attractive choice for a wide range of applications in science and medicine.
Historical Context
The use of hydrogels in cell culture is not a recent development. Its origins can be traced back several decades when researchers began seeking improved methods to culture cells more effectively. Early formulations used agarose and collagen which provided a basic environment for various cell types. However, limitations in material properties and lack of control over structural integrity hindered progress.
In the late 20th century, advancements in polymer chemistry led to the creation of synthetic hydrogels with improved tunability and stability. These developments spurred increased interest in 3D cell culture, particularly as researchers recognized that traditional techniques fell short when it came to replicating complex in vivo environments. Since then, considerable research has focused on optimizing hydrogel composition and structure to cater to specific cell types and applications. As a result, the current landscape of 3D hydrogel technology reflects a rich tapestry of innovation and increased understanding of cell behavior.
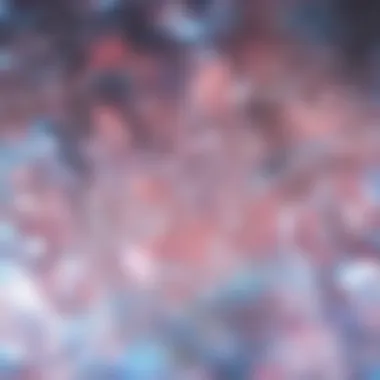
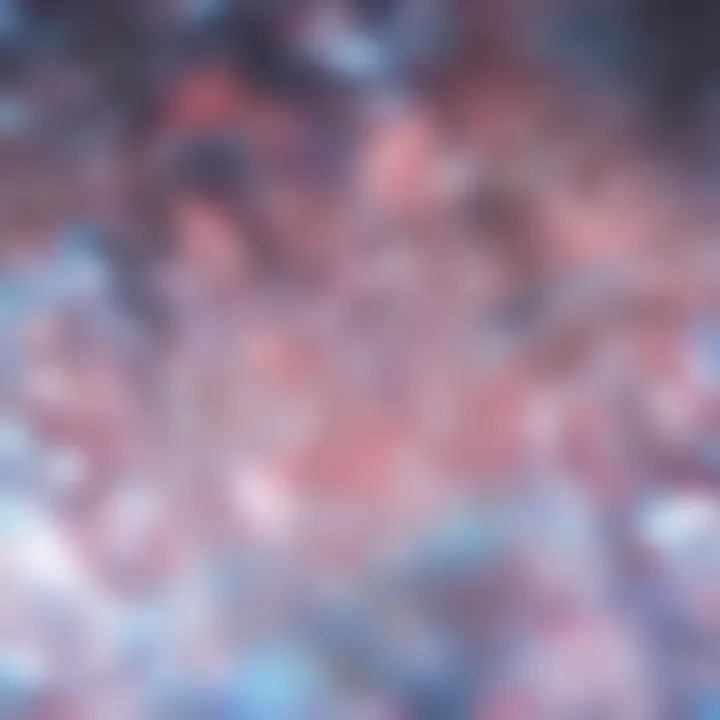
Fundamental Principles of Hydrogel Technology
Understanding the fundamental principles of hydrogel technology is crucial for comprehending its applications in 3D cell culture. Hydrogels are characterized by their unique ability to retain a significant amount of water while maintaining a three-dimensional structure. This property allows them to closely mimic the natural extracellular matrix, which is essential for supporting cellular functions.
Hydrogels are versatile materials composed primarily of hydrophilic polymer networks that can swell in water. The interaction of these materials with biological systems has significant implications for areas such as drug delivery, tissue engineering, and regenerative medicine.
Material Composition
The material composition of hydrogels is vital as it directly influences their functionality. Natural hydrogels, such as alginate, collagen, and gelatin, are derived from biological sources and exhibit biocompatibility. Their composition often includes polysaccharides or proteins, which contribute to their ability to support cell attachment and growth.
In contrast, synthetic hydrogels like poly(ethylene glycol) or polyvinyl alcohol offer tunable properties. These materials can be engineered to achieve desired mechanical and degradation characteristics, allowing for optimization in various applications. Understanding the distinctions in composition is important for selecting the right hydrogel for specific research needs.
Physical Properties
Physical properties of hydrogels include their mechanical strength, porosity, and swelling behavior. These properties determine how well a hydrogel can provide structural support to encapsulated cells. A physically robust hydrogel can withstand the stresses of cellular activities and external forces.
Porosity is another critical factor. Hydrogels that allow nutrient and waste diffusion are crucial for maintaining cellular health. They must also possess appropriate swelling capacities to avoid excessive rigidity or laxity in their environment. Balancing these physical properties is essential in developing effective culture systems.
Biochemical Properties
The biochemical properties of hydrogels play a pivotal role in cell interaction. These properties include the presence of bioactive molecules, such as growth factors or adhesion peptides. These molecules can significantly influence cell behavior, promoting proliferation or differentiation.
Moreover, the interaction between cells and the hydrogel matrix can alter gene expression and protein synthesis in the cells, impacting their overall function. Thus, tailoring biochemical properties enhances the utility of hydrogels in research and therapeutic applications.
"Hydrogels, with their unique properties, not only serve as 3D scaffolds but also facilitate complex cellular interactions that are crucial for advancing biomedical research."
In summary, the fundamental principles of hydrogel technology encompass material composition, physical properties, and biochemical properties. A thorough understanding of these aspects is essential to harness the full potential of 3D hydrogel cell culture in various fields of research and therapy.
Types of Hydrogels Used in Cell Culture
3D hydrogel cell culture relies heavily on the types of hydrogels selected for experiments. Different hydrogels bring unique characteristics that can enhance cell growth, differentiation, and functionality. The choice of hydrogel affects not only the behavior of the cells but also the outcomes of experiments. Understanding the distinctions, benefits, and considerations associated with various types of hydrogels is essential for researchers aiming to optimize their cell culture models.
Natural Hydrogels
Natural hydrogels are bio-derived substances that closely mimic the cellular environment. Common examples of natural hydrogels include collagen, hyaluronic acid, gelatin, and alginate. One of the key advantages of using these hydrogels is their biocompatibility, promoting cell adhesion and proliferation. Because these materials are derived from biological sources, they often provide biochemical cues that can facilitate cell function.
- Biochemical activity: Natural hydrogels typically retain bioactive motifs found in the extracellular matrix, which can influence cellular behavior. This supports applications in tissue engineering where cell native behavior is paramount.
- Degradation rates: Natural hydrogels can be designed to degrade at specific rates, enabling controlled release of growth factors or nutrients, which can be critical in regenerative medicine.
However, there are challenges too. These materials often lack mechanical strength and can exhibit batch-to-batch variability. This variability can introduce inconsistencies into experiments, complicating the interpretation of results.
Synthetic Hydrogels
On the other hand, synthetic hydrogels are created through chemical processes. These materials allow for precise control over the mechanical and physical properties. Common synthetic hydrogels include polyethylene glycol (PEG), polyacrylamide, and polyvinyl alcohol (PVA). The advantages of synthetic hydrogels become especially apparent in advanced applications.
- Tailored properties: Researchers can customize synthetic hydrogels based on specific needs, adjusting parameters like stiffness, porosity, and degradation rates. This flexibility can lead to improved experimental design and outcomes.
- Reproducibility: The production process of synthetic hydrogels often allows for consistent material properties, which can enhance replicability in scientific experiments.
Nevertheless, synthetic materials may not always possess the same biochemical signals as natural alternatives, possibly neglecting critical cellular interactions. The lack of innate bioactivity can be a limitation when trying to recreate complex tissue environments.
In summary, both natural and synthetic hydrogels play vital roles in 3D hydrogel cell culture. Their unique properties and challenges should be considered carefully to harness their benefits in cellular research and therapy. The enhanced understanding of these materials leads the way for significant advancements in biomedicine.
Applications of 3D Hydrogel Cell Culture
The realm of 3D hydrogel cell culture is crucial in advancing biological research and medical applications. This section highlights four pivotal domains where 3D hydrogels have significantly transformed methodologies and outcomes. The unique properties of hydrogels, such as their ability to mimic the natural extracellular matrix, enhance cell behavior study and therapeutic innovations. We will explore regenerative medicine, cancer research, drug development, and tissue engineering within this context.
Regenerative Medicine
Regenerative medicine aims to restore the function of damaged tissues and organs. Here, 3D hydrogel cell cultures play a vital role. These hydrogels support cell viability and function, providing a supportive framework for cell growth. With their tunable mechanical properties, researchers can design hydrogels that closely resemble the native tissue environment. This reduces the chances of inflammatory reactions when implanted into the body. Moreover, hydrogels facilitate the delivery of bioactive molecules that promote healing. By simulating various tissue architectures, they enhance the study of cell interactions, paving the way for innovative treatment strategies in conditions like cardiac and neural repair.
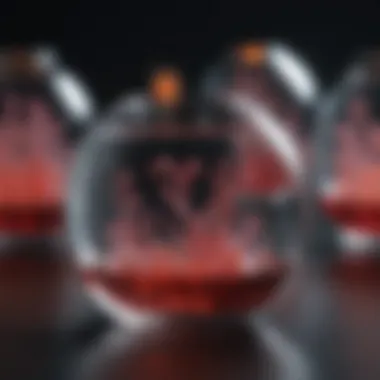
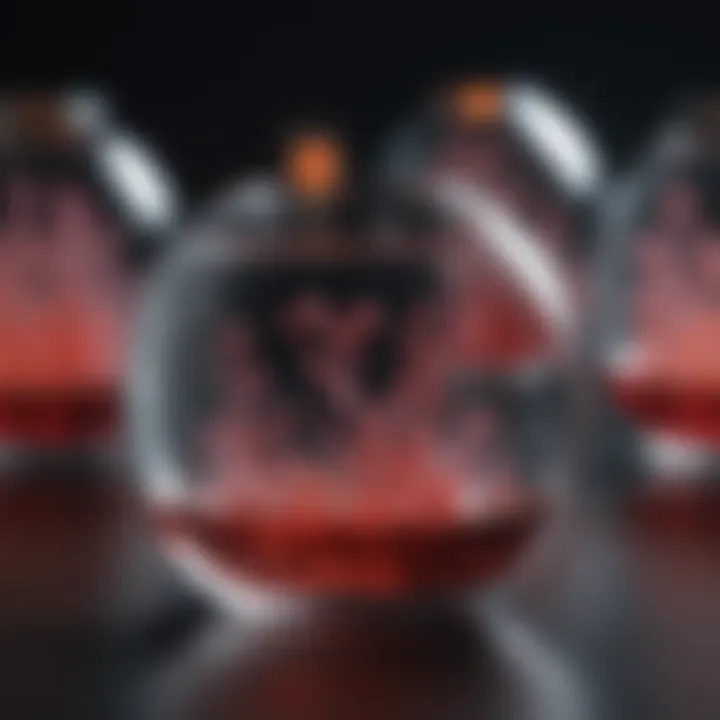
Cancer Research
Cancer remains a leading global health challenge, with research continually seeking better models for tumor study. 3D hydrogel systems present a superior alternative to traditional 2D cultures by enabling a more realistic tumor microenvironment. Cells within 3D hydrogels show enhanced proliferation and gene expression that mimic in vivo situations. This capability allows researchers to observe cancer cell growth patterns, invasion, and response to therapies in more detail. For instance, studies involving breast cancer cells in hydrogel scaffolds have revealed new insights into metastatic behavior. Such findings can lead to more effective treatments tailored to individual tumor profiles.
Drug Development
Drug development is a notoriously lengthy and costly process. The integration of 3D hydrogel cell culture provides a versatile platform for drug screening and toxicity assessment. These systems allow for high-throughput assays that more accurately reflect drug responses. By utilizing patient-derived cells in 3D cultures, researchers can assess pharmacokinetics and efficacy under conditions that closely resemble the human body. Studies have shown that drugs often display different attributes in 3D versus 2D environments. This insight can lead to more reliable predictions about clinical outcomes. Furthermore, hydrogels are useful in studying drug delivery mechanisms, enhancing the development of targeted therapies that reduce side effects.
Tissue Engineering
Tissue engineering seeks to create functional biological substitutes for damaged tissues. 3D hydrogel platforms are integral to this field, as they provide a scaffold that dictates cell morphology and behavior. Depending on their composition, hydrogels can be engineered to support various cell types, facilitating the production of specific tissues like cartilage or bone. Additionally, they can be designed to release growth factors that encourage tissue regeneration. The combination of 3D printing and hydrogel technology further broadens the potential for creating complex tissue structures with spatially controlled architecture. This innovation can ultimately aid in developing organoids that mimic human organ functions for research and transplantation.
Mechanisms of Cell Behavior in 3D Cultures
The study of mechanisms governing cell behavior in three-dimensional (3D) hydrogel cultures is vital for advancing both biological research and practical applications. Traditional two-dimensional (2D) cultures fail to mimic the complex microenvironments found in vivo. Consequently, understanding how cells interact with hydrogels in a 3D context provides crucial insights that can inform better practices in regenerative medicine, cancer research, and drug development.
Cell Migration and Proliferation
Cell migration and proliferation are essential aspects of tissue development and regeneration. In 3D hydrogel environments, cells demonstrate more natural migratory behavior compared to 2D cultures. This can be attributed to the supportive structure provided by the hydrogel, which allows cells to better encapsulate and orient themselves.
- Factors Influencing Migration
The migration of cells within a 3D hydrogel is influenced by matrix composition, stiffness, and porosity. These factors dictate how cells can move and respond to biochemical signals. - Proliferation Mechanism
Cell proliferation in 3D cultures has been linked to enhanced cell-cell and cell-matrix interactions. This results in better survival rates and increased metabolic activity. In contrast, 2D cultures often lead to contact inhibition, limiting growth.
Understanding the dynamics of cell migration and proliferation in 3D hydrogels is crucial. This knowledge allows researchers to design better scaffolds that encourage optimal cell functionality during various experimental procedures.
Cell Differentiation
The process of cell differentiation is another critical area where 3D hydrogel cell cultures show significant advantages. In a suitable 3D environment, stem and progenitor cells exhibit enhanced differentiation capabilities into desired cell types. This is essential for tissue engineering and regenerative medicine applications.
- Influence of Hydrogel Properties
The biochemical and physical properties of the hydrogel directly affect differentiation. For example, hydrogels that mimic the extracellular matrix can provide cues necessary for specific differentiation pathways. - Signaling Pathways
Factors such as growth factors and matrix stiffness can activate key signaling pathways that drive differentiation. 3D hydrogels allow researchers to control these variables more effectively than conventional culture methods, promoting target cell lineage.
"Understanding the mechanisms behind cell behavior in 3D cultures is not just an academic exercise; it's essential for developing effective tissue engineering applications and regenerative therapies."
By investigating the mechanisms of cell behavior in 3D cultures, researchers can better tailor hydrogel properties for specific applications. This enhances the efficacy of research endeavors and improves outcomes in clinical scenarios.
Challenges in 3D Hydrogel Cell Culture
The exploration of 3D hydrogel cell culture is essential not just for its applications but also for the significant challenges it presents. Understanding these challenges is crucial to enhance the effectiveness and reliability of hydrogel technologies in various environments. This section examines scalability and reproducibility, two key issues that can impact research outcomes and clinical applications.
Scalability Issues
Scalability represents a persistent challenge within the 3D hydrogel cell culture arena. As researchers aim to expand hydrogel use beyond laboratory environments to larger, commercial scales, various factors must be considered. The intricacies involved in scaling up production without compromising quality can be daunting.
As culture systems become larger, achieving uniformity in hydrogel properties becomes more difficult. Variability in cross-linking density or composition can lead to inconsistent cellular responses. This creates a barrier for utilizing 3D hydrogels in reliable clinical settings, as variations can lead to unpredictable results which can affect therapeutic effectiveness.
Additionally, cost-effectiveness becomes a major concern when attempting to scale production. The production methods used on a small scale may not be economically viable for mass production. Finding a balance between optimizing the material properties and maintaining low costs is an ongoing difficulty. Achieving a scalable manufacturing process while keeping in mind the biochemical properties unique to each type of hydrogel requires substantial innovation and research.
Reproducibility Concerns
Reproducibility is another significant concern in 3D hydrogel cell culture. For a scientific field to advance, researchers must be able to replicate findings consistently. However, with current hydrogel technologies, differences in procedures, including variations in material selection and hydrogel preparation, can lead to distinct outcomes.
Such inconsistencies arise from differing experimental conditions and environmental factors. Parameters such as temperature, pH, and ionic strength can fluctuate, affecting the hydrogel's behavior and, subsequently, cell interaction. As a result, achieving reliable experimental outcomes can be challenging.
Moreover, reproducibility issues can hinder collaboration across research institutions. When one lab’s results cannot be reproduced by another, it limits the sharing of knowledge and slows down overall scientific progress. This necessitates stricter standardization in the protocols for hydrogel fabrication and cell culture methodologies.
In summary, while 3D hydrogel cell culture offers promising applications in various fields, scalability and reproducibility remain significant hurdles. Addressing these challenges is vital for advancing the use of these hydrogels in practical applications, especially in medicine and biotechnology.
"Consistent results are the cornerstone of scientific research; without them, our interpretations can easily lead to misleading conclusions."
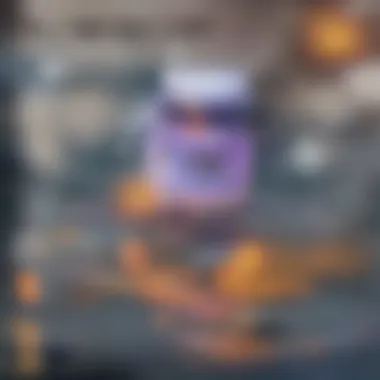
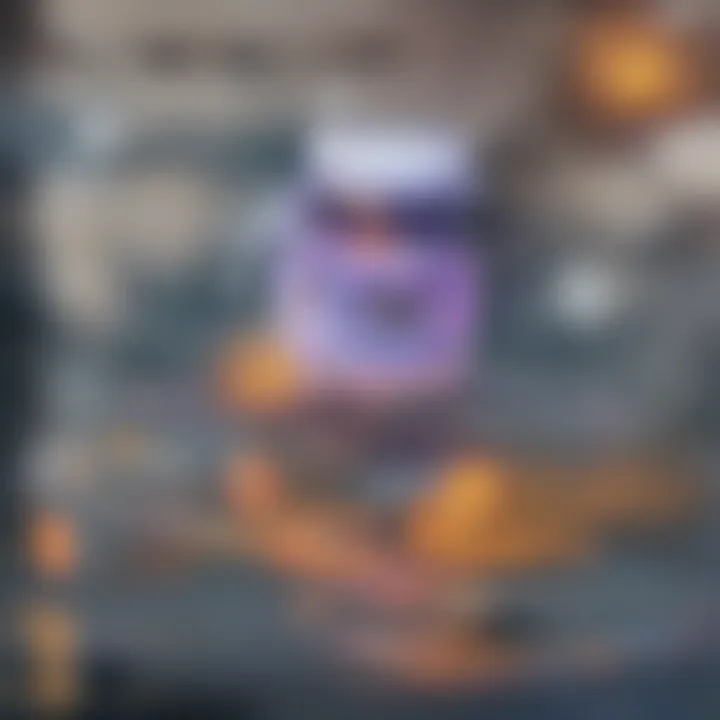
Future innovations must focus on these areas to enhance the reliability and application of 3D hydrogels in cellular studies.
Future Directions in Hydrogel Technology
The future of hydrogel technology in cell culture presents a fascinating landscape of possibilities and innovations. As researchers explore the potential of 3D hydrogel systems, many new directions are emerging, poised to enhance our understanding and capabilities in biological research. This section will focus on the specific advancements being made in hydrogel formulations and their integration with bioprinting techniques.
Innovations in Hydrogel Formulations
Innovative hydrogel formulations are crucial for advancing 3D cell culture methodologies. Researchers aim to develop materials that more closely mimic the natural extracellular matrix, providing a conducive environment for cell growth and differentiation. These include:
- Smart Hydrogels: These responsive materials can change their properties in response to environmental stimuli such as pH, light, or temperature. This adaptability allows for dynamic control of cell behavior and facilitates more complex biological studies.
- Composite Hydrogels: By combining different types of hydrogels, scientists can create composites that incorporate beneficial properties from multiple sources. This can enhance mechanical strength or introduce specific biochemical functionalities.
- Bioactive Hydrogels: These formulations include factors like growth factors and proteins that can directly affect cellular activities. This enables more precise engineering of cell interactions, which is beneficial for applications in regenerative medicine.
Innovations like these are not only expanding the utility of hydrogels in research but also paving the way for real-world applications in therapies and drug delivery systems.
Integration with Bioprinting Techniques
The integration of hydrogel technology with bioprinting techniques marks a significant stride in tissue engineering. Bioprinting allows for precise layer-by-layer deposition of cells and hydrogels, enabling the creation of complex tissue structures that closely resemble natural tissues. Benefits of this integration include:
- Customizable Structures: Researchers can design tissue scaffolds with specific architectures tailored to particular applications, allowing for more meaningful studies and potential therapies.
- Enhanced Cell Viability: Bioprinting can help maintain cell viability by controlling the microenvironment during the printing process. This ensures that cells are not exposed to harmful conditions that could compromise their functions.
- Scalability: The ability to easily replicate and scale bioprinted hydrogels supports large-scale production for clinical applications. This is important for the commercialization of tissue-engineered products.
"As the boundaries between bioprinting and hydrogel technologies blur, new opportunities arise for the creation of living systems with the potential to transform medical treatments."
Regulatory Aspects of Hydrogel Use
The exploration of 3D hydrogel cell culture technologies extends beyond scientific innovation; it necessitates rigorous regulatory frameworks. Regulation plays a crucial role in ensuring the safety and efficacy of these materials used in both research and clinical applications. Given the dynamic nature of hydrogel composition and their diverse uses in regenerative medicine and tissue engineering, oversight is essential for the protection of public health.
Hydrogels used in cell culture must be thoroughly evaluated to meet specific safety standards. This involves assessing the biocompatibility of materials, as well as their degradation products, which could pose risks to cellular function. Regulatory agencies aim to mitigate risks associated with the introduction of foreign materials into biological systems. As the applications of these hydrogels diversify, updated guidelines become imperative to encompass all potential uses.
Safety Considerations
The safety of hydrogels is paramount. Several aspects must be considered:
- Material Selection: The choice of polymer significantly affects biocompatibility. Naturally derived materials like alginate or synthetic options like polyethylene glycol (PEG) require different assessments.
- Cellular Responses: It’s important to evaluate how different cell types react when cultured in these hydrogels. Cytotoxicity tests can reveal harmful effects and prevent harmful cell behavior.
- Contamination Risks: Hydrogels, if not manufactured under sterile conditions, can harbor pathogens that threaten both research integrity and patient health.
A regulatory approach emphasizing thorough testing leads to a higher standard of safety for products entering the market.
Ethics in Cell Culture Research
Alongside safety, ethical considerations in hydrogel use are critical. Ethical integrity ensures that scientific advancements don't compromise moral or social values. Here are key factors:
- Animal Welfare: When developing and testing hydrogels, researchers must consider the impact on animal models, as using animals for testing should minimize suffering.
- Informed Consent: In clinical settings, patient awareness and consent relating to hydrogel-based therapies are essential. Transparency about potential risks fosters trust.
- Data Management: Ethical standards in data management must be upheld to avoid misinterpretations that can arise from biased data, particularly in clinical trials involving hydrogels.
"Regulation not only guarantees safety but also nurtures ethical standards in advancing hydrogel technology."
Closure
The significance of 3D hydrogel cell culture in biological research and tissue engineering cannot be overstated. This article has explored the foundational principles of hydrogel technology while examining its various applications, such as in regenerative medicine and cancer research. The intricate details presented in previous sections have provided insights into not only the material properties but also the complex behaviors of cells when placed in a three-dimensional matrix.
At the core, the advantages of employing hydrogels in cell culture are significant. They allow for a more physiological environment, which in turn can enhance cell viability, migration, and differentiation. Furthermore, the adaptability of hydrogels in terms of formulation and mechanical properties means they can be tailored to meet specific research needs, unlike traditional two-dimensional cultures.
However, it is important to acknowledge that while these advancements signify progress, they also bring forth challenges, particularly in scalability and reproducibility. Such challenges necessitate continuous investigation into innovative solutions—this will ultimately fortify the foundation upon which future research will be conducted.
Summary of Key Points
- 3D hydrogel cell culture enhances physiological relevance over 2D culture systems, providing a more accurate biological environment for cells.
- The adaptability of hydrogels allows specific tailoring to various research applications, promoting advancements in tissue engineering and regenerative medicine.
- Current challenges include issues related to scalability and reproducibility, which must be addressed as research progresses.
Calls for Further Research
Further studies are essential in several areas:
- Material Innovation: New formulations of hydrogels could improve functionality and biocompatibility. Research should explore diverse natural and synthetic materials that could better mimic the extracellular matrix.
- Integration Techniques: The conjunction of bioprinting with hydrogel technologies may enhance cellular arrangement and viability, warranting a deep dive into how these methods can work in concert.
- Longitudinal Studies: Research should focus on the long-term behavior of tissues engineered in hydrogels to assess their suitability for eventual clinical applications.
As the field of 3D hydrogel cell culture continues to evolve, the potential applications and implications of this technology remain vast. Ongoing research and combined efforts from academics and industry will significantly advance the development of effective therapies that tackle various diseases.